- Scoping Review
- Open access
- Published: 14 November 2021
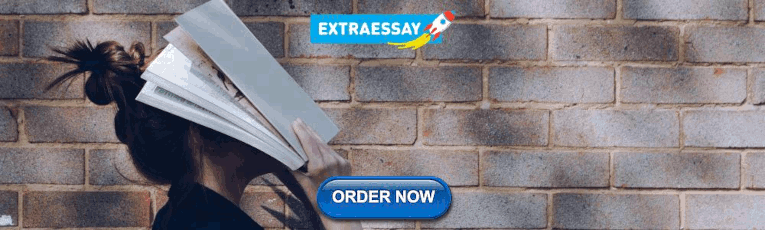
Effectiveness and safety of SARS-CoV-2 vaccine in real-world studies: a systematic review and meta-analysis
- Qiao Liu 1 na1 ,
- Chenyuan Qin 1 , 2 na1 ,
- Min Liu 1 &
- Jue Liu ORCID: orcid.org/0000-0002-1938-9365 1 , 2
Infectious Diseases of Poverty volume 10 , Article number: 132 ( 2021 ) Cite this article
56k Accesses
204 Citations
372 Altmetric
Metrics details
To date, coronavirus disease 2019 (COVID-19) becomes increasingly fierce due to the emergence of variants. Rapid herd immunity through vaccination is needed to block the mutation and prevent the emergence of variants that can completely escape the immune surveillance. We aimed to systematically evaluate the effectiveness and safety of COVID-19 vaccines in the real world and to establish a reliable evidence-based basis for the actual protective effect of the COVID-19 vaccines, especially in the ensuing waves of infections dominated by variants.
We searched PubMed, Embase and Web of Science from inception to July 22, 2021. Observational studies that examined the effectiveness and safety of SARS-CoV-2 vaccines among people vaccinated were included. Random-effects or fixed-effects models were used to estimate the pooled vaccine effectiveness (VE) and incidence rate of adverse events after vaccination, and their 95% confidence intervals ( CI ).
A total of 58 studies (32 studies for vaccine effectiveness and 26 studies for vaccine safety) were included. A single dose of vaccines was 41% (95% CI : 28–54%) effective at preventing SARS-CoV-2 infections, 52% (31–73%) for symptomatic COVID-19, 66% (50–81%) for hospitalization, 45% (42–49%) for Intensive Care Unit (ICU) admissions, and 53% (15–91%) for COVID-19-related death; and two doses were 85% (81–89%) effective at preventing SARS-CoV-2 infections, 97% (97–98%) for symptomatic COVID-19, 93% (89–96%) for hospitalization, 96% (93–98%) for ICU admissions, and 95% (92–98%) effective for COVID-19-related death, respectively. The pooled VE was 85% (80–91%) for the prevention of Alpha variant of SARS-CoV-2 infections, 75% (71–79%) for the Beta variant, 54% (35–74%) for the Gamma variant, and 74% (62–85%) for the Delta variant. The overall pooled incidence rate was 1.5% (1.4–1.6%) for adverse events, 0.4 (0.2–0.5) per 10 000 for severe adverse events, and 0.1 (0.1–0.2) per 10 000 for death after vaccination.
Conclusions
SARS-CoV-2 vaccines have reassuring safety and could effectively reduce the death, severe cases, symptomatic cases, and infections resulting from SARS-CoV-2 across the world. In the context of global pandemic and the continuous emergence of SARS-CoV-2 variants, accelerating vaccination and improving vaccination coverage is still the most important and urgent matter, and it is also the final means to end the pandemic.
Graphical Abstract
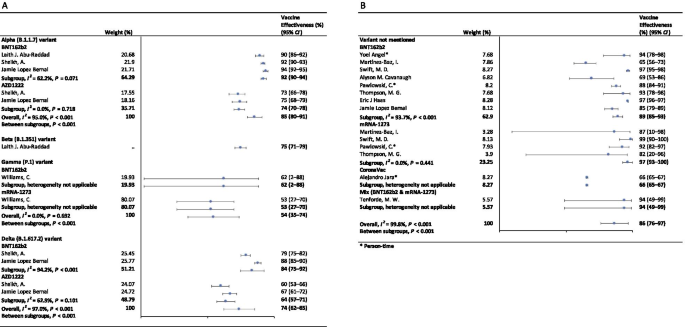
Since its outbreak, coronavirus disease 2019 (COVID-19) has spread rapidly, with a sharp rise in the accumulative number of infections worldwide. As of August 8, 2021, COVID-19 has already killed more than 4.2 million people and more than 203 million people were infected [ 1 ]. Given its alarming-spreading speed and the high cost of completely relying on non-pharmaceutical measures, we urgently need safe and effective vaccines to cover susceptible populations and restore people’s lives into the original [ 2 ].
According to global statistics, as of August 2, 2021, there are 326 candidate vaccines, 103 of which are in clinical trials, and 19 vaccines have been put into normal use, including 8 inactivated vaccines and 5 protein subunit vaccines, 2 RNA vaccines, as well as 4 non-replicating viral vector vaccines [ 3 ]. Our World in Data simultaneously reported that 27.3% of the world population has received at least one dose of a COVID-19 vaccine, and 13.8% is fully vaccinated [ 4 ].
To date, COVID-19 become increasingly fierce due to the emergence of variants [ 5 , 6 , 7 ]. Rapid herd immunity through vaccination is needed to block the mutation and prevent the emergence of variants that can completely escape the immune surveillance [ 6 , 8 ]. Several reviews systematically evaluated the effectiveness and/or safety of the three mainstream vaccines on the market (inactivated virus vaccines, RNA vaccines and viral vector vaccines) based on random clinical trials (RCT) yet [ 9 , 10 , 11 , 12 , 13 ].
In general, RNA vaccines are the most effective, followed by viral vector vaccines and inactivated virus vaccines [ 10 , 11 , 12 , 13 ]. The current safety of COVID-19 vaccines is acceptable for mass vaccination, but long-term monitoring of vaccine safety is needed, especially in older people with underlying conditions [ 9 , 10 , 11 , 12 , 13 ]. Inactivated vaccines had the lowest incidence of adverse events and the safety comparisons between mRNA vaccines and viral vectors were controversial [ 9 , 10 ].
RCTs usually conduct under a very demanding research circumstance, and tend to be highly consistent and limited in terms of population characteristics and experimental conditions. Actually, real-world studies differ significantly from RCTs in terms of study conditions and mass vaccination in real world requires taking into account factors, which are far more complex, such as widely heterogeneous populations, vaccine supply, willingness, medical accessibility, etc. Therefore, the real safety and effectiveness of vaccines turn out to be a major concern of international community. The results of a mass vaccination of CoronaVac in Chile demonstrated a protective effectiveness of 65.9% against the onset of COVID-19 after complete vaccination procedures [ 14 ], while the outcomes of phase 3 trials in Brazil and Turkey were 50.7% and 91.3%, reported on Sinovac’s website [ 14 ]. As for the Delta variant, the British claimed 88% protection after two doses of BNT162b2, compared with 67% for AZD1222 [ 15 ]. What is surprising is that the protection of BNT162b2 against infection in Israel is only 39% [ 16 ]. Several studies reported the effectiveness and safety of the COVID-19 vaccine in the real world recently, but the results remain controversial [ 17 , 18 , 19 , 20 ]. A comprehensive meta-analysis based upon the real-world studies is still in an urgent demand, especially for evaluating the effect of vaccines on variation strains. In the present study, we aimed to systematically evaluate the effectiveness and safety of the COVID-19 vaccine in the real world and to establish a reliable evidence-based basis for the actual protective effect of the COVID-19 vaccines, especially in the ensuing waves of infections dominated by variants.
Search strategy and selection criteria
Our methods were described in detail in our published protocol [PROSPERO (Prospective register of systematic reviews) registration, CRD42021267110]. We searched eligible studies published by 22 July 2021, from three databases including PubMed, Embase and Web of Science by the following search terms: (effectiveness OR safety) AND (COVID-19 OR coronavirus OR SARS-CoV-2) AND (vaccine OR vaccination). We used EndNoteX9.0 (Thomson ResearchSoft, Stanford, USA) to manage records, screen and exclude duplicates. This study was strictly performed according to the Preferred Reporting Items for Systematic Reviews and Meta-Analyses (PRISMA).
We included observational studies that examined the effectiveness and safety of severe acute respiratory syndrome coronavirus 2 (SARS-CoV-2) vaccines among people vaccinated with SARS-CoV-2 vaccines. The following studies were excluded: (1) irrelevant to the subject of the meta-analysis, such as studies that did not use SARS-CoV-2 vaccination as the exposure; (2) insufficient data to calculate the rate for the prevention of COVID-19, the prevention of hospitalization, the prevention of admission to the ICU, the prevention of COVID-19-related death, or adverse events after vaccination; (3) duplicate studies or overlapping participants; (4) RCT studies, reviews, editorials, conference papers, case reports or animal experiments; and (5) studies that did not clarify the identification of COVID-19.
Studies were identified by two investigators (LQ and QCY) independently following the criteria above, while discrepancies reconciled by a third investigator (LJ).
Data extraction and quality assessment
The primary outcome was the effectiveness of SARS-CoV-2 vaccines. The following data were extracted independently by two investigators (LQ and QCY) from the selected studies: (1) basic information of the studies, including first author, publication year and study design; (2) characteristics of the study population, including sample sizes, age groups, setting or locations; (3) kinds of the SARS-CoV-2 vaccines; (4) outcomes for the effectiveness of SARS-CoV-2 vaccines: the number of laboratory-confirmed COVID-19, hospitalization for COVID-19, admission to the ICU for COVID-19, and COVID-19-related death; and (5) outcomes for the safety of SARS-CoV-2 vaccines: the number of adverse events after vaccination.
We evaluated the risk of bias using the Newcastle–Ottawa quality assessment scale for cohort studies and case–control studies [ 21 ]. and assess the methodological quality using the checklist recommended by Agency for Healthcare Research and Quality (AHRQ) [ 22 ]. Cohort studies and case–control studies were classified as having low (≥ 7 stars), moderate (5–6 stars), and high risk of bias (≤ 4 stars) with an overall quality score of 9 stars. For cross-sectional studies, we assigned each item of the AHRQ checklist a score of 1 (answered “yes”) or 0 (answered “no” or “unclear”), and summarized scores across items to generate an overall quality score that ranged from 0 to 11. Low, moderate, and high risk of bias were identified as having a score of 8–11, 4–7 and 0–3, respectively.
Two investigators (LQ and QCY) independently assessed study quality, with disagreements resolved by a third investigator (LJ).
Data synthesis and statistical analysis
We performed a meta-analysis to pool data from included studies and assess the effectiveness and safety of SARS-CoV-2 vaccines by clinical outcomes (rates of the prevention of COVID-19, the prevention of hospitalization, the prevention of admission to the ICU, the prevention of COVID-19-related death, and adverse events after vaccination). Random-effects or fixed-effects models were used to pool the rates and adjusted estimates across studies separately, based on the heterogeneity between estimates ( I 2 ). Fixed-effects models were used if I 2 ≤ 50%, which represented low to moderate heterogeneity and random-effects models were used if I 2 > 50%, representing substantial heterogeneity.
We conducted subgroup analyses to investigate the possible sources of heterogeneity by using vaccine kinds, vaccination status, sample size, and study population as grouping variables. We used the Q test to conduct subgroup comparisons and variables were considered significant between subgroups if the subgroup difference P value was less than 0.05. Publication bias was assessed by funnel plot and Egger’s regression test. We analyzed data using Stata version 16.0 (StataCorp, Texas, USA).
A total of 4844 records were searched from the three databases. 2484 duplicates were excluded. After reading titles and abstracts, we excluded 2264 reviews, RCT studies, duplicates and other studies meeting our exclude criteria. Among the 96 studies under full-text review, 41 studies were excluded (Fig. 1 ). Ultimately, with three grey literatures included, this final meta-analysis comprised 58 eligible studies, including 32 studies [ 14 , 15 , 17 , 18 , 19 , 20 , 23 , 24 , 25 , 26 , 27 , 28 , 29 , 30 , 31 , 32 , 33 , 34 , 35 , 36 , 37 , 38 , 39 , 40 , 41 , 42 , 43 , 44 , 45 , 46 , 47 , 48 ] for vaccine effectiveness and 26 studies [ 49 , 50 , 51 , 52 , 53 , 54 , 55 , 56 , 57 , 58 , 59 , 60 , 61 , 62 , 63 , 64 , 65 , 66 , 67 , 68 , 69 , 70 , 71 , 72 , 73 , 74 ] for vaccine safety. Characteristics of included studies are showed in Additional file 1 : Table S1, Additional file 2 : Table S2. The risk of bias of all studies we included was moderate or low.
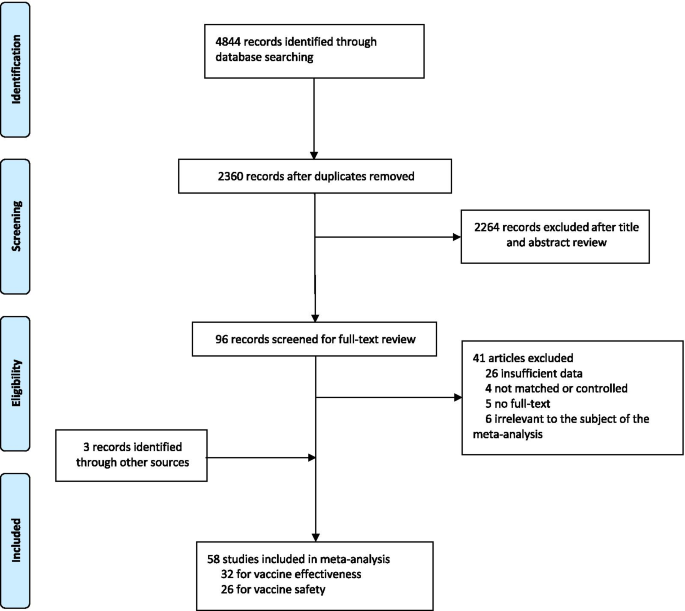
Flowchart of the study selection
Vaccine effectiveness for different clinical outcomes of COVID-19
We separately reported the vaccine effectiveness (VE) by the first and second dose of vaccines, and conducted subgroup analysis by the days after the first or second dose (< 7 days, ≥ 7 days, ≥ 14 days, and ≥ 21 days; studies with no specific days were classified as 1 dose, 2 dose or ≥ 1 dose).
For the first dose of SARS-CoV-2 vaccines, the pooled VE was 41% (95% CI : 28–54%) for the prevention of SARS-CoV-2 infection, 52% (95% CI : 31–73%) for the prevention of symptomatic COVID-19, 66% (95% CI : 50–81%) for the prevention of hospital admissions, 45% (95% CI : 42–49%) for the prevention of ICU admissions, and 53% (95% CI : 15–91%) for the prevention of COVID-19-related death (Table 1 ). The subgroup, ≥ 21 days after the first dose, was found to have the highest VE in each clinical outcome of COVID-19, regardless of ≥ 1 dose group (Table 1 ).
For the second dose of SARS-CoV-2 vaccines, the pooled VE was 85% (95% CI : 81–89%) for the prevention of SARS-CoV-2 infection, 97% (95% CI : 97–98%) for the prevention of symptomatic COVID-19, 93% (95% CI: 89–96%) for the prevention of hospital admissions, 96% (95% CI : 93–98%) for the prevention of ICU admissions, and 95% (95% CI : 92–98%) for the prevention of COVID-19-related death (Table 1 ). VE was 94% (95% CI : 78–98%) in ≥ 21 days after the second dose for the prevention of SARS-CoV-2 infection, higher than other subgroups, regardless of 2 dose group (Table 1 ). For the prevention of symptomatic COVID-19, VE was also relatively higher in 21 days after the second dose (99%, 95% CI : 94–100%). Subgroups showed no statistically significant differences in the prevention of hospital admissions, ICU admissions and COVID-19-related death (subgroup difference P values were 0.991, 0.414, and 0.851, respectively).
Vaccine effectiveness for different variants of SARS-CoV-2 in fully vaccinated people
In the fully vaccinated groups (over 14 days after the second dose), the pooled VE was 85% (95% CI: 80–91%) for the prevention of Alpha variant of SARS-CoV-2 infection, 54% (95% CI : 35–74%) for the Gamma variant, and 74% (95% CI : 62–85%) for the Delta variant. There was only one study [ 23 ] focused on the Beta variant, which showed the VE was 75% (95% CI : 71–79%) for the prevention of the Beta variant of SARS-CoV-2 infection. BNT162b2 vaccine had the highest VE in each variant group; 92% (95% CI : 90–94%) for the Alpha variant, 62% (95% CI : 2–88%) for the Gamma variant, and 84% (95% CI : 75–92%) for the Delta variant (Fig. 2 ).
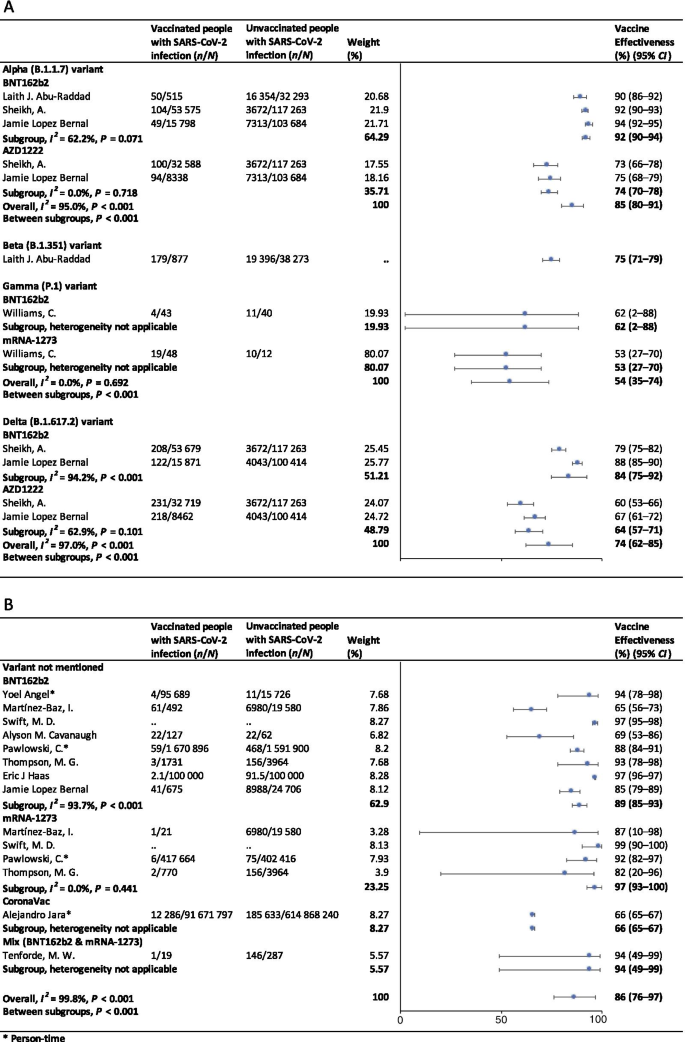
Forest plots for the vaccine effectiveness of SARS-CoV-2 vaccines in fully vaccinated populations. A Vaccine effectiveness against SARS-CoV-2 variants; B Vaccine effectiveness against SARS-CoV-2 with variants not mentioned. SARS-CoV-2 severe acute respiratory syndrome coronavirus 2, COVID-19 coronavirus disease 2019, CI confidence interval
For studies which had not mentioned the variant of SARS-CoV-2, the pooled VE was 86% (95% CI: 76–97%) for the prevention of SARS-CoV-2 infection in fully vaccinated people. mRNA-1273 vaccine had the highest pooled VE (97%, 95% CI: 93–100%, Fig. 2 ).
Safety of SARS-CoV-2 vaccines
As Table 2 showed, the incidence rate of adverse events varied widely among different studies. We conducted subgroup analysis by study population (general population, patients and healthcare workers), vaccine type (BNT162b2, mRNA-1273, CoronaVac, and et al.), and population size (< 1000, 1000–10 000, 10 000–100 000, and > 100 000). The overall pooled incidence rate was 1.5% (95% CI : 1.4–1.6%) for adverse events, 0.4 (95% CI : 0.2–0.5) per 10 000 for severe adverse events, and 0.1 (95% CI : 0.1–0.2) per 10 000 for death after vaccination. Incidence rate of adverse events was higher in healthcare workers (53.2%, 95% CI : 28.4–77.9%), AZD1222 vaccine group (79.6%, 95% CI : 60.8–98.3%), and < 1000 population size group (57.6%, 95% CI : 47.9–67.4%). Incidence rate of sever adverse events was higher in healthcare workers (127.2, 95% CI : 62.7–191.8, per 10 000), Gam-COVID-Vac vaccine group (175.7, 95% CI : 77.2–274.2, per 10 000), and 1000–10 000 population size group (336.6, 95% CI : 41.4–631.8, per 10 000). Incidence rate of death after vaccination was higher in patients (7.6, 95% CI : 0.0–32.2, per 10 000), BNT162b2 vaccine group (29.8, 95% CI : 0.0–71.2, per 10 000), and < 1000 population size group (29.8, 95% CI : 0.0–71.2, per 10 000). Subgroups of general population, vaccine type not mentioned, and > 100 000 population size had the lowest incidence rate of adverse events, severe adverse events, and death after vaccination.
Sensitivity analysis and publication bias
In the sensitivity analyses, VE for SARS-CoV-2 infections, symptomatic COVID-19 and COVID-19-related death got relatively lower when omitting over a single dose group of Maria et al.’s work [ 33 ]; when omitting ≥ 14 days after the first dose group and ≥ 14 days after the second dose group of Alejandro et al.’s work [ 14 ], VE for SARS-CoV-2 infections, hospitalization, ICU admission and COVID-19-related death got relatively higher; and VE for all clinical status of COVID-19 became lower when omitting ≥ 14 days after the second dose group of Eric et al.’s work [ 34 ]. Incidence rate of adverse events and severe adverse events got relatively higher when omitting China CDC’s data [ 74 ]. P values of Egger’s regression test for all the meta-analysis were more than 0.05, indicating that there might not be publication bias.
To our knowledge, this is a comprehensive systematic review and meta-analysis assessing the effectiveness and safety of SARS-CoV-2 vaccines based on real-world studies, reporting pooled VE for different variants of SARS-CoV-2 and incidence rate of adverse events. This meta-analysis comprised a total of 58 studies, including 32 studies for vaccine effectiveness and 26 studies for vaccine safety. We found that a single dose of SARS-CoV-2 vaccines was about 40–60% effective at preventing any clinical status of COVID-19 and that two doses were 85% or more effective. Although vaccines were not as effective against variants of SARS-CoV-2 as original virus, the vaccine effectiveness was still over 50% for fully vaccinated people. Normal adverse events were common, while the incidence of severe adverse events or even death was very low, providing reassurance to health care providers and to vaccine recipients and promote confidence in the safety of COVID-19 vaccines. Our findings strengthen and augment evidence from previous review [ 75 ], which confirmed the effectiveness of the BNT162b2 mRNA vaccine, and additionally reported the safety of SARS-CoV-2 vaccines, giving insight on the future of SARS-CoV-2 vaccine schedules.
Although most vaccines for the prevention of COVID-19 are two-dose vaccines, we found that the pooled VE of a single dose of SARS-CoV-2 vaccines was about 50%. Recent study showed that the T cell and antibody responses induced by a single dose of the BNT162b2 vaccine were comparable to those naturally infected with SARE-CoV-2 within weeks or months after infection [ 76 ]. Our findings could help to develop vaccination strategies under certain circumstances such as countries having a shortage of vaccines. In some countries, in order to administer the first dose to a larger population, the second dose was delayed for up to 12 weeks [ 77 ]. Some countries such as Canada had even decided to delay the second dose for 16 weeks [ 78 ]. However, due to a suboptimum immune response in those receiving only a single dose of a vaccine, such an approach had a chance to give rise to the emergence of variants of SARS-CoV-2 [ 79 ]. There remains a need for large clinical trials to assess the efficacy of a single-dose administration of two-dose vaccines and the risk of increasing the emergence of variants.
Two doses of SARS-CoV-2 vaccines were highly effective at preventing hospitalization, severe cases and deaths resulting from COVID-19, while the VE of different groups of days from the second vaccine dose showed no statistically significant differences. Our findings emphasized the importance of getting fully vaccinated, for the fact that most breakthrough infections were mild or asymptomatic. A recent study showed that the occurrence of breakthrough infections with SARS-CoV-2 in fully vaccinated populations was predictable with neutralizing antibody titers during the peri-infection period [ 80 ]. We also found getting fully vaccinated was at least 50% effective at preventing SARS-CoV-2 variants infections, despite reduced effectiveness compared with original virus; and BNT162b2 vaccine was found to have the highest VE in each variant group. Studies showed that the highly mutated variants were indicative of a form of rapid, multistage evolutionary jumps, which could preferentially occur in the milieu of partial immune control [ 81 , 82 ]. Therefore, immunocompromised patients should be prioritized for anti-COVID-19 immunization to mitigate persistent SARS-CoV-2 infections, during which multimutational SARS-CoV-2 variants could arise [ 83 ].
Recently, many countries, including Israel, the United States, China and the United Kingdom, have introduced a booster of COVID-19 vaccine, namely the third dose [ 84 , 85 , 86 , 87 ]. A study of Israel showed that among people vaccinated with BNT162b2 vaccine over 60 years, the risk of COVID-19 infection and severe illness in the non-booster group was 11.3 times (95% CI: 10.4–12.3) and 19.5 times (95% CI: 12.9–29.5) than the booster group, respectively [ 84 ]. Some studies have found that the third dose of Moderna, Pfizer-BioNTech, Oxford-AstraZeneca and Sinovac produced a spike in infection-blocking neutralizing antibodies when given a few months after the second dose [ 85 , 87 , 88 ]. In addition, the common adverse events associated with the third dose did not differ significantly from the symptoms of the first two doses, ranging from mild to moderate [ 85 ]. The overall incidence rate of local and systemic adverse events was 69% (57/97) and 20% (19/97) after receiving the third dose of BNT162b2 vaccine, respectively [ 88 ]. Results of a phase 3 clinical trial involving 306 people aged 18–55 years showed that adverse events after receiving a third dose of BNT162b2 vaccine (5–8 months after completion of two doses) were similar to those reported after receiving a second dose [ 85 ]. Based on V-safe, local reactions were more frequently after dose 3 (5323/6283; 84.7%) than dose 2 (5249/6283; 83.5%) among people who received 3 doses of Moderna. Systemic reactions were reported less frequently after dose 3 (4963/6283; 79.0%) than dose 2 (5105/6283; 81.3%) [ 86 ]. On August 4, WHO called for a halt to booster shots until at least the end of September to achieve an even distribution of the vaccine [ 89 ]. At this stage, the most important thing we should be thinking about is how to reach a global cover of people at risk with the first or second dose, rather than focusing on the third dose.
Based on real world studies, our results preliminarily showed that complete inoculation of COVID-19 vaccines was still effective against infection of variants, although the VE was generally diminished compared with the original virus. Particularly, the pooled VE was 54% (95% CI : 35–74%) for the Gamma variant, and 74% (95% CI : 62–85%) for the Delta variant. Since the wide spread of COVID-19, a number of variants have drawn extensive attention of international community, including Alpha variant (B.1.1.7), first identified in the United Kingdom; Beta variant (B.1.351) in South Africa; Gamma variant (P.1), initially appeared in Brazil; and the most infectious one to date, Delta variant (B.1.617.2) [ 90 ]. Israel recently reported a breakthrough infection of SARS-CoV-2, dominated by variant B.1.1.7 in a small number of fully vaccinated health care workers, raising concerns about the effectiveness of the original vaccine against those variants [ 80 ]. According to an observational cohort study in Qatar, VE of the BNT162b2 vaccine against the Alpha (B.1.1.7) and Beta (B.1.351) variants was 87% (95% CI : 81.8–90.7%) and 75.0% (95% CI : 70.5–7.9%), respectively [ 23 ]. Based on the National Immunization Management System of England, results from a recent real-world study of all the general population showed that the AZD1222 and BNT162b2 vaccines protected against symptomatic SARS-CoV-2 infection of Alpha variant with 74.5% (95% CI : 68.4–79.4%) and 93.7% (95% CI : 91.6–95.3%) [ 15 ]. In contrast, the VE against the Delta variant was 67.0% (95% CI : 61.3–71.8%) for two doses of AZD1222 vaccine and 88% (95% CI : 85.3–90.1%) for BNT162b2 vaccine [ 15 ].
In terms of adverse events after vaccination, the pooled incidence rate was very low, only 1.5% (95% CI : 1.4–1.6%). However, the prevalence of adverse events reported in large population (population size > 100 000) was much lower than that in small to medium population size. On the one hand, the vaccination population in the small to medium scale studies we included were mostly composed by health care workers, patients with specific diseases or the elderly. And these people are more concerned about their health and more sensitive to changes of themselves. But it remains to be proved whether patients or the elderly are more likely to have adverse events than the general. Mainstream vaccines currently on the market have maintained robust safety in specific populations such as cancer patients, organ transplant recipients, patients with rheumatic and musculoskeletal diseases, pregnant women and the elderly [ 54 , 91 , 92 , 93 , 94 ]. A prospective study by Tal Goshen-lag suggests that the safety of BNT162b2 vaccine in cancer patients is consistent with those previous reports [ 91 ]. In addition, the incidence rate of adverse events reported in the heart–lung transplant population is even lower than that in general population [ 95 ]. On the other hand, large scale studies at the national level are mostly based on national electronic health records or adverse event reporting systems, and it is likely that most mild or moderate symptoms are actually not reported.
Compared with the usual local adverse events (such as pain at the injection site, redness at the injection site, etc.) and normal systemic reactions (such as fatigue, myalgia, etc.), serious and life-threatening adverse events were rare due to our results. A meta-analysis based on RCTs only showed three cases of anaphylactic shock among 58 889 COVID-19 vaccine recipients and one in the placebo group [ 11 ]. The exact mechanisms underlying most of the adverse events are still unclear, accordingly we cannot establish a causal relation between severe adverse events and vaccination directly based on observational studies. In general, varying degrees of adverse events occur after different types of COVID-19 vaccination. Nevertheless, the benefits far outweigh the risks.
Our results showed the effectiveness and safety of different types of vaccines varied greatly. Regardless of SARS-CoV-2 variants, vaccine effectiveness varied from 66% (CoronaVac [ 14 ]) to 97% (mRNA-1273 [ 18 , 20 , 45 , 46 ]). The incidence rate of adverse events varied widely among different types of vaccines, which, however, could be explained by the sample size and population group of participants. BNT162b2, AZD1222, mRNA-1273 and CoronaVac were all found to have high vaccine efficacy and acceptable adverse-event profile in recent published studies [ 96 , 97 , 98 , 99 ]. A meta-analysis, focusing on the potential vaccine candidate which have reached to the phase 3 of clinical development, also found that although many of the vaccines caused more adverse events than the controls, most were mild, transient and manageable [ 100 ]. However, severe adverse events did occur, and there remains the need to implement a unified global surveillance system to monitor the adverse events of COVID-19 vaccines around the world [ 101 ]. A recent study employed a knowledge-based or rational strategy to perform a prioritization matrix of approved COVID-19 vaccines, and led to a scale with JANSSEN (Ad26.COV2.S) in the first place, and AZD1222, BNT162b2, and Sputnik V in second place, followed by BBIBP-CorV, CoronaVac and mRNA-1273 in third place [ 101 ]. Moreover, when deciding the priority of vaccines, the socioeconomic characteristics of each country should also be considered.
Our meta-analysis still has several limitations. First, we may include limited basic data on specific populations, as vaccination is slowly being promoted in populations under the age of 18 or over 60. Second, due to the limitation of the original real-world study, we did not conduct subgroup analysis based on more population characteristics, such as age. When analyzing the efficacy and safety of COVID-19 vaccine, we may have neglected the discussion on the heterogeneity from these sources. Third, most of the original studies only collected adverse events within 7 days after vaccination, which may limit the duration of follow-up for safety analysis.
Based on the real-world studies, SARS-CoV-2 vaccines have reassuring safety and could effectively reduce the death, severe cases, symptomatic cases, and infections resulting from SARS-CoV-2 across the world. In the context of global pandemic and the continuous emergence of SARS-CoV-2 variants, accelerating vaccination and improving vaccination coverage is still the most important and urgent matter, and it is also the final means to end the pandemic.
Availability of data and materials
All data generated or analyzed during this study are included in this published article and its additional information files.
Abbreviations
Coronavirus disease 2019
Severe Acute Respiratory Syndrome Coronavirus 2
Vaccine effectiveness
Confidence intervals
Intensive care unit
Random clinical trials
Preferred reporting items for systematic reviews and meta-analyses
COVID-19 Dashboard by the Center for Systems Science and Engineering (CSSE) at Johns Hopkins University (JHU). 2021. https://coronavirus.jhu.edu/map.html . Accessed 20 Aug 2021.
Barranco R, Rocca G, Molinelli A, Ventura F. Controversies and challenges of mass vaccination against SARS-CoV-2 in Italy: medico-legal perspectives and considerations. Healthcare (Basel). 2021. https://doi.org/10.3390/healthcare9091163 .
Article Google Scholar
COVID-19 vaccine tracker. 2021. https://vac-lshtm.shinyapps.io/ncov_vaccine_landscape/ . Accessed 20 Aug 2021.
Coronavirus (COVID-19) Vaccinations. 2021. https://ourworldindata.org/covid-vaccinations . Accessed 20 Aug 2021.
Kirby T. New variant of SARS-CoV-2 in UK causes surge of COVID-19. Lancet Respir Med. 2021;9(2):e20–1. https://doi.org/10.1016/s2213-2600(21)00005-9 .
Article CAS PubMed PubMed Central Google Scholar
Callaway E. Fast-spreading COVID variant can elude immune responses. Nature. 2021;589(7843):500–1. https://doi.org/10.1038/d41586-021-00121-z .
Article CAS PubMed Google Scholar
Reardon S. How the Delta variant achieves its ultrafast spread. Nature. 2021. https://doi.org/10.1038/d41586-021-01986-w .
Article PubMed Google Scholar
Li R, Liu J, Zhang H. The challenge of emerging SARS-CoV-2 mutants to vaccine development. J Genet Genomics. 2021;48(2):102–6. https://doi.org/10.1016/j.jgg.2021.03.001 .
Article PubMed PubMed Central Google Scholar
Chen M, Yuan Y, Zhou Y, Deng Z, Zhao J, Feng F, Zou H, Sun C. Safety of SARS-CoV-2 vaccines: a systematic review and meta-analysis of randomized controlled trials. Infect Dis Poverty. 2021;10(1):94. https://doi.org/10.1186/s40249-021-00878-5 .
Ling Y, Zhong J, Luo J. Safety and effectiveness of SARS-CoV-2 vaccines: a systematic review and meta-analysis. J Med Virol. 2021. https://doi.org/10.1002/jmv.27203 .
Pormohammad A, Zarei M, Ghorbani S, Mohammadi M, Razizadeh MH, Turner DL, Turner RJ. Efficacy and safety of COVID-19 vaccines: a systematic review and meta-analysis of randomized clinical trials. Vaccines (Basel). 2021. https://doi.org/10.3390/vaccines9050467 .
Sathian B, Asim M, Banerjee I, Roy B, Pizarro AB, Mancha MA, van Teijlingen ER, Kord-Varkaneh H, Mekkodathil AA, Subramanya SH, et al. Development and implementation of a potential coronavirus disease 2019 (COVID-19) vaccine: a systematic review and meta-analysis of vaccine clinical trials. Nepal J Epidemiol. 2021;11(1):959–82. https://doi.org/10.3126/nje.v11i1.36163 .
Yuan P, Ai P, Liu Y, Ai Z, Wang Y, Cao W, Xia X, Zheng JC. Safety, tolerability, and immunogenicity of COVID-19 vaccines: a systematic review and meta-analysis. medRxiv. 2020. https://doi.org/10.1101/2020.11.03.20224998 .
Jara A, Undurraga EA, González C, Paredes F, Fontecilla T, Jara G, Pizarro A, Acevedo J, Leo K, Leon F, et al. Effectiveness of an inactivated SARS-CoV-2 vaccine in Chile. N Engl J Med. 2021. https://doi.org/10.1056/NEJMoa2107715 .
Lopez Bernal J, Andrews N, Gower C, Gallagher E, Simmons R, Thelwall S, Stowe J, Tessier E, Groves N, Dabrera G, et al. Effectiveness of COVID-19 vaccines against the B.1.617.2 (Delta) variant. N Engl J Med. 2021. https://doi.org/10.1056/NEJMoa2108891 .
Israel says Pfizer Covid vaccine is just 39% effective as delta spreads, but still prevents severe illness. 2021. https://www.cnbc.com/2021/07/23/delta-variant-pfizer-covid-vaccine-39percent-effective-in-israel-prevents-severe-illness.html . Accessed 20 Aug 2021.
Zacay G, Shasha D, Bareket R, Kadim I, Hershkowitz Sikron F, Tsamir J, Mossinson D, Heymann AD. BNT162b2 vaccine effectiveness in preventing asymptomatic infection with SARS-CoV-2 virus: a nationwide historical cohort study. Open Forum Infect Dis. 2021;8(6): ofab262. https://doi.org/10.1093/ofid/ofab262 .
Martínez-Baz I, Miqueleiz A, Casado I, Navascués A, Trobajo-Sanmartín C, Burgui C, Guevara M, Ezpeleta C, Castilla J. Effectiveness of COVID-19 vaccines in preventing SARS-CoV-2 infection and hospitalisation, Navarre, Spain, January to April 2021. Eurosurveillance. 2021. https://doi.org/10.2807/1560-7917.Es.2021.26.21.2100438 .
Tenforde MW, Olson SM, Self WH, Talbot HK, Lindsell CJ, Steingrub JS, Shapiro NI, Ginde AA, Douin DJ, Prekker ME, et al. Effectiveness of Pfizer-BioNTech and moderna vaccines against COVID-19 among hospitalized adults aged ≥65 years—United States, January–March 2021. MMWR Morb Mortal Wkly Rep. 2021;70(18):674–9. https://doi.org/10.15585/mmwr.mm7018e1 .
Pawlowski C, Lenehan P, Puranik A, Agarwal V, Venkatakrishnan AJ, Niesen MJM, O’Horo JC, Virk A, Swift MD, Badley AD, et al. FDA-authorized mRNA COVID-19 vaccines are effective per real-world evidence synthesized across a multi-state health system. Med (N Y). 2021. https://doi.org/10.1016/j.medj.2021.06.007 .
Wells G, Shea B, O'Connell D, Peterson J, Welch V, Losos M, Tugwell P. The Newcastle-Ottawa Scale (NOS) for assessing the quality of nonrandomised studies in meta-analyses. http://www.ohri.ca/programs/clinical_epidemiology/oxford.asp . Accessed 20 Aug 2021.
Rostom A, Dubé C, Cranney A, et al. Celiac Disease. Rockville (MD): Agency for Healthcare Research and Quality (US); 2004 Sep. (Evidence Reports/Technology Assessments, No. 104.) Appendix D. Quality Assessment Forms. Available from: https://www.ncbi.nlm.nih.gov/books/NBK35156/ . Accessed 20 Aug 2021
Abu-Raddad LJ, Chemaitelly H, Butt AA. Effectiveness of the BNT162b2 COVID-19 vaccine against the B.1.1.7 and B.1.351 Variants. N Engl J Med. 2021;385(2):187–9. https://doi.org/10.1056/NEJMc2104974 .
Angel Y, Spitzer A, Henig O, Saiag E, Sprecher E, Padova H, Ben-Ami R. Association between vaccination with BNT162b2 and incidence of symptomatic and asymptomatic SARS-CoV-2 infections among health care workers. JAMA. 2021;325(24):2457–65. https://doi.org/10.1001/jama.2021.7152 .
Azamgarhi T, Hodgkinson M, Shah A, Skinner JA, Hauptmannova I, Briggs TWR, Warren S. BNT162b2 vaccine uptake and effectiveness in UK healthcare workers—a single centre cohort study. Nat Commun. 2021;12(1):3698. https://doi.org/10.1038/s41467-021-23927-x .
Bianchi FP, Germinario CA, Migliore G, Vimercati L, Martinelli A, Lobifaro A, Tafuri S, Stefanizzi P. BNT162b2 mRNA COVID-19 vaccine effectiveness in the prevention of SARS-CoV-2 infection: a preliminary report. J Infect Dis. 2021. https://doi.org/10.1093/infdis/jiab262 .
Britton A, Jacobs Slifka KM, Edens C, Nanduri SA, Bart SM, Shang N, Harizaj A, Armstrong J, Xu K, Ehrlich HY, et al. Effectiveness of the Pfizer-BioNTech COVID-19 vaccine among residents of two skilled nursing facilities experiencing COVID-19 outbreaks—Connecticut, December 2020–February 2021. MMWR Morb Mortal Wkly Rep. 2021;70(11):396–401. https://doi.org/10.15585/mmwr.mm7011e3 .
Cavanaugh AM, Fortier S, Lewis P, Arora V, Johnson M, George K, Tobias J, Lunn S, Miller T, Thoroughman D, et al. COVID-19 outbreak associated with a SARS-CoV-2 R1 lineage variant in a skilled nursing facility after vaccination program—Kentucky, March 2021. MMWR Morb Mortal Wkly Rep. 2021;70(17):639–43. https://doi.org/10.15585/mmwr.mm7017e2 .
Chemaitelly H, Yassine HM, Benslimane FM, Al Khatib HA, Tang P, Hasan MR, Malek JA, Coyle P, Ayoub HH, Al Kanaani Z, et al. mRNA-1273 COVID-19 vaccine effectiveness against the B.1.1.7 and B.1.351 variants and severe COVID-19 disease in Qatar. Nat Med. 2021. https://doi.org/10.1038/s41591-021-01446-y .
Chodick G, Tene L, Patalon T, Gazit S, Ben Tov A, Cohen D, Muhsen K. Assessment of effectiveness of 1 dose of BNT162b2 vaccine for SARS-CoV-2 infection 13 to 24 days after immunization. JAMA Netw Open. 2021;4(6): e2115985. https://doi.org/10.1001/jamanetworkopen.2021.15985 .
Chodick G, Tene L, Rotem RS, Patalon T, Gazit S, Ben-Tov A, Weil C, Goldshtein I, Twig G, Cohen D, et al. The effectiveness of the TWO-DOSE BNT162b2 vaccine: analysis of real-world data. Clin Infect Dis. 2021. https://doi.org/10.1093/cid/ciab438 .
Dagan N, Barda N, Kepten E, Miron O, Perchik S, Katz MA, Hernán MA, Lipsitch M, Reis B, Balicer RD. BNT162b2 mRNA COVID-19 vaccine in a nationwide mass vaccination setting. N Engl J Med. 2021;384(15):1412–23. https://doi.org/10.1056/NEJMoa2101765 .
Flacco ME, Soldato G, Acuti Martellucci C, Carota R, Di Luzio R, Caponetti A, Manzoli L. Interim estimates of COVID-19 vaccine effectiveness in a mass vaccination setting: data from an Italian Province. VacCInes (Basel). 2021. https://doi.org/10.3390/vaccines9060628 .
Haas EJ, Angulo FJ, McLaughlin JM, Anis E, Singer SR, Khan F, Brooks N, Smaja M, Mircus G, Pan K, et al. Impact and effectiveness of mRNA BNT162b2 vaccine against SARS-CoV-2 infections and COVID-19 cases, hospitalisations, and deaths following a nationwide vaccination campaign in Israel: an observational study using national surveillance data. Lancet. 2021;397(10287):1819–29. https://doi.org/10.1016/s0140-6736(21)00947-8 .
Hall VJ, Foulkes S, Saei A, Andrews N, Oguti B, Charlett A, Wellington E, Stowe J, Gillson N, Atti A, et al. COVID-19 vaccine coverage in health-care workers in England and effectiveness of BNT162b2 mRNA vaccine against infection (SIREN): a prospective, multicentre, cohort study. Lancet. 2021;397(10286):1725–35. https://doi.org/10.1016/s0140-6736(21)00790-x .
Hyams C, Marlow R, Maseko Z, King J, Ward L, Fox K, Heath R, Tuner A, Friedrich Z, Morrison L, et al. Effectiveness of BNT162b2 and ChAdOx1 nCoV-19 COVID-19 vaccination at preventing hospitalisations in people aged at least 80 years: a test-negative, case-control study. Lancet Infect Dis. 2021. https://doi.org/10.1016/s1473-3099(21)00330-3 .
Khan N, Mahmud N. Effectiveness of SARS-CoV-2 vaccination in a veterans affairs cohort of patients with inflammatory bowel disease with diverse exposure to immunosuppressive medications. Gastroenterology. 2021. https://doi.org/10.1053/j.gastro.2021.05.044 .
Knobel P, Serra C, Grau S, Ibañez R, Diaz P, Ferrández O, Villar R, Lopez AF, Pujolar N, Horcajada JP, et al. COVID-19 mRNA vaccine effectiveness in asymptomatic healthcare workers. Infect Control Hosp Epidemiol. 2021. https://doi.org/10.1017/ice.2021.287 .
Lopez Bernal J, Andrews N, Gower C, Robertson C, Stowe J, Tessier E, Simmons R, Cottrell S, Roberts R, O’Doherty M, et al. Effectiveness of the Pfizer-BioNTech and Oxford-AstraZeneca vaccines on covid-19 related symptoms, hospital admissions, and mortality in older adults in England: test negative case-control study. BMJ. 2021;373: n1088. https://doi.org/10.1136/bmj.n1088 .
Mazagatos C, Monge S, Olmedo C, Vega L, Gallego P, Martín-Merino E, Sierra MJ, Limia A, Larrauri A. Effectiveness of mRNA COVID-19 vaccines in preventing SARS-CoV-2 infections and COVID-19 hospitalisations and deaths in elderly long-term care facility residents, Spain, weeks 53, 2020 to 13 2021. Eurosurveillance. 2021. https://doi.org/10.2807/1560-7917.Es.2021.26.24.2100452 .
Pilishvili T, Fleming-Dutra KE, Farrar JL, Gierke R, Mohr NM, Talan DA, Krishnadasan A, Harland KK, Smithline HA, Hou PC, et al. Interim estimates of vaccine effectiveness of Pfizer-BioNTech and Moderna COVID-19 vaccines among health care personnel—33 US Sites, January–March 2021. MMWR Morb Mortal Wkly Rep. 2021;70(20):753–8. https://doi.org/10.15585/mmwr.mm7020e2 .
Sheikh A, McMenamin J, Taylor B, Robertson C. SARS-CoV-2 Delta VOC in Scotland: demographics, risk of hospital admission, and vaccine effectiveness. Lancet. 2021;397(10293):2461–2. https://doi.org/10.1016/s0140-6736(21)01358-1 .
Shrotri M, Krutikov M, Palmer T, Giddings R, Azmi B, Subbarao S, Fuller C, Irwin-Singer A, Davies D, Tut G, et al. Vaccine effectiveness of the first dose of ChAdOx1 nCoV-19 and BNT162b2 against SARS-CoV-2 infection in residents of long-term care facilities in England (VIVALDI): a prospective cohort study. Lancet Infect Dis. 2021. https://doi.org/10.1016/s1473-3099(21)00289-9 .
Skowronski DM, Setayeshgar S, Zou M, Prystajecky N, Tyson JR, Galanis E, Naus M, Patrick DM, Sbihi H, El Adam S, et al. Single-dose mRNA vaccine effectiveness against SARS-CoV-2, including Alpha and Gamma variants: a test-negative design in adults 70 years and older in British Columbia,Canada. Clin Infect Dis. 2021. https://doi.org/10.1093/cid/ciab616 .
Swift MD, Breeher LE, Tande AJ, Tommaso CP, Hainy CM, Chu H, Murad MH, Berbari EF, Virk A. Effectiveness of mRNA COVID-19 vaccines against SARS-CoV-2 infection in a cohort of healthcare personnel. Clin Infect Dis. 2021. https://doi.org/10.1093/cid/ciab361 .
Thompson MG, Burgess JL, Naleway AL, Tyner H, Yoon SK, Meece J, Olsho LEW, Caban-Martinez AJ, Fowlkes AL, Lutrick K, et al. Prevention and attenuation of COVID-19 with the BNT162b2 and mRNA-1273 Vaccines. N Engl J Med. 2021. https://doi.org/10.1056/NEJMoa2107058 .
Vasileiou E, Simpson CR, Shi T, Kerr S, Agrawal U, Akbari A, Bedston S, Beggs J, Bradley D, Chuter A, et al. Interim findings from first-dose mass COVID-19 vaccination roll-out and COVID-19 hospital admissions in Scotland: a national prospective cohort study. Lancet. 2021;397(10285):1646–57. https://doi.org/10.1016/s0140-6736(21)00677-2 .
Williams C, Al-Bargash D, Macalintal C, Stuart R, Seth A, Latham J, Gitterman L, Fedsin S, Godoy M, Kozak R, et al. COVID-19 outbreak associated with a SARS-CoV-2 P.1 lineage in a long-term care home after implementation of a vaccination program—Ontario, April–May 2021. Clin Infect Dis. 2021. https://doi.org/10.1093/cid/ciab617 .
Alhazmi A, Alamer E, Daws D, Hakami M, Darraj M, Abdelwahab S, Maghfuri A, Algaissi A. Evaluation of side effects associated with COVID-19 vaccines in Saudi Arabia. Vaccines (Basel). 2021. https://doi.org/10.3390/vaccines9060674 .
Andrzejczak-Grządko S, Czudy Z, Donderska M. Side effects after COVID-19 vaccinations among residents of Poland. Eur Rev Med Pharmacol Sci. 2021;25(12):4418–21. https://doi.org/10.26355/eurrev_202106_26153 .
Baldolli A, Michon J, Appia F, Galimard C, Verdon R, Parienti JJ. Tolerance of BNT162b2 mRNA COVI-19 vaccine in patients with a medical history of COVID-19 disease: a case control study. Vaccine. 2021;39(32):4410–3. https://doi.org/10.1016/j.vaccine.2021.06.054 .
Cherian S, Paul A, Ahmed S, Alias B, Manoj M, Santhosh AK, Varghese DR, Krishnan N, Shenoy P. Safety of the ChAdOx1 nCoV-19 and the BBV152 vaccines in 724 patients with rheumatic diseases: a post-vaccination cross-sectional survey. Rheumatol Int. 2021;41(8):1441–5. https://doi.org/10.1007/s00296-021-04917-0 .
Chevallier P, Coste-Burel M, Le Bourgeois A, Peterlin P, Garnier A, Béné MC, Imbert BM, Drumel T, Le Gouill S, Moreau P, et al. Safety and immunogenicity of a first dose of SARS-CoV-2 mRNA vaccine in allogeneic hematopoietic stem-cells recipients. EJHaem. 2021. https://doi.org/10.1002/jha2.242 .
Connolly CM, Ruddy JA, Boyarsky BJ, Avery RK, Werbel WA, Segev DL, Garonzik-Wang J, Paik JJ. Safety of the first dose of mRNA SARS-CoV-2 vaccines in patients with rheumatic and musculoskeletal diseases. Ann Rheum Dis. 2021. https://doi.org/10.1136/annrheumdis-2021-220231 .
Furer V, Eviatar T, Zisman D, Peleg H, Paran D, Levartovsky D, Zisapel M, Elalouf O, Kaufman I, Meidan R, et al. Immunogenicity and safety of the BNT162b2 mRNA COVID-19 vaccine in adult patients with autoimmune inflammatory rheumatic diseases and in the general population: a multicentre study. Ann Rheum Dis. 2021. https://doi.org/10.1136/annrheumdis-2021-220647 .
Gee J, Marquez P, Su J, Calvert GM, Liu R, Myers T, Nair N, Martin S, Clark T, Markowitz L, et al. First month of COVID-19 vaccine safety monitoring—United States, December 14, 2020–January 13, 2021. MMWR Morb Mortal Wkly Rep. 2021;70(8):283–8. https://doi.org/10.15585/mmwr.mm7008e3 .
Hashimoto T, Ozaki A, Bhandari D, Sawano T, Sah R, Tanimoto T. High anaphylaxis rates following vaccination with the Pfizer BNT162b2 mRNA vaccine against COVID-19 in Japanese health care workers; a secondary analysis of initial post-approval safety data. J Travel Med. 2021. https://doi.org/10.1093/jtm/taab090 .
Lv G, Yuan J, Xiong X, Li M. Mortality rate and characteristics of deaths following COVID-19 vaccination. Front Med (Lausanne). 2021;8: 670370. https://doi.org/10.3389/fmed.2021.670370 .
McMurry R, Lenehan P, Awasthi S, Silvert E, Puranik A, Pawlowski C, Venkatakrishnan AJ, Anand P, Agarwal V, O’Horo JC, et al. Real-time analysis of a mass vaccination effort confirms the safety of FDA-authorized mRNA COVID-19 vaccines. Med (N Y). 2021. https://doi.org/10.1016/j.medj.2021.06.006 .
Monin L, Laing AG, Muñoz-Ruiz M, McKenzie DR, Del Molino Del Barrio I, Alaguthurai T, Domingo-Vila C, Hayday TS, Graham C, Seow J, et al. Safety and immunogenicity of one versus two doses of the COVID-19 vaccine BNT162b2 for patients with cancer: interim analysis of a prospective observational study. Lancet Oncol. 2021;22(6):765–78. https://doi.org/10.1016/s1470-2045(21)00213-8 .
Pagotto V, Ferloni A, Mercedes Soriano M, Díaz M, Braguinsky Golde N, González MI, Asprea V, Staneloni MI, Zingoni P, Vidal G, et al. Active monitoring of early safety of Sputnik V vaccine in Buenos Aires, Argentina. MediCIna (B Aires). 2021;81(3):408–14.
Google Scholar
Peled Y, Ram E, Lavee J, Sternik L, Segev A, Wieder-Finesod A, Mandelboim M, Indenbaum V, Levy I, Raanani E, et al. BNT162b2 vaccination in heart transplant recipients: Clinical experience and antibody response. J Heart Lung Transplant. 2021. https://doi.org/10.1016/j.healun.2021.04.003 .
Quiroga B, Sánchez-Álvarez E, Goicoechea M, de Sequera P. COVID-19 vaccination among Spanish nephrologists: acceptance and side effects. J Healthc Qual Res. 2021. https://doi.org/10.1016/j.jhqr.2021.05.002 .
Ram R, Hagin D, Kikozashvilli N, Freund T, Amit O, Bar-On Y, Beyar-Katz O, Shefer G, Moshiashvili MM, Karni C, et al. Safety and immunogenicity of the BNT162b2 mRNA COVID-19 vaccine in patients after allogeneic HCT or CD19-based CART therapy—a single center prospective cohort study. Transplant Cell Ther. 2021. https://doi.org/10.1016/j.jtct.2021.06.024 .
Revon-Riviere G, Ninove L, Min V, Rome A, Coze C, Verschuur A, de Lamballerie X, André N. The BNT162b2 mRNA COVID-19 vaccine in adolescents and young adults with cancer: a monocentric experience. Eur J Cancer. 2021;154:30–4. https://doi.org/10.1016/j.ejca.2021.06.002 .
Riad A, Pokorná A, Mekhemar M, Conrad J, Klugarová J, Koščík M, Klugar M, Attia S. Safety of ChAdOx1 nCoV-19 vaccine: independent evidence from two EU states. Vaccines (Basel). 2021. https://doi.org/10.3390/vaccines9060673 .
Riad A, Sağıroğlu D, Üstün B, Pokorná A, Klugarová J, Attia S, Klugar M. Prevalence and risk factors of CoronaVac Side effects: an independent cross-sectional study among healthcare workers in Turkey. J Clin Med. 2021. https://doi.org/10.3390/jcm10122629 .
Rosman Y, Lavi N, Meir-Shafrir K, Lachover-Roth I, Cohen-Engler A, Mekori YA, Confino-Cohen R. Safety of BNT162b2 mRNA COVID-19 vaccine in patients with mast cell disorders. J Allergy Clin Immunol Pract. 2021. https://doi.org/10.1016/j.jaip.2021.06.032 .
Signorelli C, Odone A, Gianfredi V, Capraro M, Kacerik E, Chiecca G, Scardoni A, Minerva M, Mantecca R, Musarò P, et al. Application of the “immunization islands” model to improve quality, efficiency and safety of a COVID-19 mass vaccination site. Ann Ig. 2021;33(5):499–512. https://doi.org/10.7416/ai.2021.2456 .
Vallée A, Chan-Hew-Wai A, Bonan B, Lesprit P, Parquin F, Catherinot É, Choucair J, Billard D, Amiel-Taieb C, Camps È, et al. Oxford-AstraZeneca COVID-19 vaccine: need of a reasoned and effective vaccine campaign. Public Health. 2021;196:135–7. https://doi.org/10.1016/j.puhe.2021.05.030 .
Wang J, Hou Z, Liu J, Gu Y, Wu Y, Chen Z, Ji J, Diao S, Qiu Y, Zou S, et al. Safety and immunogenicity of COVID-19 vaccination in patients with non-alcoholic fatty liver disease (CHESS2101): a multicenter study. J Hepatol. 2021. https://doi.org/10.1016/j.jhep.2021.04.026 .
Zhang MX, Zhang TT, Shi GF, Cheng FM, Zheng YM, Tung TH, Chen HX. Safety of an inactivated SARS-CoV-2 vaccine among healthcare workers in China. Expert Rev Vaccines. 2021. https://doi.org/10.1080/14760584.2021.1925112 .
Shay DK, Gee J, Su JR, Myers TR, Marquez P, Liu R, Zhang B, Licata C, Clark TA, Shimabukuro TT. Safety monitoring of the Janssen (Johnson & Johnson) COVID-19 Vaccine—United States, March–April 2021. MMWR Morb Mortal Wkly Rep. 2021;70(18):680–4. https://doi.org/10.15585/mmwr.mm7018e2 .
Prevention CCfDCa. Information analysis of COVID-19 vaccine adverse reaction monitoring in China. 2021-5-28. http://www.chinacdc.cn/jkzt/ymyjz/ymyjjz_6758/202105/t20210528_230908.html . Accessed 20 Aug 2021.
Kow CS, Hasan SS. Real-world effectiveness of BNT162b2 mRNA vaccine: a meta-analysis of large observational studies. Inflammopharmacology. 2021;29(4):1075–90. https://doi.org/10.1007/s10787-021-00839-2 .
Angyal A, Longet S, Moore S, Payne RP, Harding A et al. T-Cell and Antibody Responses to First BNT162b2 Vaccine Dose in Previously SARS-CoV-2-Infected and Infection-Naive UK Healthcare Workers: A Multicentre, Prospective, Observational Cohort Study. Available at SSRN: https://ssrn.com/abstract=3820576 or https://doi.org/10.2139/ssrn.3820576 . Accessed 20 Aug 2021.
Pimenta D, Yates C, Pagel C, Gurdasani D. Delaying the second dose of covid-19 vaccines. BMJ. 2021;372: n710. https://doi.org/10.1136/bmj.n710 .
Tauh T, Mozel M, Meyler P, Lee SM. An updated look at the 16-week window between doses of vaccines in BC for COVID-19. BC Med J. 2021;63(3):102–3.
Kadire SR, Wachter RM, Lurie N. Delayed second dose versus standard regimen for COVID-19 vaccination. N Engl J Med. 2021;384(9): e28. https://doi.org/10.1056/NEJMclde2101987 .
Bergwerk M, Gonen T, Lustig Y, Amit S, Lipsitch M, Cohen C, Mandelboim M, Gal Levin E, Rubin C, Indenbaum V, et al. COVID-19 breakthrough infections in vaccinated health care workers. N Engl J Med. 2021. https://doi.org/10.1056/NEJMoa2109072 .
Truong TT, Ryutov A, Pandey U, Yee R, Goldberg L, Bhojwani D, Aguayo-Hiraldo P, Pinsky BA, Pekosz A, Shen L, et al. Persistent SARS-CoV-2 infection and increasing viral variants in children and young adults with impaired humoral immunity. medRxiv. 2021. https://doi.org/10.1101/2021.02.27.21252099 .
Choi B, Choudhary MC, Regan J, Sparks JA, Padera RF, Qiu X, Solomon IH, Kuo HH, Boucau J, Bowman K, et al. Persistence and evolution of SARS-CoV-2 in an Immunocompromised Host. N Engl J Med. 2020;383(23):2291–3. https://doi.org/10.1056/NEJMc2031364 .
Corey L, Beyrer C, Cohen MS, Michael NL, Bedford T, Rolland M. SARS-CoV-2 variants in patients with immunosuppression. N Engl J Med. 2021;385(6):562–6. https://doi.org/10.1056/NEJMsb2104756 .
Bar-On YM, Goldberg Y, Mandel M, Bodenheimer O, Freedman L, Kalkstein N, Mizrahi B, Alroy-Preis S, Ash N, Milo R, et al. Protection of BNT162b2 vaccine booster against Covid-19 in Israel. N Engl J Med. 2021;385(15):1393–400. https://doi.org/10.1056/NEJMoa2114255 .
Hause AM, Baggs J, Gee J, Marquez P, Myers TR, Shimabukuro TT, Shay DK. Safety monitoring of an additional dose of COVID-19 vaccine—United States, August 12–September 19, 2021. MMWR Morb Mortal Wkly Rep. 2021;70(39):1379–84. https://doi.org/10.15585/mmwr.mm7039e4 .
Furlow B. Immunocompromised patients in the USA and UK should receive third dose of COVID-19 vaccine. Lancet Rheumatol. 2021. https://doi.org/10.1016/s2665-9913(21)00313-1 .
Flaxman A, Marchevsky NG, Jenkin D, Aboagye J, Aley PK, Angus B, Belij-Rammerstorfer S, Bibi S, Bittaye M, Cappuccini F, et al. Reactogenicity and immunogenicity after a late second dose or a third dose of ChAdOx1 nCoV-19 in the UK: a substudy of two randomised controlled trials (COV001 and COV002). Lancet. 2021;398(10304):981–90. https://doi.org/10.1016/s0140-6736(21)01699-8 .
Peled Y, Ram E, Lavee J, Segev A, Matezki S, Wieder-Finesod A, Halperin R, Mandelboim M, Indenbaum V, Levy I, et al. Third dose of the BNT162b2 vaccine in heart transplant recipients: immunogenicity and clinical experience. J Heart Lung Transplant. 2021. https://doi.org/10.1016/j.healun.2021.08.010 .
WHO. WHO press conference on coronavirus disease (COVID-19)—4 August 2021. 2021. https://www.who.int/multi-media/details/who-press-conference-on-coronavirus-disease-(covid-19)---4-august-2021 . Accessed 20 Aug 2021.
Cascella M, Rajnik M, Aleem A, Dulebohn SC, Di Napoli R. Features, evaluation, and treatment of coronavirus (COVID-19). In: StatPearls. edn. Treasure Island (FL): StatPearls Publishing Copyright © 2021, StatPearls Publishing LLC.; 2021.
Goshen-Lago T, Waldhorn I, Holland R, Szwarcwort-Cohen M, Reiner-Benaim A, Shachor-Meyouhas Y, Hussein K, Fahoum L, Baruch M, Peer A, et al. Serologic status and toxic effects of the SARS-CoV-2 BNT162b2 vaccine in patients undergoing treatment for cancer. JAMA Oncol. 2021. https://doi.org/10.1001/jamaoncol.2021.2675 .
Ou MT, Boyarsky BJ, Motter JD, Greenberg RS, Teles AT, Ruddy JA, Krach MR, Jain VS, Werbel WA, Avery RK, et al. Safety and reactogenicity of 2 doses of SARS-CoV-2 vaccination in solid organ transplant recipients. Transplantation. 2021. https://doi.org/10.1097/tp.0000000000003780 .
Bookstein Peretz S, Regev N, Novick L, Nachshol M, Goffer E, Ben-David A, Asraf K, Doolman R, Sapir E, Regev Yochay G, et al. Short-term outcome of pregnant women vaccinated by BNT162b2 mRNA COVID-19 vaccine. Ultrasound Obstet Gynecol. 2021. https://doi.org/10.1002/uog.23729 .
Shimabukuro TT, Kim SY, Myers TR, Moro PL, Oduyebo T, Panagiotakopoulos L, Marquez PL, Olson CK, Liu R, Chang KT, et al. Preliminary findings of mRNA COVID-19 vaccine safety in pregnant persons. N Engl J Med. 2021;384(24):2273–82. https://doi.org/10.1056/NEJMoa2104983 .
Peled Y, Ram E, Lavee J, Sternik L, Segev A, Wieder-Finesod A, Mandelboim M, Indenbaum V, Levy I, Raanani E, et al. BNT162b2 vaccination in heart transplant recipients: clinical experience and antibody response. J Heart Lung Transplant. 2021;40(8):759–62. https://doi.org/10.1016/j.healun.2021.04.003 .
Thomas SJ, Moreira ED Jr, Kitchin N, Absalon J, Gurtman A, Lockhart S, Perez JL, Pérez Marc G, Polack FP, Zerbini C, et al. Safety and efficacy of the BNT162b2 mRNA COVID-19 vaccine through 6 months. N Engl J Med. 2021. https://doi.org/10.1056/NEJMoa2110345 .
Falsey AR, Sobieszczyk ME, Hirsch I, Sproule S, Robb ML, Corey L, Neuzil KM, Hahn W, Hunt J, Mulligan MJ, et al. Phase 3 safety and efficacy of AZD1222 (ChAdOx1 nCoV-19) COVID-19 vaccine. N Engl J Med. 2021. https://doi.org/10.1056/NEJMoa2105290 .
El Sahly HM, Baden LR, Essink B, Doblecki-Lewis S, Martin JM, Anderson EJ, Campbell TB, Clark J, Jackson LA, Fichtenbaum CJ, et al. Efficacy of the mRNA-1273 SARS-CoV-2 vaccine at completion of blinded phase. N Engl J Med. 2021. https://doi.org/10.1056/NEJMoa2113017 .
Tanriover MD, Doğanay HL, Akova M, Güner HR, Azap A, Akhan S, Köse Ş, Erdinç F, Akalın EH, Tabak ÖF, et al. Efficacy and safety of an inactivated whole-virion SARS-CoV-2 vaccine (CoronaVac): interim results of a double-blind, randomised, placebo-controlled, phase 3 trial in Turkey. Lancet. 2021;398(10296):213–22. https://doi.org/10.1016/s0140-6736(21)01429-x .
Kumar S, Saurabh MK, Maharshi V. Efficacy and safety of potential vaccine candidates against coronavirus disease 2019: a systematic review. J Adv Pharm Technol Res. 2021;12(3):215–21. https://doi.org/10.4103/japtr.JAPTR_229_20 .
Burgos-Salcedo J. A rational strategy to support approved COVID-19 vaccines prioritization. Hum Vaccin Immunother. 2021;17(10):3474–7. https://doi.org/10.1080/21645515.2021.1922060 .
Download references
Acknowledgements
This study was funded by the National Natural Science Foundation of China (72122001; 71934002) and the National Science and Technology Key Projects on Prevention and Treatment of Major infectious disease of China (2020ZX10001002). The funders had no role in study design, data collection and analysis, decision to publish, or preparation of the paper. No payment was received by any of the co-authors for the preparation of this article.
Author information
Qiao Liu and Chenyuan Qin are joint first authors
Authors and Affiliations
Department of Epidemiology and Biostatistics, School of Public Health, Peking University, Beijing, 100191, China
Qiao Liu, Chenyuan Qin, Min Liu & Jue Liu
Institute for Global Health and Development, Peking University, Beijing, 100871, China
Chenyuan Qin & Jue Liu
You can also search for this author in PubMed Google Scholar
Contributions
LQ and QCY contributed equally as first authors. LJ and LM contributed equally as correspondence authors. LJ and LM conceived and designed the study; LQ, QCY and LJ carried out the literature searches, extracted the data, and assessed the study quality; LQ and QCY performed the statistical analysis and wrote the manuscript; LJ, LM, LQ and QCY revised the manuscript. All authors read and approved the final manuscript.
Corresponding authors
Correspondence to Min Liu or Jue Liu .
Ethics declarations
Ethics approval and consent to participate.
Not applicable.
Consent for publication
Competing interests.
The authors have no conflicts of interest to declare that are relevant to the content of this article.
Supplementary Information
Additional file 1: table s1..
Characteristic of studies included for vaccine effectiveness.
Additional file 2: Table S2.
Characteristic of studies included for vaccine safety.
Rights and permissions
Open Access This article is licensed under a Creative Commons Attribution 4.0 International License, which permits use, sharing, adaptation, distribution and reproduction in any medium or format, as long as you give appropriate credit to the original author(s) and the source, provide a link to the Creative Commons licence, and indicate if changes were made. The images or other third party material in this article are included in the article's Creative Commons licence, unless indicated otherwise in a credit line to the material. If material is not included in the article's Creative Commons licence and your intended use is not permitted by statutory regulation or exceeds the permitted use, you will need to obtain permission directly from the copyright holder. To view a copy of this licence, visit http://creativecommons.org/licenses/by/4.0/ . The Creative Commons Public Domain Dedication waiver ( http://creativecommons.org/publicdomain/zero/1.0/ ) applies to the data made available in this article, unless otherwise stated in a credit line to the data.
Reprints and permissions
About this article
Cite this article.
Liu, Q., Qin, C., Liu, M. et al. Effectiveness and safety of SARS-CoV-2 vaccine in real-world studies: a systematic review and meta-analysis. Infect Dis Poverty 10 , 132 (2021). https://doi.org/10.1186/s40249-021-00915-3
Download citation
Received : 07 September 2021
Accepted : 01 November 2021
Published : 14 November 2021
DOI : https://doi.org/10.1186/s40249-021-00915-3
Share this article
Anyone you share the following link with will be able to read this content:
Sorry, a shareable link is not currently available for this article.
Provided by the Springer Nature SharedIt content-sharing initiative
- Effectiveness
- Meta-analysis
Infectious Diseases of Poverty
ISSN: 2049-9957
- Submission enquiries: Access here and click Contact Us
- General enquiries: [email protected]
SYSTEMATIC REVIEW article
How has research on the effectiveness and safety of covid-19 vaccination been evaluated: a scope review with emphasis on coronavac.
- 1 Grupo de Epidemiología, Universidad de Antioquia, Medellín, Colombia
- 2 Grupo de Enfermedades Tropicales y Resistencia Bacteriana, Universidad del Sinú, Montería, Colombia
- 3 Grupo de investigación EMAP - Estadística y Matemáticas Aplicadas, Pontificia Universidad Javeriana, Cali, Colombia
- 4 Grupo de Investigación, Secretaría de Salud Distrital, Cali, Colombia
- 5 Grupo de Investigación Clínica - PECET (GIC-PECET), Universidad de Antioquia, Medellín, Colombia
- 6 Grupo de Investigación en Economía, Gestión y Salud, ECGESA. Pontificia Universidad Javeriana, Cali, Colombia
- 7 Departamento de Salud pública y Epidemiología, Pontificia Universidad Javeriana, Cali, Colombia
Introduction: The control of the COVID-19 epidemic has been focused on the development of vaccines against SARS-CoV-2. All developed vaccines have reported safety and efficacy results in preventing infection and its consequences, although the quality of evidence varies depending on the vaccine considered. Different methodological designs have been used for their evaluation, which can influence our understanding of the effects of these interventions. CoronaVac is an inactivated vaccine, and it has been assessed in various studies, including clinical trials and observational studies. Given these differences, our objective was to explore the published information to answer the question: how has the efficacy/effectiveness and safety of CoronaVac been evaluated in different studies? This is to identify potential gaps and challenges to be addressed in understanding its effect.
Methods: A scoping review was carried out following the methodology proposed by the Joanna Briggs Institute, which included studies carried out in humans as of 2020, corresponding to systematic reviews, clinical trials, analytical or descriptive observational studies, in which the effectiveness and/or safety of vaccines for COVID19 were evaluated or described. There were no age restrictions for the study participants.
Results: The efficacy/effectiveness and safety of this vaccine was assessed through 113 studies. Nineteen corresponded to experimental studies, 7 of Phase II, 5 of Phase IV, and 4 were clinical trials with random assignment. Although some clinical trials with random assignment have been carried out, these have limitations in terms of feasibility, follow-up times, and with this, the possibility of evaluating safety outcomes that occur with low frequencies. Not all studies have used homogeneous methods of analysis. Both the prevention of infection, and the prevention of outcomes such as hospitalization or death, have been valued through similar outcomes, but some through multivariate analysis of dependencies, and others through analysis that try to infer causally through different control methods of confounding.
Conclusion: Published information on the evaluation of the efficacy/effectiveness and safety of the CoronaVac is abundant. However, there are differences in terms of vaccine application schedules, population definition, outcomes evaluated, follow-up times, and safety assessment, as well as non-standardization in the reporting of results, which may hinder the generalizability of the findings. It is important to generate meetings and consensus strategies for the methods and reporting of this type of studies, which will allow to reduce the heterogeneity in their presentation and a better understanding of the effect of these vaccines.
1 Introduction
Starting from the first reports coming from China and from countries in Europe and Asia, about the infection produced by SARS-CoV-2, its high contagion, and lethality of up to 14% in older adults, and the subsequent declaration of a COVID-19 pandemic, and together with the measures established by the healthcare authorities to manage the disease, efforts began to develop effective and safe vaccines that would contribute to speeding up the control of this health condition, through the reduction of infections, complications, and deaths associated with this disease ( 1 ).
For this reason, pandemic control efforts have focused on developing vaccines against SARS-CoV-2 that are capable of acting against infection, disease, or transmission, and thus contribute to disease control ( 2 ). In this context, different research groups have developed vaccines using different platforms, including mRNA, viral vectors, and inactivated viruses ( 3 ).
Unlike most drugs, whose benefits are limited to the individual taking them, vaccines have the potential to produce far-reaching effects on general public health and well-being, cognitive development, and, ultimately, economic productivity ( 4 ). However, the global advances in vaccination coverage achieved during the first years of the 21st century have been threatened by the emergence of anti-vaccination groups that have questioned vaccine efficacy to create public distrust of vaccines and immunization programs. This requires an adequate and conscious evaluation of both the efficacy/effectiveness and the different aspects that can affect the safety of the people who receive them ( 5 ).
In general, vaccines that have gained approval for human use have been effective in preventing COVID-19, particularly in preventing severe disease and death. However, reports on their implementation are mainly based on follow-up studies of the adult population ( 6 ). Additionally, if the vaccination prevents symptoms from developing and asymptomatic infections are less likely to be discovered than symptomatic ones, it is feasible that the effectiveness against any infection has been overstated. A competitive tendency toward underestimate arises when estimates are based on tests with inadequate specificity, particularly when testing are conducted more frequently than has been estimated for various COVID-19 vaccinations ( 7 ).
All vaccines seem to be safe and efficacious against all variations of interest in preventing hospitalization, death, and severe COVID-19; however, the quality of the data differs significantly between the vaccines under consideration ( 8 ).
Different methodological designs have been used to evaluate the effectiveness and safety of vaccines for COVID-19. Most clinical trials were carried out before the appearance of variants of concern, and the duration, subgroups evaluated, and analysis methods were not homogeneous between vaccines, creating uncertainty about some effects and comparisons ( 9 ).
CoronaVac is an inactivated whole-virus vaccine against COVID-19 adjuvanted with aluminum hydroxide created from African green monkey kidney cells (Vero cells) inoculated with SARS-CoV-2 (strain CN02). The Chinese company Sinovac Biotech developed the vaccine, and on June 1, 2021, the World Health Organization (WHO) approved the vaccine for emergency use ( 10 ). Using two 3 μg doses of CoronaVac, the overall efficacy for avoiding symptomatic COVID-19 (before the emergence of concerning variations) has been assessed at 67.7% (95%CI: 35.9 to 83.7%) ( 10 ). Compared to COVID-19 prevention, its impact in preventing hospital stays, ICU admissions, and fatalities has been much stronger. Three-dose regimens have also been shown to raise seroconversion levels of neutralizing antibodies, even against variants like Omicron. Few serious vaccine-related adverse reactions have been reported ( 10 ).
However, given the differences that may exist in the methods used to assess the efficacy, effectiveness, and safety of vaccines against COVID-19, our objective was to explore the published research on COVID-19 vaccines, focusing on CoronaVac, in order to answer the question: How has the efficacy/effectiveness and safety of CoronaVac been assessed in different designs and study phases of the vaccines used to control COVID-19?
A scoping review was carried out under a protocol registered in the Open Science Framework (OSF; osf.io/aeut4), and following the methodology proposed by the Joanna Briggs Institute ( 11 ), which included studies carried out in humans as of 2020, corresponding to systematic reviews, clinical trials, and analytical or descriptive observational studies in which the effectiveness and/or safety of vaccines for COVID19 were evaluated or described. There were no age restrictions on the study participants.
Abstracts from congresses were not evaluated because they had not been subjected to systematic peer evaluation at the time, nor were studies published in languages other than English or Spanish.
2.1 Search methods for study identification
To identify potentially relevant articles for review, the following databases were searched, starting from 2020: MEDLINE, EMBASE, LILACS, Scopus, and Cochrane.
The following valid strategy was used for MEDLINE through PubMed and then adapted to other databases:
(((SARS-CoV-2[MeSH Terms]) OR (COVID-19[MeSH Terms])) OR (Coronavirus[MeSH Terms])) AND ((COVID-19 Vaccines[MeSH Terms]) OR (Coronavirus vaccines[Title/Abstract])).
The full search strategy is presented in the Supplementary material .
2.2 Study selection
The initial screening of the studies was independently performed by two reviewers in pairs (PA-AG and PR-SR). The RIS files of each database were uploaded to Rayyan software ( 12 ). Disagreements were resolved by a third author (JA).
Both reviewers assessed all titles and abstracts and excluded those considered irrelevant for the review, those not meeting the inclusion criteria, or because they were duplicates. Subsequently, 15 reviewers independently (JA, PA, DA, AC, AG, LL, LM, DO, GQ, SR, CR, PR, MS, CT, MA) evaluated the full text of the studies to verify the eligibility criteria. A cross-review was carried out for studies evaluating CoronaVac by four reviewers (PA, AG, PR, and SR).
2.3 Variable
Of the definitively selected studies, the following variables were extracted in a paired form: (i) type of study, (ii) population studied, (iii) intervention (vaccine) evaluated, (iv) control, (v) follow-up time, (vi) efficacy and/or effectiveness outcomes, and (vii) safety outcomes.
2.4 Data synthesis
For each outcome, a description of the results was made following the description in the document and/or Supplementary material of the article.
3.1 Study selection
The search identified 42,813 titles for the initial evaluation, of which 40,372 were excluded after a review of the title, abstract, and possible duplication. A total of 2,441 full texts were reviewed to verify the eligibility criteria, of which 1,685 were included in the synthesis ( Figure 1 ; Supplementary material ).
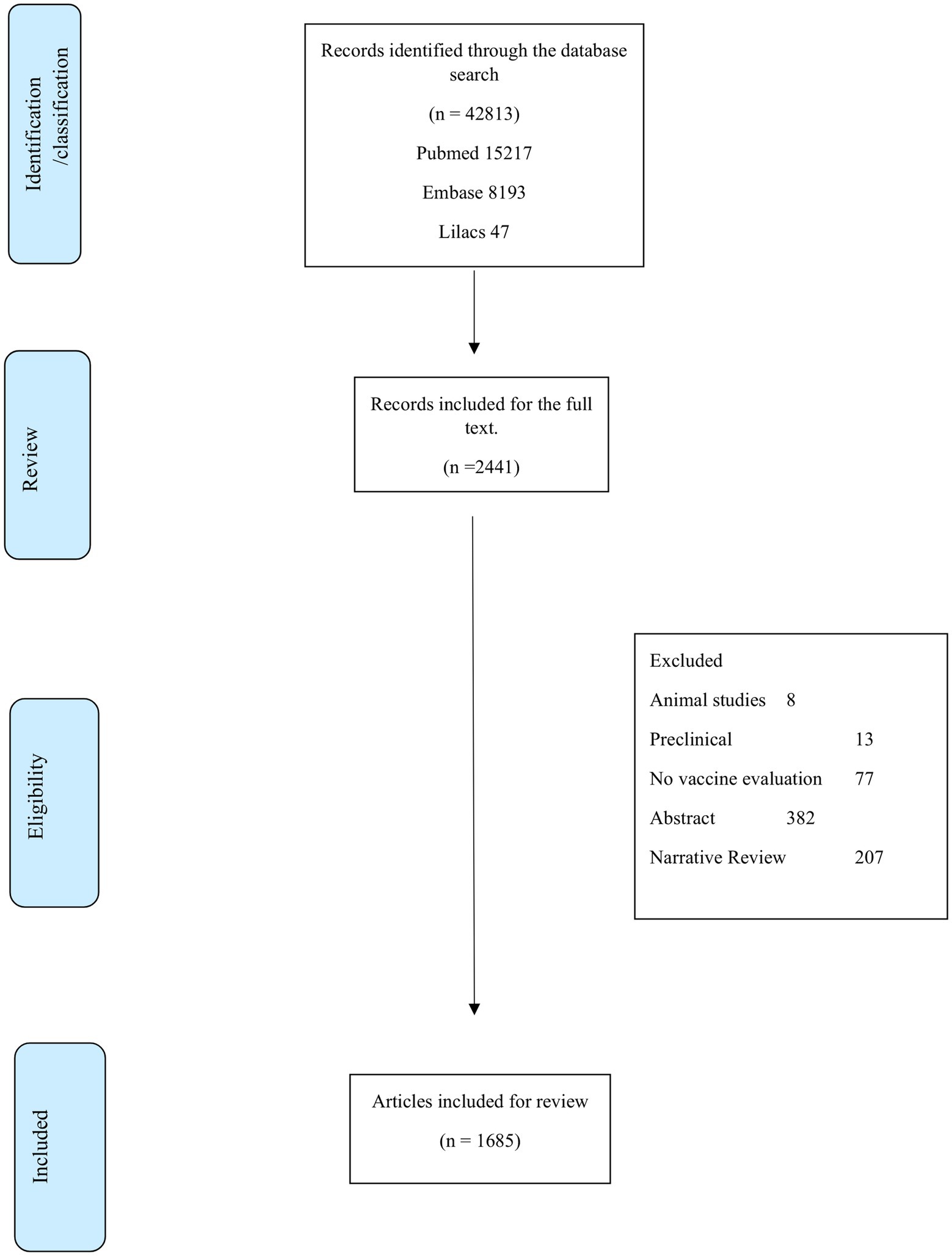
Figure 1 . Flow diagram of the literature review process.
3.2 Synthesis of the results
One hundred vaccines were evaluated through randomized clinical trials (RCT). The other studies corresponded to observational studies, 705 (43.9%) analytical studies, and mainly cohort studies (467; 29.1%). Three hundred and seventy-seven patients (23.5%) were series or case reports.
One hundred twenty-six studies (7.8%) did not specify the vaccine evaluated. Other studies have evaluated one or more specific vaccines. Seven hundred thirty-two studies did not include a vaccine or a control group. Two hundred and thirty-eight evaluated several types of vaccines, and 160 compared a vaccine against a placebo. The number of patients or vaccine doses evaluated in each study went from one (case report) to 306,473,169 doses of applied vaccines ( 13 ).
Regarding the population assessed, 44.4% of the studies evaluated the effects of vaccines on adults. 3.4% in adults and adolescents, 2% in adolescents, 1.2% in immunosuppressed individuals, 1.2% in children, 0.9% in pregnant women, and 0.25% in people living with HIV. The overall monitoring time ranged from hours to 6 months; this difference occurred between studies that evaluated immunological outcomes, which could occur within hours or days, and those that evaluated clinical outcomes.
A total of 15.1% of the studies evaluated the effectiveness or efficacy of vaccines by evaluating their effects on preventing infection, hospitalization, or death from infection. 59.1% of the studies corresponded to the description of safety events. The events were described heterogeneously. In some studies, they are only recorded as “mild adverse events” or “mild systemic events.” Few studies reported specific events such as myocarditis, and hepatic or allergic alterations. Of the studies, 25.8% described immunological outcomes, 368 studies through the measurement of antibodies, and 64 through the effects mediated by cellular immunity.
3.2.1 CoronaVac
The efficacy, effectiveness, and safety of this vaccine have been assessed in 113 studies. Nineteen corresponded to experimental studies, seven of Phase II, five of Phase IV, and four were clinical trials with random assignment, carried out in adults in Chile, Indonesia, and Turkey ( 14 – 17 ), comparing the effect of the vaccine versus placebo. The other studies were observational studies, most of which were case reports, case series, or descriptions of cohorts. Of these, 45.1% were conducted in Asia, 23% in Latin America, and 22.1% in Europe, mainly in Turkey (of 27/29 European studies).
As for the population, 87.6% of the studies were conducted in adults, while the representation of studies in pregnant women, children, immunosuppressed people, or people living with HIV ranged between 0.9 and 3.5% of the studies.
Sixty studies (53.1%) evaluated the effect of CoronaVac in a control group. The others were case reports or descriptions of cohorts without comparison. Of these, 42 (70%) described events in patients who received CoronaVac and another vaccine, without performing an effectiveness or efficacy analysis. Other studies evaluated the efficacy and effectiveness by measuring the effect of preventing hospitalization, death, or COVID. Of the total, 34 studies evaluated CoronaVac (30.1%) and described some immune outcomes.
Although the objective of the review was not to assess the effectiveness of the vaccine, but rather how it has been evaluated, the results of some of the identified studies are shown below in order to present relevant information about the methods used and possible differences between them, which lead to discussing the effect that this can have on the analysis and use of CoronaVac and other vaccines. More details on the results of the identified studies can be found in the Supplementary material section.
3.2.2 Efficacy/effectiveness of CoronaVac
3.2.2.1 prevention of covid-19.
Cheng et al. ( 18 ) evaluated the effectiveness of BNT162B2 and CoronaVac in patients with chronic kidney disease in Hong Kong. 28,374 people were not vaccinated, 27,129 received two doses of BNT162b2, and 47,640 received two doses of CoronaVac in this retrospective cohort analysis. Following inverse probability of treatment weighting with 1% extreme values, a cohort that was well-balanced and had a standardized mean difference of less than 0.1 was generated.
The effectiveness of CoronaVac on Turkish healthcare professionals was assessed by Can et al. ( 19 ). 4,067 medical personnel worked at a University Hospital in Istanbul, where this retrospective cohort study was carried out. In the fully vaccinated group, the follow-up period was defined as beginning 14 days following the second dose. If PCR test findings were positive or the trial came to an end, healthcare personnel were excluded. Healthcare personnel who were not vaccinated were prohibited from participating in any COVID-19 vaccination. The vaccine’s unadjusted and adjusted effectiveness were calculated using the incidence rate ratio and Cox regression. 29% of the healthcare staff had not received any vaccinations, whereas 71% had received all recommended doses.
Jara et al. ( 20 ) conducted an evaluation of a prospective, observational, national-level cohort of individuals (≥ 16 years) associated with the Fondo Nacional de Salud insurance program in Chile. They used individual-level data to assess the efficacy of booster vaccines, namely BNT162B2 (Pfizer-Biontech), AZD1222 (Oxford-AstraZeneca), and CoronaVac (Syovac Biotech), in individuals who had completed a primary immunization schedule with CoronaVac, in comparison to those who had not received any vaccinations. The hazard ratios were estimated using inverse probability-weighted and stratified survival regression models that took into account the time-varying vaccination status and adjusted for pertinent clinical, socioeconomic, and demographic confounders. An estimate was made of the change in risk associated with the primary immunization series and booster shot from being unvaccinated to vaccinated. 11,174,257 persons in total fulfilled the trial’s eligibility conditions; of these, 4,127,546 finished the two doses of the CoronaVac primary immunization regimen and got a booster dose during the study period. 2,019,260 (48.9%) individuals received a BNT162b2 booster, 186,946 (4.5%) received a homologous booster with CoronaVac, and 1,921,340 (46–5%) participants received an AZD1222 booster. The weighted stratified Cox model was utilized to compute the modified vaccination efficacy in preventing COVID-19.
Utilizing hospitalization, vaccination, and National COVID-19 notification data, Cerqueira-Silva et al. ( 21 ) conducted a case–control study in Brazil to evaluate the efficacy of four vaccines (CoronaVac [synovac], ChAdOx1 nCoV-19 [AstraZeneca], Ad26.COV2.S [Janssen], and BNT162b2 [Pfizer-Bionntech]) in individuals with laboratory-confirmed prior SARS-COV-2 infection. The probabilities of test positivity and the likelihood of hospitalization or death from COVID-19 were compared based on vaccination status and the amount of time that had passed from the first or second dose of vaccinations using multivariable conditional logistic regression.
The same authors conducted a similar study in Brazil ( 22 ), using linked national Brazilian databases to conduct a negative-test design study with nearly 14 million participants (~ 16 million tests) to estimate the effectiveness of the CoronaVac vaccine over time and the BNT162B2 booster vaccination against severe COVID-19 outcomes (hospitalization or death) and severe acute respiratory syndrome, as confirmed by RT-PCR (SARS-COV-2).
To evaluate the effectiveness of homologous and heterologous boosters against COVID-19 in the context of OMICRON, Ranzani et al. ( 23 ) conducted a nationwide case–control study (with negative PCR results) to assess homologous and heterologous (BNT162B2) booster doses in adults who received two doses of CoronaVac in Brazil in the OMICRON context.
A case–control research was carried out in Thailand by Sritipsukho et al. ( 24 ) to assess the efficacy of various vaccination regimens in preventing COVID-19 during the time when the delta variant was the predominant causing virus (≥ 95%). By correcting for individual demographic and clinical factors, the efficacy of vaccines was assessed.
3.2.2.2 Prevention of hospitalization and death
Cheng et al. ( 18 ) found that both vaccines reduced hospitalization and death related to COVID-19, which was the opposite of the outcome of preventing COVID-19 infection. The vaccination efficacy for BNT162b2 users was 64% (95% CI: 57–69%) for hospitalization associated to COVID-19 and 86% (95% CI: 80–90%) for COVID-19-related death. Regarding hospitalization and death associated to COVID-19, the vaccine efficacy for CoronaVac was 44% (95% CI: 37–49%) and 70% (95% CI: 64–75%), respectively.
In the Jara et al. ( 20 ) study, the adjusted effectiveness of the vaccine against hospitalization due to COVID-19, ICU admission, and death was 86.3% (83.7–88.5), 92.2% (88.7–94.6), and 86.7% (80.5–91.0) for a CoronaVac homolog booster; 96.1% (95.3–96.9), 96, 2% (94.6–97.3), and 96.8% (93.9–98.3) for a BNT162b2 booster; and 97.7% (97.3–98.0), 98.9% (98.5–99.2), and 98.1% (97.3–98.6) for an AZD1222 booster, respectively.
In Brazil ( 21 ), the effectiveness against hospitalization or death 14 or more days after the completion of the vaccination schedule was 81.3% (75.3–85.8) for CoronaVac, 89.9% (83.5–93.8) for ChAdOx1 nCoV-19, and 57.7% (−2.6–82.5) for Ad26.COV2.S, and 89.7% (54.3–97.7) for BNT162b2.
3.2.2.3 Immunological outcomes
Bueno et al. ( 14 ), conducting a randomized placebo-controlled clinical trial in Chile, assessed the effectiveness of CoronaVac by assigning participants to either a placebo or two doses of CoronaVac spaced 2 weeks apart. Enrollments totaled 434, with 397 individuals in the 18–59 age range and 37 in the 60+ age range. 81 subjects had hemoral assessments. 2 and 4 weeks after the second dosage, respectively, the seroconversion rates for specific anti-S1-receptor binding domain (RBD) immunoglobulin G (IgG) were 82.22 and 84.44% in the 18–59 years age group and 62, 69 and 70.37% in the ≥60 years age group. A notable rise in the amount of neutralizing antibodies in circulation was noted two and 4 weeks following the second dosage. 47 participants had their cells evaluated. After stimulation with Mega Pools of SARS-CoV-2 peptides, a notable increase in T cell responses was seen, as evidenced by the release of interferon-γ (IFN-γ).
According to Zeng et al. ( 25 ) the following were the findings of two single-center, double-blind, randomized, placebo-controlled phase II clinical trials: adults from Jiangsu, China, aged 18 to 59 years were first assigned (1:1) into two vaccination schedule cohorts: one for the days 0 and 14 of vaccination (cohort 1), and another for the days 0 and 28 of vaccination (cohort 2). Each cohort was then randomly assigned (2:2:1) to either a placebo group or a 3 μg or 6 μg dose of CoronaVac. A third dose was given to half of the participants in each cohort 6 months after the second dose, and an additional dose was given to the other half of the individuals 28 days following the second dose, as a result of a protocol revision. In a separate phase II experiment carried out in Hebei, China, individuals who met the eligibility criteria of 60 years or above were randomized to receive three injections of 1.5, 3, or 6 μg of vaccine or a placebo. The first two doses of the vaccine were given 28 days apart, while the second and third doses were given 6 months apart. For the per-protocol population (those who finished their allotted third dose), the primary research outcomes were geometric mean titers (GMTs), geometric mean increments (GMIs), and seropositivity of neutralizing antibodies to SARS-CoV-2. Out of the 600 participants, who were between the ages of 18 and59, 540 (90%) were qualified for a third dose. Of these, 269 (50%) received the third primary dose (cohorts 1a-14d-2 m and 2a-28d-2 m) 2 months after the second dose, and 271 (50%) received a booster dose 8 months later (cohorts 1b-14d-8 m and 2b-28d-8 m). For the 1b-14d-8 m cohort ( n = 53; GMT 3.9 [95% CI 3.1–5.0]) and 2b-28d-8 m cohort ( n = 49; GMT 6.8 [95% CI 5.2–8.8]), neutralizing antibody titers elicited by the first two treatments in the 3 μg group declined after 6 months to close or below the seropositive cut-off point (GMT of 8). The GMTs measured 14 days later increased to 137.9 (95% CI: 99.9–190.4) for the 1b-14d-8 m cohort and to 143.1 (110.8–184.7) 28 days later for the 2b-28d-8 m cohort when a booster dose was administered 8 months following a second dose. After the principal third dosage, GMTs increased somewhat in cohorts 1a-14d-2 m (n = 54) and 2a-28d-2 m ( n = 53). In cohort 1a, GMTs increased from 21.8 (95% CI: 17.3–27.6) on day 28 after the second dose to 45.8 (35.7–58.9) on day 28 after the third dose. Six months following the third dose, GMTs had dropped to almost the positive threshold: in the 1a-14d-2 m group, they were 9.2 (95% CI 7.1–12.0), while in the 2a-28d-2 m cohort, they were 10.0 (7.3–13.7). Similarly, 6 months following the initial two-dose series, neutralizing antibody titers dropped to almost or below the seropositive threshold among people 60 years of age or older who received booster doses (303 [87%] of 350 participants were eligible for a third dosage). Eight months following the second treatment, which markedly raised neutralizing antibody concentrations, a third dose was administered: After the second dose on day 28, GMTs climbed to 42.9 (95% CI: 31.0–59.4), and after the third dose on day 28 ( n = 29), GMTs increased to 158.5 (96.6–259.2).
Chantasrisawad et al. ( 26 ) assessed healthy children aged 5 to 11 who were given two intramuscular doses of either Covilo or CoronaVac and 10 μg of BNT162b2. Neutralizing antibodies against the Omicron version were assessed using a pseudovirus neutralization test (pVNT, ID50) and a surrogate viral neutralization test (sVNT, % inhibition) 14–21 days following the booster. The antibody responses were contrasted with those of a concurrent cohort of kids who got two BNT162b2 doses separated by 3 weeks. A total of 59 children, consisting of 20 CoronaVac recipients and 39 Covilo recipients, were registered between April and May 2022, with a mean age (SD) of 8.5 years (1.7). The primary series’ median interval was 49 days, with an interquartile range of 33–51. Following the booster, the geometric means (MG) of pVNT and sVNT were 499 (95%CI: 399–624) and 72.2% inhibition (95%CI: 67.2–77.6), respectively. From zero to 72 %, the percentage of kids with sVNT against Omicron strain ≥68% inhibition rose. In comparison to the parallel cohort, the geometric mean ratios (GMR) of sVNT and pVNT were 4.3 and 12.2, respectively. In comparison to children who received a booster dosage between 4 and 6 weeks, the GMR of sVNT and pVNT among those who received it at a time interval of more than 6 weeks was 1.2 (95% CI: 1.1–1.3) and 1.8 (95% CI 1.2–2.7).
In Turkey, ( 27 ) et al. assessed the variables influencing the antibody response in 235 adults over 65 years of age following two doses of the inactivated SARS-CoV-2 vaccination (CoronaVac). Four weeks following the first and second vaccination doses, the mean levels of anti-SARS-CoV-2 IgG antibodies were 37.70 ± 57.08 IU/mL and 194.61 ± 174.88 IU/mL, respectively. Additionally, 4 weeks following the first vaccination dose, 134 out of 235 participants (57.02%) had an antibody level of less than 25.6 IU/mL (negative); 4 weeks following the second vaccination dose, this percentage was 11.48% ( n = 27). Eight participants (29.6%) had no comorbidities, while 19 (70.4%) with an antibody level less than 25.6 IU/mL 4 weeks after the first dose of the vaccination had at least one comorbid condition, including diabetes mellitus ( F = 2.352, p = 0.006). Individuals with comorbidities and those 65 years of age or older showed lower antibody response rates.
Demirbakan et al. ( 28 ) examined the presence of immunoglobulin G antibodies in the receptor-binding region of the S1 subunit of the SARS-CoV-2 spike protein in 1072 healthcare workers following immunization in a descriptive observational research. 28 days, 21 days, and 3 months following the first, second, and second dosages, respectively, were the times at which blood samples were taken. Anti-spike antibodies were found in 834/1072 (77.8%) subjects 4 weeks following the initial vaccination dose. Between 18 and 34 years of age, seropositivity was observed to be greater in both men and women (84.6%) compared to 70.6% ( p < 0.001) in the former group. In 1008 of 1,012 (99.6%) cases, anti-spike antibodies were found 21 days after the second dose, and in 803 of 836 (96.1%) cases, anti-spike antibodies were found 3 months later.
3.2.2.4 Safety
According to Bueno et al. ( 14 ) in their placebo-controlled clinical trial, pain at the injection site was the primary adverse reaction in 434 volunteers, and it occurred more frequently in the vaccine arm than in the placebo arm. The majority of the negative effects that were seen were modest and limited. No significant negative events were noted.
The frequency of adverse reactions was reported by Zeng et al. ( 25 ) without providing any additional effect measurements. In every immunization group, all adverse responses that were reported within 28 days after the third dose were classified as either grade 1 or 2. In the 1a-14d-2 m cohort, 150 participants reported three serious adverse events (2%); in the 1b-14d-8 m cohort, 150 participants reported four (3%); in the 2a-28d-2 m and 2b-28d-8 m cohorts, 150 participants reported one (1%); overall 349 people reported 24 (7%) serious adverse events.
Cheng et al. ( 18 ) observed an incidence rate of any adverse events of special interest following the first vaccination dose of 34.28 (95% CI: 29.81–39.23) and 38.39 (95% CI: 34.81–42.23) per 10,000 doses of BNT162b2 and CoronaVac, respectively, in their retrospective cohort of patients with chronic kidney disease. BNT162b2 (incidence rate ratio [95% CI]: first dose: 0.86 [0.69–1.08]; second dose: 0.96 [0.76–1.22]; third dose: 0.60 [0.33–1.10]) and CoronaVac (incidence rate ratio [95% CI]: first dose: 0.76 [0.64–0.91]; second dose: 0.86 [0.71–1.05]; third dose: 0.74 [0.36–1.54]) did not show an increased risk of overall adverse event of special interest when compared to the baseline period.
4 Discussion
The COVID-19 pandemic has affected the world’s population with a high morbidity and mortality rate. Recent reports have described persistent symptoms that extend beyond the initial period of the disease. It has been observed that adverse consequences, in addition to respiratory effects, are produced at different levels: cardiovascular, neurological, or immunological; cutaneous, gastrointestinal, or kidney manifestations, as well as in mental health, both as a result of acute infection and by the so-called post-COVID-19 syndrome ( 29 ). In this context, developing effective and safe vaccines was the determining control measure for pandemic management since, in addition to reducing the transmission of infections and allowing the control of the disease, vaccines had a determining role in reducing severe and fatal complications associated with infection ( 30 ). In addition to the above, the time in which the vaccine candidates were available, where it took less than a year for developers to complete the design, manufacturing, efficacy and safety testing and evaluation and approval for use, is an immeasurable scientific and public health learning, as well as an example of cooperation between healthcare authorities, the scientific community and private sector ( 31 ).
This review presents an analysis of the methods, populations, and scope of the studies that have evaluated the efficacy/effectiveness and safety of the vaccines available for COVID-19, emphasizing CoronaVac. Differences were found in terms of the proportion of populations evaluated, follow-up times, and times of the studies regarding the appearance of variants of concern.
Although some clinical trials with random assignment have been carried out to assess efficiency and safety outcomes with CoronaVac, these have limitations in terms of feasibility, follow-up times, and with this, the possibility of evaluating safety outcomes that occur with low frequencies ( 32 ). In this sense, it is important to carry out observational data analysis. However, not all studies have used homogeneous methods of analysis. Both the prevention of infection, and the prevention of outcomes such as hospitalization or death, have been valued through similar outcomes, but some through multivariate analysis of dependencies, and others through analysis that try to infer causally through different control methods of confounding. Studies have compared the evaluation of the same outcome through different methods, including multivariable logistic regression, propensity matching, propensity adjustment, and propensity-base weighting. However, researchers described that the estimates are very sensitive to the explicit or implicit weighting system in an adjustment technique, so it must be clear for which population a global treatment estimate is most appropriate ( 33 ).
It is important to recognize that there are common challenges in the collection, notification, and use of epidemiological data, such as the exhaustiveness and representativeness of the results and their comparability in time, among others. Therefore, it is necessary to identify the strongest analytical designs (among them the interrupted temporal series and comparative longitudinal studies), accompanied by sensitivity analysis of the results and being explicit, starting from the design, in the type of biases and problems that can be found in the data analysis that is available ( 34 ).
Concerning the evaluation of the immune response to the different types of vaccines, it has been oriented both to the antibody-mediated response and that mediated by cellular immunity. Among the antibody-mediated response, the reference standard has been established with the specific neutralizing antibody response against spike proteins of the virus, and a proxy to this response assessing neutralizing capacity has been measured in other studies by immunoglobulin G (IgG) antibody levels against the SARS-CoV-2 receptor binding domain (RBD) ( 35 ).
In the different studies, the decrease in the response levels to specific neutralizing antibodies was assumed to indicate the vaccine protection level when the levels of specific neutralizing antibodies fell between 4 and 6 months. The statistical methods used for their measurement are not homogeneous among all studies which has been used to recommend the application of boosters with vaccines produced in homologous or heterologous platforms of those received in established vaccination schemes ( 36 , 37 ).
To assess the duration of vaccine protection in the real world, it is also important to consider the difficulties in assessing the cellular memory immune response. The measurement of the CD4+ and CD8+ T lymphocytes response expressed in the production of different activation markers is heterogeneous, depending on antigenic stimuli such as peptides from circulating virus variants, cells from infected individuals, or peptides from different vaccines, in addition to diversity in the host response, which does not allow to have precise indicators to define optimal vaccination schedules ( 38 , 39 ).
In this context, inactivated whole virus vaccines, such as CoronaVac, by preserving epitopes of the virus, could respond in a broader spectrum to the different variants of circulating viruses or to new mutations, which could lead to the optimization of global vaccination schedules ( 10 ).
The main strength of our study lies in its systematic development, which reduces the possibility of biases in study selection. The use of different databases, including Latin American ones, allows for a broader search, although it is acknowledged that due to the magnitude of research on this topic, there may still be unreported or unfound studies, behaving as gray literature. The review results enabled us to achieve our objective, which was to describe how the efficacy/effectiveness of COVID-19 vaccines has been evaluated, with emphasis on CoronaVac. This allowed for the identification of some differences in these methods and some persisting gaps in defining more homogeneous methods for evaluation, regardless of whether these studies had high or low certainty in their evidence, which should be revisited if the objective is to evaluate the effectiveness and/or safety in the population of these interventions. However, the findings presented could be assessed and discussed with broader groups of experts in the field, which would help generate more accurate recommendations regarding their significance and potential implications.
In addition to the mentioned limitations, it is important to acknowledge that this type of review, having less precise question definitions compared to systematic reviews of effectiveness and safety (with their PICO structure), may result in some gaps in the application of search terms that could affect the results. Additionally, the vast amount of information, as was the case in our review, can create difficulties in synthesis and analysis, so it is crucial, as mentioned, to continue the discussion in groups with increasingly greater expertise in the subject ( 40 ). Lastly, while it is tempting to provide quantitative results regarding the synthesis conducted, the most important aspect is to address the original question regarding the gaps in the evaluation of these vaccines.
5 Conclusion
Published information on the evaluation of the efficacy/effectiveness and safety of the different vaccines against COVID-19 is abundant. However, there are differences in terms of vaccine application schedules, population definition, outcomes evaluated, follow-up times, and safety assessment, as well as non-standardization in the reporting of results, which may hinder the generalizability of the findings. It is important to define the relevance of the analysis methods in advance, considering these differences and the heterogeneity that can be produced in the analysis and meta-analysis of this information. It is important to generate meetings and consensus strategies for the methods and reporting of this type of studies, which will allow to reduce the heterogeneity in their presentation and a better understanding of the effect of these vaccines.
Data availability statement
The original contributions presented in the study are included in the article/ Supplementary material , further inquiries can be directed to the corresponding author.
Author contributions
JA-Á: Conceptualization, Methodology, Writing – original draft, Writing – review & editing, Investigation. PA-V: Writing – original draft, Writing – review & editing, Investigation. DA-L: Writing – original draft, Writing – review & editing, Investigation. AC-E: Writing – original draft, Writing – review & editing, Investigation. AG-H: Writing – original draft, Writing – review & editing, Investigation. LL-C: Writing – original draft, Writing – review & editing, Investigation. IM: Writing – original draft, Writing – review & editing, Investigation. DO-L: Writing – original draft, Writing – review & editing, Investigation. GQ: Writing – original draft, Writing – review & editing, Investigation. SR-B: Writing – original draft, Writing – review & editing, Investigation. CR-B: Funding acquisition, Writing – original draft, Writing – review & editing, Investigation. PR: Writing – original draft, Writing – review & editing, Investigation. MS-O: Writing – original draft, Writing – review & editing, Investigation. CT-A: Writing – original draft, Writing – review & editing, Investigation. MA-M: Funding acquisition, Project administration, Writing – original draft, Writing – review & editing, Investigation, Project Administration.
The author(s) declare financial support was received for the research, authorship, and/or publication of this article. CDC-China COVEP Fund Project #211-CO-04 (Submission 03/06/2022). The funders had no role in the design and development of the study, collection, management, analysis, interpretation of the data, writing the report, and the decision to submit the manuscript for publication.
Acknowledgments
We would like to thank Doracelly Hincapié-Palacio and María Teresa Rugeles-López, professors and researchers at the University of Antioquia, for their contributions and suggestions in the discussion of the results.
Conflict of interest
The authors declare that the research was conducted in the absence of any commercial or financial relationships that could be construed as a potential conflict of interest.
Publisher’s note
All claims expressed in this article are solely those of the authors and do not necessarily represent those of their affiliated organizations, or those of the publisher, the editors and the reviewers. Any product that may be evaluated in this article, or claim that may be made by its manufacturer, is not guaranteed or endorsed by the publisher.
Supplementary material
The Supplementary material for this article can be found online at: https://www.frontiersin.org/articles/10.3389/fpubh.2024.1321327/full#supplementary-material
1. Sharma, D, and Shalimar,. COVID-19: finally on wane, with reduced lethality. Comb Chem High Throughput Screen . (2022) 25:768–70. doi: 10.2174/1386207324666210811130046
PubMed Abstract | Crossref Full Text | Google Scholar
2. Hodgson, SH, Mansatta, K, Mallett, G, Harris, V, Emary, KRW, and Pollard, AJ. What defines an efficacious COVID-19 vaccine? A review of the challenges assessing the clinical efficacy of vaccines against SARS-CoV-2. Lancet Infect Dis . (2021) 21:e26–35. doi: 10.1016/S1473-3099(20)30773-8
3. Fang, E, Liu, X, Li, M, Zhang, Z, Song, L, Zhu, B, et al. Advances in COVID-19 mRNA vaccine development. Signal Transduct Target Ther . (2022) 7:94. doi: 10.1038/s41392-022-00950-y
4. Doherty, M, Buchy, P, Standaert, B, Giaquinto, C, and Prado-Cohrs, D. Vaccine impact: benefits for human health. Vaccine . (2016) 34:6707–14. doi: 10.1016/j.vaccine.2016.10.025
Crossref Full Text | Google Scholar
5. Conklin, L, Hviid, A, Orenstein, WA, Pollard, AJ, Wharton, M, and Zuber, P. Vaccine safety issues at the turn of the 21st century. BMJ Glob Health . (2021) 6:898. doi: 10.1136/bmjgh-2020-004898
6. Tregoning, JS, Flight, KE, Higham, SL, Wang, Z, and Pierce, BF. Progress of the COVID-19 vaccine effort: viruses, vaccines and variants versus efficacy, effectiveness and escape. Nat Rev Immunol . (2021) 21:626–36. doi: 10.1038/s41577-021-00592-1
7. Williams, LR, Ferguson, NM, Donnelly, CA, and Grassly, NC. Measuring vaccine efficacy against infection and disease in clinical trials: sources and magnitude of bias in coronavirus disease 2019 (COVID-19) vaccine efficacy estimates. Clin infect dis: official publication of the Infect Dis Society of America agosto de . (2022) 75:e764–73. doi: 10.1093/cid/ciab914
8. Fiolet, T, Kherabi, Y, MacDonald, CJ, Ghosn, J, and Peiffer-Smadja, N. Comparing COVID-19 vaccines for their characteristics, efficacy and effectiveness against SARS-CoV-2 and variants of concern: a narrative review. Clin microbiol infect: official publication of the European Society of Clin Microbiol Infect Dis . (2022) 28:202–21. doi: 10.1016/j.cmi.2021.10.005
9. Graña, C, Ghosn, L, Evrenoglou, T, Jarde, A, Minozzi, S, Bergman, H, et al. Efficacy and safety of COVID-19 vaccines. Cochrane Database Syst Rev . (2022) 2023:477. doi: 10.1002/14651858.CD015477
10. Jin, L, Li, Z, Zhang, X, Li, J, and Zhu, F. CoronaVac: a review of efficacy, safety, and immunogenicity of the inactivated vaccine against SARS-CoV-2. Hum Vaccin Immunother . (2022) 18:2096970. doi: 10.1080/21645515.2022.2096970
11. Peters, MDJ, Godfrey, C, McInerney, P, Munn, Z, Tricco, AC, and Khalil, H. Chapter 11: Scoping Reviews (2020 version). E Aromataris and Z Munn, editors. JBI Manual for Evidence Synthesis. JBI; (2020). Available from https://synthesismanual.jbi.global .
Google Scholar
12. Ouzzani, M, Hammady, H, Fedorowicz, Z, and Elmagarmid, A. Rayyan-a web and mobile app for systematic reviews. Systematic Rev . (2016) 5:210. doi: 10.1186/s13643-016-0384-4
13. Boruah, A, Westenberg, E, Hanif Khan, A, Kee Hoo, F, Guekht, A, Spatola, M, et al. Characterization and analysis of neurologic adverse events associated with COVID-19 vaccination. Neurol Int . (2022) 98:784. doi: 10.1212/WNL.98.18_supplement.3784
14. Bueno, SM, Abarca, K, González, PA, Gálvez, NMS, Soto, JA, Duarte, LF, et al. Safety and immunogenicity of an inactivated severe acute respiratory syndrome coronavirus 2 vaccine in a subgroup of healthy adults in Chile. Clin infect dis: official publication of the Infect Dis Society of America . (2022) 75:e792–804. doi: 10.1093/cid/ciab823
15. Duarte, LF, Gálvez, NMS, Iturriaga, C, Melo-González, F, Soto, JA, Schultz, BM, et al. Immune profile and clinical outcome of breakthrough cases after vaccination with an inactivated SARS-CoV-2 vaccine. Front Immunol . (2021) 12:742914. doi: 10.3389/fimmu.2021.742914
16. Tanriover, MD, Doğanay, HL, Akova, M, Güner, HR, Azap, A, Akhan, S, et al. Efficacy and safety of an inactivated whole-virion SARS-CoV-2 vaccine (CoronaVac): interim results of a double-blind, randomised, placebo-controlled, phase 3 trial in Turkey. Lancet (London, England) . (2021) 398:213–22. doi: 10.1016/S0140-6736(21)01429-X
17. Fadlyana, E, Rusmil, K, Tarigan, R, Rahmadi, AR, Prodjosoewojo, S, Sofiatin, Y, et al. A phase III, observer-blind, randomized, placebo-controlled study of the efficacy, safety, and immunogenicity of SARS-CoV-2 inactivated vaccine in healthy adults aged 18-59 years: an interim analysis in Indonesia. Vaccine . (2021) 39:6520–8. doi: 10.1016/j.vaccine.2021.09.052
18. Cheng, FWT, Fan, M, Wong, CKH, Chui, CSL, Lai, FTT, Li, X, et al. The effectiveness and safety of mRNA (BNT162b2) and inactivated (CoronaVac) COVID-19 vaccines among individuals with chronic kidney diseases. Kidney Int . (2022) 102:922–5. doi: 10.1016/j.kint.2022.07.018
19. Can, G, Acar, HC, Aydin, SN, Balkan, II, Karaali, R, Budak, B, et al. Waning effectiveness of CoronaVac in real life: a retrospective cohort study in health care workers. Vaccine . (2022) 40:2574–9. doi: 10.1016/j.vaccine.2022.03.032
20. Jara, A, Undurraga, EA, Zubizarreta, JR, González, C, Pizarro, A, Acevedo, J, et al. Effectiveness of homologous and heterologous booster doses for an inactivated SARS-CoV-2 vaccine: a large-scale prospective cohort study. Lancet Glob Health . (2022) 10:e798–806. doi: 10.1016/S2214-109X(22)00112-7
21. Cerqueira-Silva, T, Andrews, JR, Boaventura, VS, Ranzani, OT, de Araújo, OV, Paixão, ES, et al. Effectiveness of CoronaVac, ChAdOx1 nCoV-19, BNT162b2, and Ad26.COV2.S among individuals with previous SARS-CoV-2 infection in Brazil: a test-negative, case-control study. The lancet. Infect Dis Ther . (2022) 22:791–801. doi: 10.1016/S1473-3099(22)00140-2
22. Cerqueira-Silva, T, Katikireddi, SV, de Araujo, OV, Flores-Ortiz, R, Júnior, JB, Paixão, ES, et al. Vaccine effectiveness of heterologous CoronaVac plus BNT162b2 in Brazil. Nat Med . (2022) 28:838–43. doi: 10.1038/s41591-022-01701-w
23. Ranzani, OT, Hitchings, MDT, de Melo, RL, de França, GVA, de FR, FC, Lind, ML, et al. Effectiveness of an inactivated Covid-19 vaccine with homologous and heterologous boosters against Omicron in Brazil. Nat Commun . (2022) 13:5536. doi: 10.1038/s41467-022-33169-0
24. Sritipsukho, P, Khawcharoenporn, T, Siribumrungwong, B, Damronglerd, P, Suwantarat, N, Satdhabudha, A, et al. Comparing real-life effectiveness of various COVID-19 vaccine regimens during the delta variant-dominant pandemic: a test-negative case-control study. Emerg Microbes Infect . (2022) 11:585–92. doi: 10.1080/22221751.2022.2037398
25. Zeng, G, Wu, Q, Pan, H, Li, M, Yang, J, Wang, L, et al. Immunogenicity and safety of a third dose of CoronaVac, and immune persistence of a two-dose schedule, in healthy adults: interim results from two single-Centre, double-blind, randomised, placebo-controlled phase 2 clinical trials. Lancet Infect Dis . (2022) 22:483–95. doi: 10.1016/S1473-3099(21)00681-2
26. Chantasrisawad, N, Puthanakit, T, Kornsitthikul, K, Jaru-Ampornpan, P, Tawan, M, Matapituk, P, et al. Immunogenicity to SARS-CoV-2 Omicron variant among school-aged children with 2-dose of inactivated SARS-CoV-2 vaccines followed by BNT162b2 booster. Vaccine: X . (2022) 12:100221. doi: 10.1016/j.jvacx.2022.100221
27. Karamese, M, and Tutuncu, EE. The effectiveness of inactivated SARS-CoV-2 vaccine (CoronaVac) on antibody response in participants aged 65 years and older. J Med Virol . (2022) 94:173–7. doi: 10.1002/jmv.27289
28. Demirbakan, H, Koçer, I, Erdoğan, M, and Bayram, A. Assessing humoral immune response after two doses of an inactivated SARS-CoV-2 vaccine (CoronaVac) in healthcare workers. Public Health . (2022) 205:1–5. doi: 10.1016/j.puhe.2022.01.011
29. Peramo-Álvarez, FP, López-Zúñiga, MÁ, and López-Ruz, MÁ. Medical sequels of COVID-19. Med Clin (Barc) . (2021) 157:388–94. doi: 10.1016/j.medcli.2021.04.023
30. Park, JW, Lagniton, PNP, Liu, Y, and Xu, RH. mRNA vaccines for COVID-19: what, why and how. Int J Biol Sci . (2021) 17:1446–60. doi: 10.7150/ijbs.59233
31. Hodgson, J . The pandemic pipeline. Nat Biotechnol . (2020) 38:523–32. doi: 10.1038/d41587-020-00005-z
32. Gilmartin-Thomas, JF, Liew, D, and Hopper, I. Observational studies and their utility for practice. Aust Prescr . (2018) 41:82–5. doi: 10.18773/austprescr.2018.017
33. Kurth, T, Walker, AM, Glynn, RJ, Chan, KA, Gaziano, JM, Berger, K, et al. Results of multivariable logistic regression, propensity matching, propensity adjustment, and propensity-based weighting under conditions of nonuniform effect. Am J Epidemiol . (2006) 163:262–70. doi: 10.1093/aje/kwj047
34. Stoto, MA, Woolverton, A, Kraemer, J, Barlow, P, and Clarke, M. COVID-19 data are messy: analytic methods for rigorous impact analyses with imperfect data. Glob Health . (2022) 18:2. doi: 10.1186/s12992-021-00795-0
35. Lopera, TJ, Chvatal-Medina, M, Flórez-Álvarez, L, Zapata-Cardona, MI, Taborda, NA, Rugeles, MT, et al. Humoral response to BNT162b2 vaccine against SARS-CoV-2 variants decays after six months. Front Immunol . (2022) 13:879036. doi: 10.3389/fimmu.2022.879036
36. Widge, AT, Rouphael, NG, Jackson, LA, Anderson, EJ, Roberts, PC, Makhene, M, et al. Durability of responses after SARS-CoV-2 mRNA-1273 vaccination. N Engl J Med . (2021) 384:80–2. doi: 10.1056/NEJMc2032195
37. Doria-Rose, N, Suthar, MS, Makowski, M, O’Connell, S, McDermott, AB, Flach, B, et al. Antibody persistence through 6 months after the second dose of mRNA-1273 vaccine for Covid-19. N Engl J Med . (2021) 384:2259–61. doi: 10.1056/NEJMc2103916
38. Primorac, D, Vrdoljak, K, Brlek, P, Pavelić, E, Molnar, V, Matišić, V, et al. Adaptive immune responses and immunity to SARS-CoV-2. Front Immunol . (2022) 13:848582. doi: 10.3389/fimmu.2022.848582
39. Benjamanukul, S, Traiyan, S, Yorsaeng, R, Vichaiwattana, P, Sudhinaraset, N, Wanlapakorn, N, et al. Safety and immunogenicity of inactivated COVID-19 vaccine in health care workers. J Med Virol . (2022) 94:1442–9. doi: 10.1002/jmv.27458
40. Mak, S, and Thomas, A. An introduction to scoping reviews. J Grad Med Educ . (2022) 14:561–4. doi: 10.4300/JGME-D-22-00620.1
Keywords: COVID-19, SARS-CoV-2, vaccines, CoronaVac, review
Citation: Alzate-Ángel JC, Avilés-Vergara PA, Arango-Londoño D, Concha-Eastman A, Garcés-Hurtado A, López-Carvajal L, Minotta IL, Ortega-Lenis D, Quintero G, Reina-Bolaños S, Reina-Bolaños CA, Roa P, Sánchez-Orozco M, Tovar-Acero C and Arbeláez-Montoya MP (2024) How has research on the effectiveness and safety of COVID-19 vaccination been evaluated: a scope review with emphasis on CoronaVac. Front. Public Health . 12:1321327. doi: 10.3389/fpubh.2024.1321327
Received: 13 October 2023; Accepted: 25 March 2024; Published: 10 April 2024.
Reviewed by:
Copyright © 2024 Alzate-Ángel, Avilés-Vergara, Arango-Londoño, Concha-Eastman, Garcés-Hurtado, López-Carvajal, Minotta, Ortega-Lenis, Quintero, Reina-Bolaños, Reina-Bolaños, Roa, Sánchez-Orozco, Tovar-Acero and Arbeláez-Montoya. This is an open-access article distributed under the terms of the Creative Commons Attribution License (CC BY) . The use, distribution or reproduction in other forums is permitted, provided the original author(s) and the copyright owner(s) are credited and that the original publication in this journal is cited, in accordance with accepted academic practice. No use, distribution or reproduction is permitted which does not comply with these terms.
*Correspondence: Juan C. Alzate-Ángel, [email protected]
Disclaimer: All claims expressed in this article are solely those of the authors and do not necessarily represent those of their affiliated organizations, or those of the publisher, the editors and the reviewers. Any product that may be evaluated in this article or claim that may be made by its manufacturer is not guaranteed or endorsed by the publisher.
Thesis Helpers

Find the best tips and advice to improve your writing. Or, have a top expert write your paper.
170 Vaccination Research Paper Topics For Stellar Students
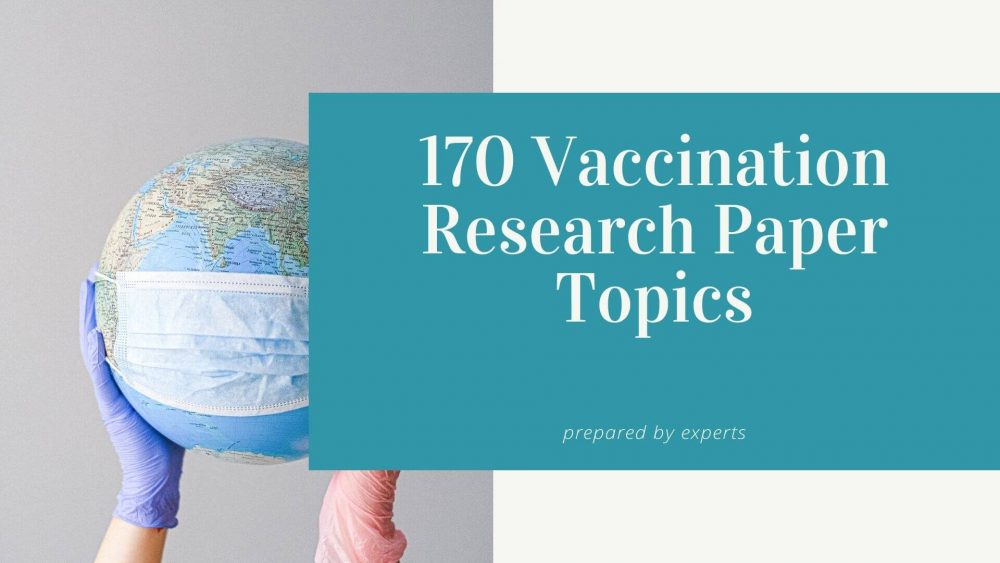
Research papers are a monumental highlight in your academic journey. They are a critical milestone in your studies that must be tackled with the utmost care and stellar diligence. Vaccination topics are susceptible as you have to show complete mastery of all details.
If you are pursuing a medicine course, then vaccination research topics might be an excellent area of interest. A good research paper starts with a great topic, and we are here to help you nail that. We understand the significance of research papers, and that is why we have handpicked 170 out-of-the-box vaccination research paper topics, titles, and ideas to make your work seamless.
Debate Topics About Vaccination
- What is reverse vaccinology?
- Look at the ways of harnessing the participation of dendritic cells in tolerance and immunity
- What are some of the approaches to advance cancer vaccines to clinical utility?
- Highlight innovative therapeutic and vaccine approaches against respiratory pathogens
- Examine immunity to malaria and vaccine strategies
- Assess molecular vaccines against pathogens in the post-genomic era
- Comprehending the limitations of today’s influenza vaccine strategies and further development of more efficient therapeutic and preventative interventions
- Study HIV-associated persistent inflammation and immune activation
- Analyze recent advances in respiratory virus infection
- What is the novel approach for anti-tumor vaccines
- Unravel the challenges and progress in the development of a B cell-based hepatitis C virus vaccine
- What is the functional relevance of Tatraspanins in the immune system?
- Look at advanced immunization technologies for next-generation vaccines
- Evaluate epitope discovery and synthetic vaccine design
- In what ways can tuberculosis be treated by targeting host immunity
- What are the immunomodulatory effects of drugs in the treatment of immune-related diseases
- Highlight natural antibodies in health and disease
- Discuss different influenza virus vaccines and immunotherapy
- What are some of the shadows of cancer immunotherapy
- Understanding the therapeutical potential of extracellular vesicles
- A review of the ethical theories and problems associated with vaccination in America
- Do vaccines love the Darwinian fitness of immune cells
Vaccination Behavior Research Topics
- Unraveling demand and supply effects on the up-take of influenza vaccinations
- Point out new approaches to the seasonal flu vaccine
- Exploring the impact of vaccination
- Investigating patient experience with, and the use of, an electronic monitoring system to assess vaccination responses
- A meta-analysis of interventions that enhance the use of adult immunization and cancer screening services
- Do vaccines seem to work against bacterial and viral infections, and are they effective?
- Gathering the evidence for the introduction of typhoid vaccine: worldwide vaccine testing
- Explore molecular mimicry to broaden the immune response to carbohydrate antigens for vaccine development
- Tumor-associated glycan and immune surveillance
- Rational design and application of idiotope vaccines
- Assessing the effects of vaccines on immune-deficient people
- What are the impacts of rapid growth and deployment of high-volume vaccines for pandemic response
Anti-vaccination Research Paper Topics
- Should the state impose vaccinations, or should the choice be left up to the child’s parents?
- What is the connection between vaccination and autism?
- Is natural immunity better than immunity through immunization?
- Examining cultural perspectives on vaccination
- Are they worth it? adverse effects of vaccination on children
- To vaccinate or not against HPV? A content analysis of vocabularies of motives
- Vaccines: religious and cultural arguments from an Islamic perspective
- Anti-science populism or biomedicine’s unresolved knots? Comparing views on the movements against mandatory pediatric vaccines
- An anthropological commentary on vaccine hesitancy, decision-making, and interventionism among religious minorities
- Understanding attitudes to vaccination
Research Topics For Covid-19 Vaccination
- Medical mistrust in the context of Covid-19: implications for intended care-seeking and quarantine policy support in the United States
- What is the acceptability of the potential COVID-19 vaccine among smokers and non-smokers?
- COVID-19 vaccine hesitancy in healthcare personnel: are there any differences among classifications
- Discuss various options that one can use to convince people to get the covid-19 vaccine
- Examining COVID-19 vaccine efficacy after the first dose: Pfizer, Moderna, AstraZeneca
- Discuss the impacts of herd immunity during the covid-19 pandemic
- What are some of the effects of covid-19 vaccination on transmission of disease?
- Discuss whether antibodies generated through vaccination recognize all-new variants of covid-19
- Investigate how the intensity of lockdowns accelerate or influence mutation of the COVID virus
- Examine how the new covid-19 strain identified in England will affect the available vaccines.
- Outline which immunoglobulin types can be used as the markers for covid-19 vaccination
- Which is the best way to deal with swaps after completing vaccinations in nursing homes
- How do we curb vaccine hesitancy among healthcare providers?
- Which one is the more dangerous, covid-19 or covid-19 vaccine? What must be the individual decision?
- Analyzing Ebola and the evolving ethics of quarantine
- Break down some of the side effects of covid-19 vaccination
- How long will immunity last after receiving the covid-19 vaccination?
- Will, a covid-19 vaccine work for everyone? Are there people who cannot get vaccinated?
- Is bivalent OPV immunization capable of mitigating the impact of covid-19?
- What are the expected long-term side effects of the vaccination for covid-19?
- Evaluate differences between the first and second doses of the covid-19 mRNA vaccine?
- Examine the ingredients in the covid-19 mRNA vaccine
- Can a person’s DNA change through mRNA vaccines?
- Factors that stops the body from continuing to produce COVID-19 spike protein after getting a COVID-19 mRNA
- Discuss whether a person vaccinated against covid-19 will be able to spread the virus to susceptible people
- Investigating vaccination adverse outcomes and costs of vaccine injury claims(VICs): In the past, present, and during COVID-19.
- Who gets cured: Covid-19 and the development of critical sociology and anthropology of cure
- Development of perception and attitude scales related to COVID-19 pandemic
- Does the mutation of the coronavirus affect the capacity of the vaccines to prevent disease?
- A case-control study: finding a link between pre-existing antibodies got after the childhood vaccinations or past infections and COVID-19?
- Queue questions: ethics of COVID-19 vaccine prioritization
- Disparities between Black and White in H1N1 vaccination among adults in the U.S. in 2009: A cautionary tale for the COVID-19 pandemic
- Autonomy and refusal in pandemics: What to do with those who refuse COVID-19 vaccines
- Knowledge, attitude, and acceptance of a COVID-19 vaccine: a global cross-sectional study
- Prospects of COVID-19 vaccine implementation in the U.S.: Challenges and potential solutions
- What are the effects of COVID-19 vaccines on pregnant women?
- Compare and contrast the efficacy of different covid-19 vaccines.
- Ways to improve covid-19 vaccine acceptance
- Determination of causation between COVID-19 vaccines and potential adverse effects
Vaccination Of Children Topics
- What is the essence of increasing HPV vaccination among children?
- Analyze the primary diseases that vaccines prevent in children
- What will happen if a child’s vaccination schedule is delayed
- Look at the vaccination schedule for children in the U.S.
- Can children receive more than one vaccine at a time?
- Examine revaccination outcomes of children with proximate vaccine seizures
- What are the impacts of measles-containing vaccination in children with the severe underlying neurologic disease?
- Evaluate the challenges involved in measuring immunization activity coverage among measles zero-dose children
- What is the connection between the polio vaccine and the risk of cancer among children?
- Do multiple vaccines affect babies’ health and immune system in an adverse war, or can their bodies handle them?
- What are the various vaccination options available for children, and are they harmful to children’s overall health?
- The case for further research and development: assessing the potential cost-effectiveness of microneedle patches in childhood measles vaccination programs
- Evaluate the accuracy of parental recall of child immunization in an inner-city population
- Evaluating maternal acculturation and childhood immunization levels among children in African-American families in Florida
- Policy analysis: the impact of the vaccine for children’s program on child immunization delivery
- The effect of managed care: investigating access of infant immunizations for poor inner-city families
- Who takes up free flu shots? Investigating the effects of an expansion in coverage
- What are the societal and parental values for the risks and benefits of childhood combination vaccines?
- Looking into trends in vaccination intentions and risk perceptions: a longitudinal study of the first year of the H1N1 pandemic
Healthcare Topics About Vaccination
- Conscious consideration of herd immunity in influenza vaccination decisions
- A case study of ethnic or racial differences in Medicare experiences and immunization
- What preservatives are used in vaccines
- Discuss the relationship between vaccines and autism
- What is the role of epidemiology in infection control?
- How t design and select the most relevant immunogenic peptide sequences
- Discuss why the Zika virus has not had a significant impact in Africa as compared to America
- What are the advantages of using the phage display technology of antibodies versus hybridism technology?
- Analyzing the impact and cost-effectiveness of vaccination programs in a country using mathematical models
- Malaria vaccines: progress and problems
- Malaria: cloning genes for antigens of plasmodium falciparum
- Fighting profits on the pandemic: The fight for vaccines in today’s economic and geopolitical context
- Molecular and biotechnological approaches to fish vaccines
- Immunogenicity of a whole-cell pertussis vaccine with low lipopolysaccharide content in infants
- Immunogrid: an integrative environment for large-scale simulation of the immune system for vaccine discovery, design, and optimization
Thesis Topics In Vaccination
- Investigating challenges and opportunities in vaccine delivery, discovery, and development
- Discuss classic methods of vaccine development
- What are some of the current problems in vaccinology?
- Assess some of the latest tools for vaccine development
- Using cost-effectiveness analysis to support research and development portfolio prioritization for product innovations in measles vaccination
- Communicating vaccine safety during the introduction and development of vaccines
- Highlighting viral vectors for use in the development of biodefense vaccines
- What is the role of US. military research programs in the invention of USA-approved vaccines for naturally occurring infectious diseases
- Curbing outbreaks: utilizing international governmental risk pools to fund research and development of infectious disease medicines and vaccines
- Vaccine stabilization: research commercialization and likely impacts
- Exam the unequal interactions of the role of patient-centered care in the inequitable diffusion of medical innovation, the human papillomavirus(HPV) vaccine
- A case study of the status of development of vaccines and vaccine research for malaria
- Enteric infections vs vaccines: a public health and clinical research agenda for developing countries
- A review of research and vaccine development for industry animals in third world countries
- How the research-based industry approaches vaccine development and establishes priorities
- A look at the status of vaccine research and development of a vaccine for HIV-1
- Modeling a cost-effective vaccination strategy for the prevention of herpes zoster infection
- Using an adequate T.B. vaccination regiment to identify immune responses associated with protection in the murine model
- A systematic analysis of the link between vaccines and atopic dermatitis
- Do vaccines provide better immunity than natural infections?
- Is there a need to be vaccinated against a disease that is not available in your country or community
- How to strengthen adult immunization via coordinated action
- Using the general equilibrium method to assess the value of a malaria vaccine: An application to African countries
- Who should take up free flu shots?
- Evaluate the impact of vaccination among health care personnel
- Retail clinics and their impact on vaccination in the U.S.
- Discuss the societal values for the benefits and risks of childhood combination vaccines
- How safe and effective is the synovial vaccine for people above 60 years
- Evaluating vaccination effectiveness of group-specific fractional-dose strategies
Law Research Topics On Vaccination
- Explain why there are age restrictions for Rotavirus vaccination?
- Vaccination or hygiene : Which factor contributes to the decline of infectious diseases?
- Outline the main factors that cause vaccine failure
- Discuss why HIV is so hard to vaccinate in uninfected people?
- In what ways do maternal vaccinations affect the fetal nervous system development
- How to deliver malaria vaccine effectively and efficiently
- Highlight the vaccines that are specifically licensed in the U.S. for pregnant women
- How does an immune genetic algorithm work?
- Evaluate the relationship between the success of artificial insemination and vaccination
- Outline the reasons why vaccines underperform in low-income countries
- Discuss U.S. immigration and vaccination policy
- Assessing the effectiveness of compelled vaccination
Vaccination Ethical Topics
- What are the requirements for a strain to be used as a vaccine?
- What is the best way to administer vaccines in children?
- Assessing the benefits of maternal vaccination on breastfed infants
- Evaluating the pros and cons of intraperitoneal vaccination
- Examine ways to measure the pattern of vaccination acceptance
- Investigate Covid-19 transmission, vaccination rate, and the fate of resistant strains
- Look into dark web marketplaces and covid-19 vaccines.
- A close look at covid-19 vaccines and kidney diseases
- Contextualizing the impact of covid-19 vaccine misinformation on vaccination intent in the U.S.
- Examining behaviors and attitudes of medical students towards covid-19 vaccines
From the exceptional vaccination debate topics, titles, and other ideas above, you can quickly tell that we have your best interest at heart. We believe that all students deserve decent scores on their papers. As a result, we are more than willing to recommend affordable online help with research papers from the best-rated sites.
Whether you are overwhelmed by other assignments, dealing with a tight deadline, or lack the spark to pull it off, you can count on cheap yet professional thesis help from trusted assignment help sites. They are ready to deliver high-quality papers, and you can confirm that from any sample or example on the platforms.

Make PhD experience your own
Leave a Reply Cancel reply
Your email address will not be published. Required fields are marked *
Evidence Review of the Adverse Effects of COVID-19 Vaccination and Intramuscular Vaccine Administration
Vaccines are a public health success story, as they have prevented or lessened the effects of many infectious diseases. To address concerns around potential vaccine injuries, the Health Resources and Services Administration (HRSA) administers the Vaccine Injury Compensation Program (VICP) and the Countermeasures Injury Compensation Program (CICP), which provide compensation to those who assert that they were injured by routine vaccines or medical countermeasures, respectively. The National Academies of Sciences, Engineering, and Medicine have contributed to the scientific basis for VICP compensation decisions for decades.
HRSA asked the National Academies to convene an expert committee to review the epidemiological, clinical, and biological evidence about the relationship between COVID-19 vaccines and specific adverse events, as well as intramuscular administration of vaccines and shoulder injuries. This report outlines the committee findings and conclusions.
Read Full Description
- Digital Resource: Evidence Review of the Adverse Effects of COVID-19 Vaccination
- Digital Resource: Evidence Review of Shoulder Injuries from Intramuscular Administration of Vaccines
- Press Release
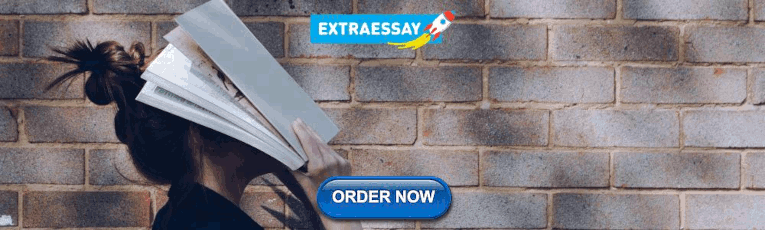
Recent News
NAS Launches Science and Innovation Fund for Ukraine
Science Academies Issue Statements to Inform G7 Talks
Supporting Family Caregivers in STEMM
A Vision for High-Quality Preschool for All
- Load More...
Numbers, Facts and Trends Shaping Your World
Read our research on:
Full Topic List
Regions & Countries
- Publications
- Our Methods
- Short Reads
- Tools & Resources
Read Our Research On:
States have mandated vaccinations since long before COVID-19
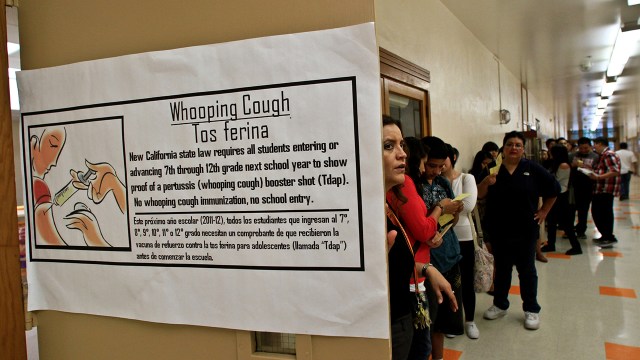
Many Republican governors reacted furiously after President Joe Biden said he would require employees at large businesses to either get vaccinated against COVID-19 or submit to weekly testing. But Republican- and Democratic-led states alike already require hundreds of thousands of their citizens – infants, toddlers and schoolchildren, mostly – to be vaccinated against a panoply of diseases. In fact, mandatory childhood immunizations have been a feature of American society since the 19th century.
Currently, the Centers for Disease Control and Prevention’s Advisory Committee on Immunization Practices recommends routine vaccination against 16 diseases from birth through age 18. The CDC recommendations, in turn, inform individual states’ vaccine mandates: Typically, children who haven’t received the required shots for their age can’t attend school (public, private or parochial) or enroll in child care programs, though there are exemptions for religious, medical or other reasons .
President Biden’s new COVID-19 vaccine mandate ignited a political controversy. At the time, several news accounts pointed out that vaccine mandates were common at the state level, including in Republican-governed states. We wanted to know just how common such mandates were and how they differed from state to state.
To find out, we searched state statutes and rules governing immunizations required for entry to schools and child care centers, by far the most widespread state vaccine mandates. We also consulted the websites of each state’s health department and, when details were still unclear, news reports and other non-official sources.
Our information about recommended vaccines for infants, children and teens came directly from the Centers for Disease Control and Prevention. For the history of vaccine development, approval and recommendations, we consulted the CDC and the Food and Drug Administration , as well as histories maintained by The College of Physicians of Philadelphia and the Children’s Hospital of Philadelphia .
Under Biden’s plan, all companies with more than 100 workers will have to either require their employees be immunized or undergo weekly testing. Biden also acted to mandate shots for federal contractors and most federal workers , and expanded a previously announced vaccine mandate for nursing-home workers to cover virtually all health care workers .
Some states already mandate certain vaccinations for specific categories of adults. New York , for example, requires that all workers in hospitals, nursing homes and other health care facilities be immunized against measles and rubella. Rhode Island requires child care workers to not only be immunized against several common childhood diseases, but to get an annual flu shot, too. Several states have specific vaccination mandates for college students.
But in the main, most vaccine mandates apply to children and teens. We studied state laws, regulations and information from state health departments to assess how widely mandated the CDC’s vaccine recommendations are.
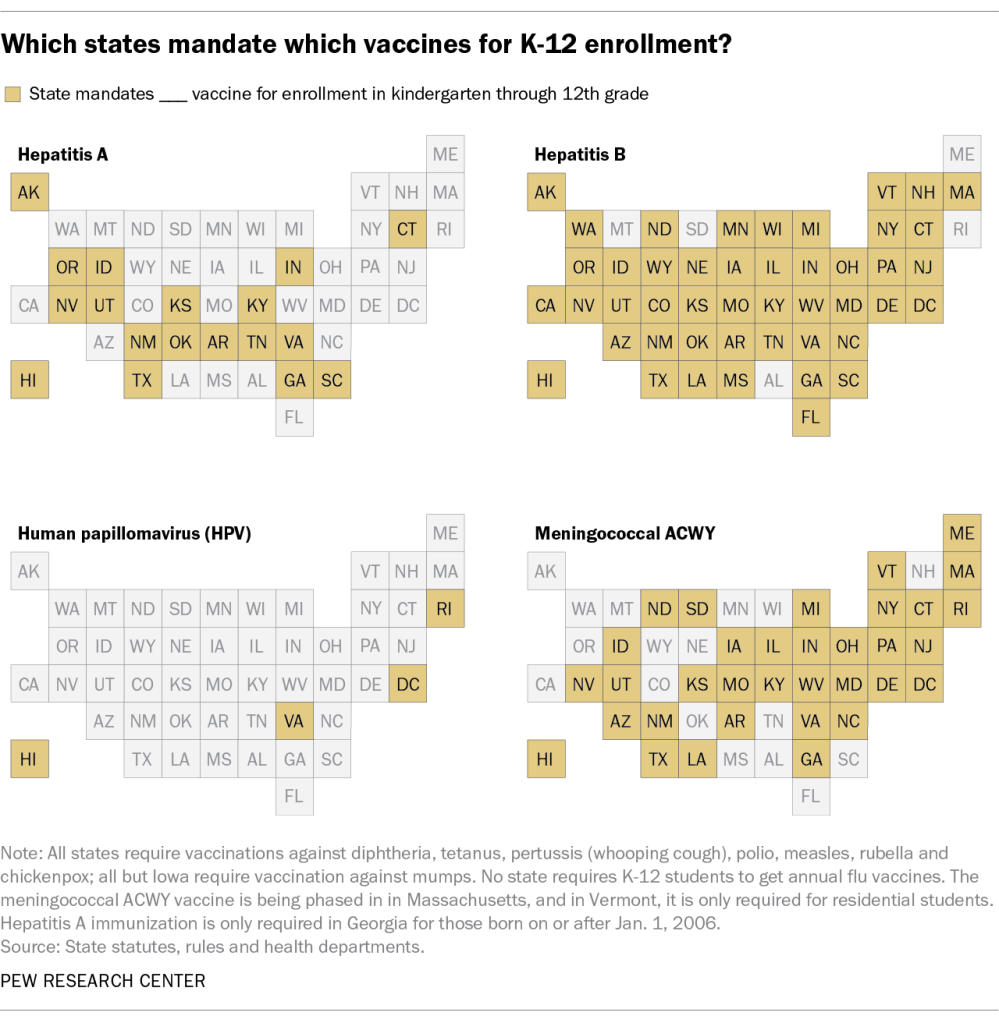
Of the 16 immunizations the CDC recommends for children and teens, all 50 states (plus the District of Columbia) mandate diphtheria, tetanus, pertussis (whooping cough), polio, measles, rubella and chickenpox. In addition, every state except Iowa mandates immunization against mumps. (The diphtheria, tetanus and pertussis vaccines usually are given as a single combined shot, as are the measles, mumps and rubella vaccines.) Except for the chickenpox vaccine, which became available in the United States in 1995, all those vaccines have been around for 50 years or more.
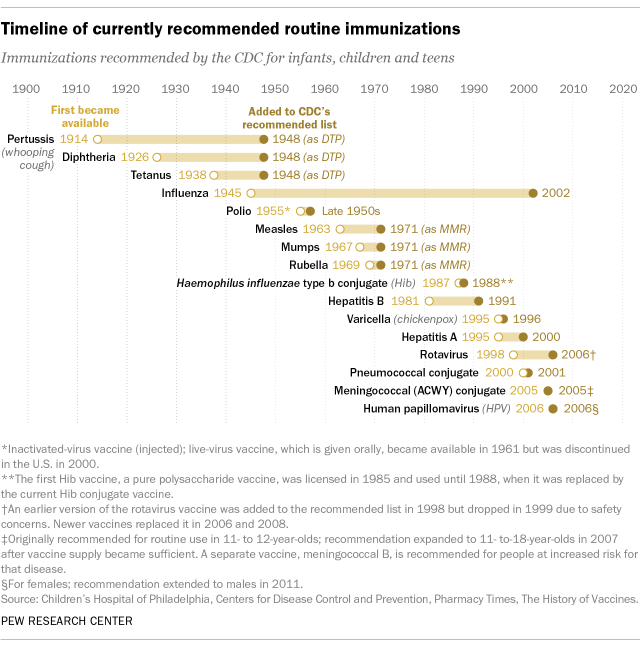
Among newer childhood vaccines, though, state mandates are something of a mixed bag. Only two states (Alabama and South Dakota) don’t require vaccination against hepatitis B at some point in a child’s life, but about half (24) don’t require it for hepatitis A. Just six states – five of them in the Northeast – require annual flu vaccines for child care or preschool enrollment, and none do so for K-12 students.
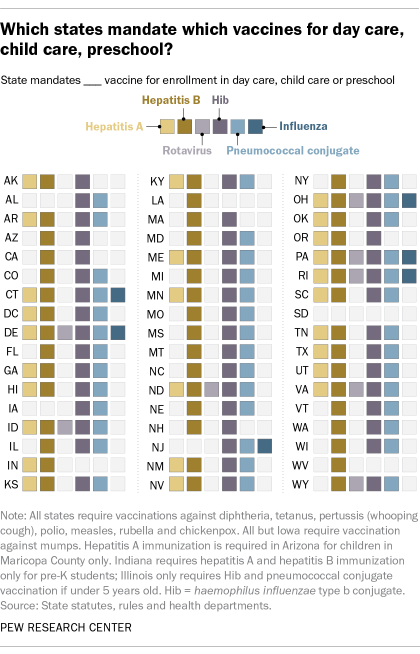
Three vaccines – against rotavirus, pneumococcal disease and Haemophilus influenzae type b, or Hib – typically are recommended for children younger than 5 years old. (Hib, despite what its name might imply, doesn’t cause the flu, but it can cause a range of other ailments , from mild ear infections to potentially deadly meningitis and blood infections.) However, while all but four states mandate the Hib vaccine for day care or pre-K, 10 don’t require the pneumococcal shot, and only eight require immunization against rotavirus.
The remaining two vaccines, against human papillomavirus (HPV) and meningococcal disease , are recommended for teens and older children around age 11 or 12. A majority of states (33, as well as D.C.) require the meningococcal vaccine, although Massachusetts is phasing in its requirement and Vermont only requires it for students living on campus. But only D.C., Hawaii, Rhode Island and Virginia require the HPV shot , which protects against cervical and other cancers but has been controversial because HPV is transmitted sexually.
Vaccination mandates in the U.S. date back to the 19th century, when many cities and states started requiring children to be immunized against smallpox; the Supreme Court upheld such mandates in a landmark 1905 decision . A combination vaccine against diphtheria, tetanus and pertussis became available in 1948, and it was quickly added as a routinely recommended shot.
In 1977, the World Health Organization’s Expanded Program on Immunization set a goal of giving every child in the world access to immunization against six diseases by 1990: dipththeria, pertussis, tetanus, polio, measles and tuberculosis, or TB. (Infants and small children are commonly vaccinated against TB in countries where the disease is prevalent. In the U.S., however, only children who are specifically at risk for contracting TB – or adults in high-exposure settings, such as health care workers – are offered the shot.)
- Coronavirus (COVID-19)
- COVID-19 & Science
- Health Policy
- State & Local Government
How Americans View the Coronavirus, COVID-19 Vaccines Amid Declining Levels of Concern
Online religious services appeal to many americans, but going in person remains more popular, about a third of u.s. workers who can work from home now do so all the time, how the pandemic has affected attendance at u.s. religious services, mental health and the pandemic: what u.s. surveys have found, most popular.
1615 L St. NW, Suite 800 Washington, DC 20036 USA (+1) 202-419-4300 | Main (+1) 202-857-8562 | Fax (+1) 202-419-4372 | Media Inquiries
Research Topics
- Age & Generations
- Economy & Work
- Family & Relationships
- Gender & LGBTQ
- Immigration & Migration
- International Affairs
- Internet & Technology
- Methodological Research
- News Habits & Media
- Non-U.S. Governments
- Other Topics
- Politics & Policy
- Race & Ethnicity
- Email Newsletters
ABOUT PEW RESEARCH CENTER Pew Research Center is a nonpartisan fact tank that informs the public about the issues, attitudes and trends shaping the world. It conducts public opinion polling, demographic research, media content analysis and other empirical social science research. Pew Research Center does not take policy positions. It is a subsidiary of The Pew Charitable Trusts .
Copyright 2024 Pew Research Center
Terms & Conditions
Privacy Policy
Cookie Settings
Reprints, Permissions & Use Policy

An official website of the United States government
The .gov means it’s official. Federal government websites often end in .gov or .mil. Before sharing sensitive information, make sure you’re on a federal government site.
The site is secure. The https:// ensures that you are connecting to the official website and that any information you provide is encrypted and transmitted securely.
- Publications
- Account settings
Preview improvements coming to the PMC website in October 2024. Learn More or Try it out now .
- Advanced Search
- Journal List
- Wiley - PMC COVID-19 Collection

COVID‐19 vaccine research and development: ethical issues
1 Department of Microbiology, Faculty of Medicine Public Health and Nursing, Universitas Gadjah Mada, Yogyakarta Indonesia
2 Medical and Health Research Ethics Committee, Faculty of Medicine Public Health and Nursing, Universitas Gadjah Mada / Dr. Sardjito General Hospital, Yogyakarta Indonesia
The achievements of vaccine research and development bring a hope to our societies that we may cope with the COVID‐19 pandemic. There are two aspects that should be maintained in balance: the immediate necessity for speed of vaccine research and the inherent need for protection of research subjects, which is the foremost concern of research ethics. This narrative review highlights ethical issues in COVID‐19 vaccine research and development that every stakeholder needs to be aware of and to consider.
Introduction
COVID‐19 is a deadly disease which continues to affect many countries in the world. The incidence is higher in the Americas (14 117 714 cases and 486 843 deaths) and Europe (4 515 514 cases and 222 624 deaths) than in South East Asia (4 786 594 cases and 84 541 deaths), Africa (1 088 093 cases and 23 101 deaths) and the Western Pacific (520 012 cases and 11 306 deaths) [ 1 ].
Vaccines are the most important public health measure to protect people from COVID‐19 worldwide, since SARS‐CoV‐2 is highly contagious and infects populations widely and globally [ 2 ]. Traditionally, vaccine development takes years, even decades: from about 40 years for polio to 5 years for Ebola,most vaccines took 15 years on average [ 3 , 4 ]. The trial process for vaccines consists of several steps which need to be conducted systematically and in a measurable stride. The length of this process is correlated with the nature of the vaccine itself, which is to protect healthy people from being infected by pathogens. Adverse events and deleterious effects will not be tolerated, vaccines are not the same as drugs that are consumed by the sick. The risk–benefit analysis for prescription drugs and vaccine administration is different.
The invention of a successful and widely available COVID‐19 vaccine will be a great leap forward for humankind, but there are several challenges to overcome: (1) a lack of understanding of the pathogenesis and the predictive role of vaccines in the clinical pathway of persons being infected by SARS‐CoV‐2 [ 5 , 6 , 7 ], (2) a huge disagreement among experts about how to determine the most immunogenic epitopes and antigens of SARS‐CoV‐2 [ 8 , 9 ], (3) the finding that antibody‐dependent enhancement (ADE) may contribute to the exaggeration of SARS‐CoV‐2 disease [ 10 , 11 ], (4) the lack of established animal models for COVID‐19 vaccine challenge testing, which raises the speculation of using controlled human infection (CHI) as a potential approach [ 3 ], and finally, (5) speculation that the duration of protection by immune response in natural infection is not long enough [ 12 ].
The race for COVID‐19 vaccine invention and development against the spread and catastrophic effects of the disease is real. WHO released a draft list of COVID‐19 candidate vaccines on 3 September 2020. At least 34 vaccine candidates are in clinical evaluation to date [ 13 ]. Several new technologies are used as COVID‐19 vaccine development platforms. Conventional techniques for the development of vaccines such as inactivated, inactivated with adjuvant and live attenuated are still being used. However, reversed vaccinology approaches are also being emplyed, such as a recombinant subunit vaccine, and a more advanced approach using vector delivery systems, along with RNA‐ and DNA‐based vaccines (Table 1 ) [ 4 , 9 , 13 ].
Candidate COVID‐19 Vaccines in Clinical Trial Phases*
The attempts to accelerate vaccine development are associated with efforts to streamline the process. Unfortunately, streamlining may have consequences for the traditional ethics of vaccine research and development, especially the long‐held principles of beneficence and non‐maleficence. This short narrative review summarises the ethical issues that may emerge from the current directions in COVID‐19 vaccine research and development during the pandemic.
Vaccine candidates must fulfil several requirements: safety, efficacy and quality. Because of the current escalation of the global COVID‐19 pandemic, some aspects may change. The speed of vaccine development may push public health ministers, heads of states and the pharmaceutical industry to change their strategy for bulk budget investment for vaccine research. They must decide to prepare mass production events based on the limited data of promising vaccine candidates [ 14 ]. The need to protect billions of earth’s inhabitants pushes governments and societies of the world to a ‘great expectation’ for the new vaccine. The overriding expectation, although with diverse interests, may influence the objective judgement typically required of candidate vaccine safety. Protecting human lives should be the priority.
mRNA‐ [ 15 ] and DNA‐based vaccine technologies [ 9 , 16 ] are being implemented in humans, especially as vaccine candidates. Several concerns about mRNA vaccine safety have been identified besides its promising potential advantages. The most important risks include the possibility that mRNA vaccines may generate strong type I interferon responses that could lead to inflammation and autoimmune conditions [ 17 ]. The safety concerns of DNA‐based vaccines involve the possibility that the targeting of DNA into the chromosomal DNA of the acceptor will trigger mutagenic effects in the functional gene located in the insertion loci [ 18 ]. At present, there are no mRNA‐ and DNA‐based vaccines against any disease authorised to be marketed.
The strategy of DNA vaccines is similar to gene therapy in that a delivery system, such as plasmid, delivers targeted DNA into cells, where it is translated into proteins that induce the acceptors’ immune response to generate targeted T‐cell and antibody responses [ 19 ]. We have experience in using DNA for several gene therapies mostly related to inherited diseases or familial predispositions. Mainstream gene therapy scientists have stated that gene therapy is only suitable for terminally ill patients because the risks are very high [ 20 ]. Vaccine administration is completely different from interventions with gene therapy since the vaccine is for healthy human subjects, and the risk–benefit consideration would be completely different too. Both terminally ill and healthy persons have the same risk for the introduction of foreign DNA into their body, but terminally ill persons may benefit through having a chance to recover from their deadly disease, whereas healthy individuals may not have any benefit because they have never encountered the particular pathogen.
When we perform the risk assessment of new technology, it is based on a theoretical framework without direct evidence concerning to what extent the probability of the risk may occur. Theoretically, DNA vaccine may be able to induce autoimmune diseases and can be inserted into any part of the chromosomes [ 21 ]. Scientists know how the mechanism works and are able to predict the risk if it might happen. But nobody knows for certain how great the probability is of producing mutagenic and deleterious effects in one part of a gene sequence when inserted into another. For example, when a test subject named Jessie Gelsinger was injected with adeno‐associated viruses (AAVs), nobody expected the deadly risk that ultimately occurred in this research subject [ 22 ]. Accordingly, the risk–benefit assessment in the use of new technology should be done carefully. It is true that sometimes we have to deal with a risk possibility that is not immediately present but theoretically possible, and vice versa. Mitigation to the deleterious effect could be started prior to the clinical trial. However, there is always the possible existence of risks that have not been identified yet and will only show in the later phases of clinical trials.
In the current pandemic, all societies expect a breakthrough in medical and health technology. In a situation where understanding of the new disease is poor and no satisfactory medical technology is available for prevention and treatment yet, it is natural to think that ‘doing something is better than nothing’. This is going to make safety judgement among stakeholders more prone to deterioration.
Controlled human infection (CHI)
One of the crucial steps of vaccine development is the challenge test, which is used to measure the potential protection of the candidate. The challenge test is usually part of the pre‐clinical study in an animal model. However, in the case of COVID‐19 and some other diseases, an animal model is not available, although there are candidates that need to be verified [ 3 , 23 , 24 , 25 ]. It seems the pathogen does not produce a similar clinical course in common animal models, which excludes safety and efficacy data from animal models alone. There was a proposal of human challenge testing to replace the pre‐clinical challenge test in animal models, with the use of controlled human infection (CHI). It will solve the problem of the animal models’ unreliability and gain time for the developers especially in phase III [ 3 , 26 ].
To some extent, it is possible to perform these challenge tests with human volunteers. It sounds like an unsafe experimentation, but the choices are extremely limited. The next question is how can we do this experiment with the current ethical review process? The WHO has issued a guideline for CHI [ 27 ]. The guideline is broad and needs local ethics committee approval for its implementation. Considerations of the pros and cons of CHI are widely discussed in COVID‐19 vaccine development. Previously, CHI was used to develop vaccines against malaria [ 28 ], typhoid [ 29 ] and cholera [ 30 ], which are diseases with established treatment [ 31 ]. Subjects who suffered from deleterious effects after experimentation could be rescued by the established treatment. Application of CHI in COVID‐19 is a very different story because there is no standard treatment for this new and highly contagious disease. Nevertheless, there have been thousands of volunteers from 162 countries who declared their willingness to be participants in this CHI [ 32 ]. The need for a vaccine is prevalent in people’s minds and equally necessary from the public health point of view. Without any precedents, it is going to be difficult to judge the risks benefits in this matter [ 33 ].
Controlled human infection could be done in a situation where there is an attenuated virus strain available, for example, using an artificial mutant virus. This approach is to prevent fatal outcomes in trial subjects. But the challenge test results from attenuated virus may not be generalisable – the attenuated strain may not be similar enough to the naturally circulating virus. In addition, producing the attenuated virus may require another step that will take almost as much time to perform as the regular phase III in typical controlled clinical trials. This additional step in an already complicated process will render futile the main purpose to gain more time to develop an effective vaccine [ 34 ].
Location and population
Development sites of COVID‐19 vaccines are involving research subjects from many countries, for example USA, Russia, Argentina, Brazil, Germany, India, Saudi Arabia, Pakistan and others [ 35 ]. The need of multi‐centred research is obvious in the vaccine development. The safety, tolerability, and efficacy of the vaccines should be obtained from different geographic areas, ethnicities, prevalence and varieties of the virus circulating in the areas [ 36 ]. The attempt to fulfil this requirement may result in the involvement of countries with limited resources and whose underdeveloped infrastructure would make the people involved become even more vulnerable as research subjects from the ethical and humane point of view. The possible exploitation of vulnerable people from less developed countries should be reviewed thoroughly. The vaccine trial should give them equitable advantages in trade, such as capacity building, transfer of technology and access to the vaccine during the current pandemic of COVID‐19.
Another concern is the availability of an adequate health facility and system to ensure that trial subjects and their families and/or communities have access to treatment and proper care in case of serious adverse events related to the trial outcomes. This must be assessed before any clinical trials begin. Providing the most comprehensive health services to the trial population will be an added value for population involvement in the trial. The best practice of vaccine clinical trials should have direct benefits for the community, such as improvement and availability of basic health facilities [ 37 ]
Vaccine acceptors are sometimes segmented into target groups, which is related to the host distribution of the target disease, for example by gender, age and specific population in the endemic area. A vaccine clinical trial is usually started in adult subjects and continued to more vulnerable subjects such as infants, young children, the elderly and women. Clinical vaccine trials will recruit vulnerable subjects. Protection measures to safeguard the vulnerable and marginalised populations should be carefully scrutinised during review. Ethical considerations must be adjusted to the individual situation to protect these vulnerable subjects from exploitation and later abandonment [ 38 ].
However, in an emergency pandemic situation, the definition of vulnerability needs to be openly discussed, and emergency calls for exceptions. The exclusion of vulnerable groups may diminish trial validity because of selection bias, so they should not be excluded without reasonable scientific and ethical justification [ 39 ].
Post‐trial access
After clinical vaccine trials, the subjects should have access to the developed vaccine. This is part of their direct advantage for their involvement in the research. While it is mentioned in the international ethical guidelines, not all researchers know and are aware of this important obligation [ 40 ]. The current COVID‐19 vaccine development involves multi‐country and intercontinental research recruiting subjects from different countries and regions. The post‐trial access to COVID‐19 vaccines should be expanded beyond the community where the trial is performed to include the country and region.
Post‐trial access is a matter which must be addressed from the very beginning of research design. Community engagement should be considered prior to the trial and involve all stakeholders: sponsors, industries, developers, investigators, subjects of the trial, communities and the government where the trial is performed.
In summary, the current COVID‐19 vaccine research and development involves people from many countries, which raises ethical issues that must be addressed by all stakeholders. Even in the emergency of a pandemic, the urgency of providing an effective COVID‐19 vaccine for humankind must be balanced with the exigency of research ethics that must be maintained. In any event, the safety and well‐being of research subjects must be protected, especially that of vulnerable subjects.
Sustainable Development Goals (SDGs): SDG 3 (good health and well‐being)
- Alzheimer's disease & dementia
- Arthritis & Rheumatism
- Attention deficit disorders
- Autism spectrum disorders
- Biomedical technology
- Diseases, Conditions, Syndromes
- Endocrinology & Metabolism
- Gastroenterology
- Gerontology & Geriatrics
- Health informatics
- Inflammatory disorders
- Medical economics
- Medical research
- Medications
- Neuroscience
- Obstetrics & gynaecology
- Oncology & Cancer
- Ophthalmology
- Overweight & Obesity
- Parkinson's & Movement disorders
- Psychology & Psychiatry
- Radiology & Imaging
- Sleep disorders
- Sports medicine & Kinesiology
- Vaccination
- Breast cancer
- Cardiovascular disease
- Chronic obstructive pulmonary disease
- Colon cancer
- Coronary artery disease
- Heart attack
- Heart disease
- High blood pressure
- Kidney disease
- Lung cancer
- Multiple sclerosis
- Myocardial infarction
- Ovarian cancer
- Post traumatic stress disorder
- Rheumatoid arthritis
- Schizophrenia
- Skin cancer
- Type 2 diabetes
- Full List »
share this!
April 15, 2024
This article has been reviewed according to Science X's editorial process and policies . Editors have highlighted the following attributes while ensuring the content's credibility:
fact-checked
reputable news agency
Why some adults may need another dose of measles vaccine
by Helena Oliviero, The Atlanta Journal-Constitution
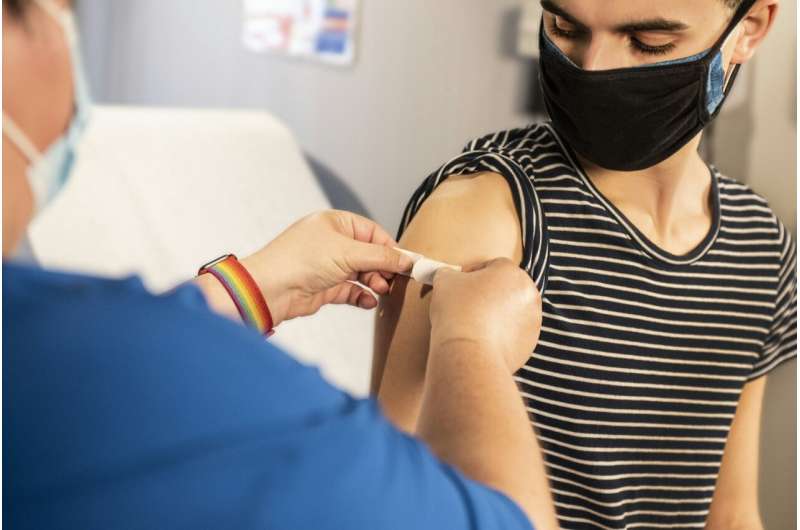
A rising number of measles cases in the U.S. this year is raising concerns over a comeback of a disease that was declared eliminated in this country 24 years ago.
A measles report from the Centers for Disease Control and Prevention last week concluded that the spike in cases means more public health efforts are needed to increase routine vaccination coverage.
This year there have been 121 infections reported in 17 states, according to the Centers for Disease Control and Prevention. That is well above the total of 58 cases documented for the country for all of 2023.
While the numbers might seem low, measles is a serious illness that can lead to severe complications.
The airborne virus is also highly contagious. If one person has it, up to 90% of the people close to that person who are not immune will also become infected, according to the CDC.
Falling vaccination rates and a rise in cases globally are raising questions for older adults who got a measles vaccine a long time ago, or maybe they aren't sure if they ever got vaccinated as a child.
Here's what you need to know about the measles vaccine and what to do if you are unsure about your vaccination status.
People born before 1957 are nearly always immune. The measles vaccine was made available in 1963, but in the decade before that virtually every child got measles by 15, making them immune. The CDC considers people born before 1957 to be protected from measles.
But for those born later, it may not be so simple. Measles vaccines first became available in 1963. Laws requiring a measles vaccine before attending school rolled out slowly and over the years so not all children were automatically vaccinated. Initially, there were two vaccines: a live attenuated version (which is a live form of the virus weakened in medical labs), or a "killed" vaccine (which contains the dead virus), which was not considered effective and is no longer used.
So some people who received the vaccine between 1963 and 1967 may have gotten a dose of the killed vaccine and they should consider getting an updated vaccine, according to Dr. Jayne Morgan, executive director of health and community education for Piedmont Healthcare.
The CDC said for those born after 1957, one dose of live vaccine is considered adequate unless a person is at high risk of exposure. Those at higher risk—such as those working in health care, who are attending college, or who travel internationally—should consider having two doses.
If you got the standard two doses of the measles vaccine after 1967, you should be protected against measles for life.
What if I don't know whether I'm immune?
For those unsure if they've either had measles or took the vaccine as a child, the CDC said there's no harm in taking another, now known as the measles, mumps and rubella (MMR) vaccine. While health care providers can do a blood test to determine immunity, that step is generally not recommended.
Jodie Guest, professor and vice chair of the department of epidemiology at Emory University's Rollins School of Public Health, also says if you are not sure of your immunity, go ahead and get vaccinated. "Go ahead and get the vaccine and do not worry about getting tested," Guest said.
Should younger adults also consider a measles vaccine?
Measles is a dangerous virus, so anyone of any age unsure of their vaccination status should get one. Guest said more than half of the people sickened with measles this year in the U.S. have required hospitalization, and close to a third of them were adults at least 20 years of age.
"We have likely forgotten how dangerous measles is as vaccination rates have been high and kept us safe," said Guest. "With vaccination rates falling, we are seeing a rise in a vaccine preventable illness that causes death and long-term, severe complications."
Severe complications from measles include pneumonia and encephalitis (swelling of the brain). Nearly one to three of every 1,000 children who become infected with measles will die from respiratory and neurologic complications, according to the CDC.
How effective is the MMR vaccine?
The measles vaccine is very effective, according to the CDC. Two doses of an MMR vaccine are about 97% effective at preventing measles if exposed to the virus. One dose is about 93% effective.
Very few people—about three out of 100—who get two doses of measles vaccine will still get measles if exposed to the virus, according to the CDC.
Experts aren't sure why. It could be that their immune systems didn't respond as well as they should have to the vaccine. But the good news is, fully vaccinated people who get measles seem more likely to have a milder illness.
2024 The Atlanta Journal-Constitution. Distributed by Tribune Content Agency, LLC.
Explore further
Feedback to editors

Occupations that are cognitively stimulating may be protective against later-life dementia
54 minutes ago

Researchers develop a new way to safely boost immune cells to fight cancer
18 hours ago

New compound from blessed thistle may promote functional nerve regeneration

New research defines specific genomic changes associated with the transmissibility of the mpox virus

New study confirms community pharmacies can help people quit smoking

Researchers discover glial hyper-drive for triggering epileptic seizures
19 hours ago

Deeper dive into the gut microbiome shows changes linked to body weight

A new therapeutic target for traumatic brain injury

Dozens of COVID virus mutations arose in man with longest known case, research finds

Researchers explore causal machine learning, a new advancement for AI in health care
Related stories.

Understanding measles and the current outbreak
Mar 27, 2024

What parents need to know about measles
Apr 8, 2024

US measles cases are up in 2024. What's driving the increase?
Apr 11, 2024

What is measles, and how can I shield myself & my family?
Feb 28, 2024

Will your old measles shot protect you from new outbreaks?
Apr 2, 2024

Preventing measles outbreaks in the US through vaccination
Aug 28, 2023
Recommended for you

How myeloid cell replacement could help treat autoimmune encephalomyelitis
23 hours ago

Retrospective genomic characterization of the 2020 Ebola outbreak
20 hours ago

Signs of multiple sclerosis show up in blood years before symptoms, study finds
Apr 19, 2024

DNA vaccine against Zika performs well in tests on mice
Apr 18, 2024
Let us know if there is a problem with our content
Use this form if you have come across a typo, inaccuracy or would like to send an edit request for the content on this page. For general inquiries, please use our contact form . For general feedback, use the public comments section below (please adhere to guidelines ).
Please select the most appropriate category to facilitate processing of your request
Thank you for taking time to provide your feedback to the editors.
Your feedback is important to us. However, we do not guarantee individual replies due to the high volume of messages.
E-mail the story
Your email address is used only to let the recipient know who sent the email. Neither your address nor the recipient's address will be used for any other purpose. The information you enter will appear in your e-mail message and is not retained by Medical Xpress in any form.
Newsletter sign up
Get weekly and/or daily updates delivered to your inbox. You can unsubscribe at any time and we'll never share your details to third parties.
More information Privacy policy
Donate and enjoy an ad-free experience
We keep our content available to everyone. Consider supporting Science X's mission by getting a premium account.
E-mail newsletter
Help | Advanced Search
Computer Science > Computation and Language
Title: leave no context behind: efficient infinite context transformers with infini-attention.
Abstract: This work introduces an efficient method to scale Transformer-based Large Language Models (LLMs) to infinitely long inputs with bounded memory and computation. A key component in our proposed approach is a new attention technique dubbed Infini-attention. The Infini-attention incorporates a compressive memory into the vanilla attention mechanism and builds in both masked local attention and long-term linear attention mechanisms in a single Transformer block. We demonstrate the effectiveness of our approach on long-context language modeling benchmarks, 1M sequence length passkey context block retrieval and 500K length book summarization tasks with 1B and 8B LLMs. Our approach introduces minimal bounded memory parameters and enables fast streaming inference for LLMs.
Submission history
Access paper:.
- HTML (experimental)
- Other Formats
References & Citations
- Google Scholar
- Semantic Scholar
BibTeX formatted citation

Bibliographic and Citation Tools
Code, data and media associated with this article, recommenders and search tools.
- Institution
arXivLabs: experimental projects with community collaborators
arXivLabs is a framework that allows collaborators to develop and share new arXiv features directly on our website.
Both individuals and organizations that work with arXivLabs have embraced and accepted our values of openness, community, excellence, and user data privacy. arXiv is committed to these values and only works with partners that adhere to them.
Have an idea for a project that will add value for arXiv's community? Learn more about arXivLabs .
Homelessness and the Persistence of Deprivation: Income, Employment, and Safety Net Participation
Homelessness is arguably the most extreme hardship associated with poverty in the United States, yet people experiencing homelessness are excluded from official poverty statistics and much of the extreme poverty literature. This paper provides the most detailed and accurate portrait to date of the level and persistence of material disadvantage faced by this population, including the first national estimates of income, employment, and safety net participation based on administrative data. Starting from the first large and nationally representative sample of adults recorded as sheltered and unsheltered homeless taken from the 2010 Census, we link restricted-use longitudinal tax records and administrative data on the Supplemental Nutrition Assistance Program (SNAP), Medicare, Medicaid, Disability Insurance (DI), Supplemental Security Income (SSI), veterans’ benefits, housing assistance, and mortality. Nearly half of these adults had formal employment in the year they were observed as homeless, and nearly all either worked or were reached by at least one safety net program. Nevertheless, their incomes remained low for the decade surrounding an observed period of homelessness, suggesting that homelessness tends to arise in the context of long-term, severe deprivation rather than large and sudden losses of income. People appear to experience homelessness because they are very poor despite being connected to the labor market and safety net, with low permanent incomes leaving them vulnerable to the loss of housing when met with even modest disruptions to life circumstances.
The Census Bureau has reviewed this data product for unauthorized disclosure of confidential information and has approved the disclosure avoidance practices applies to this release, authorization number CBDRB-FY2022-CES005-015. We thank the U.S. Census Bureau for their support, as well as John Abowd, Mark Asiala, George Carter, James Christy, Dennis Culhane, Kevin Deardorff, Conor Dougherty, Ingrid Gould Ellen, Anne Fletcher, Katie Genadek, Tatiana Homonoff, Kristin Kerns, William Koerber, Margot Kushel, Larry Locklear, Tim Marshall, Brian McKenzie, Brendan O’Flaherty, James Pugh, Trudi Renwick, Annette Riorday, Nan Roman, William Snow, Eddie Thomas, Matthew Turner, and John Voorheis for providing feedback and answering questions. We also thank participants in seminars at Yale University (Labor/Public Economics Workshop), the University of Chicago (Demography Workshop), APPAM, NTA, NBER Labor Studies, IRS/Census (Income Measurement Workshop), and the Institute for Research on Poverty. Ilina Logani and Mandana Vakil provided excellent research assistance. We appreciate the financial support of the Alfred P. Sloan Foundation, the Russell Sage Foundation, the Charles Koch Foundation, the Menard Family Foundation, and the American Enterprise Institute. Wyse thanks the National Institute on Aging for their support. The views expressed herein are those of the authors and do not necessarily reflect the views of the National Bureau of Economic Research.
MARC RIS BibTeΧ
Download Citation Data
- data appendix
Working Groups
More from nber.
In addition to working papers , the NBER disseminates affiliates’ latest findings through a range of free periodicals — the NBER Reporter , the NBER Digest , the Bulletin on Retirement and Disability , the Bulletin on Health , and the Bulletin on Entrepreneurship — as well as online conference reports , video lectures , and interviews .
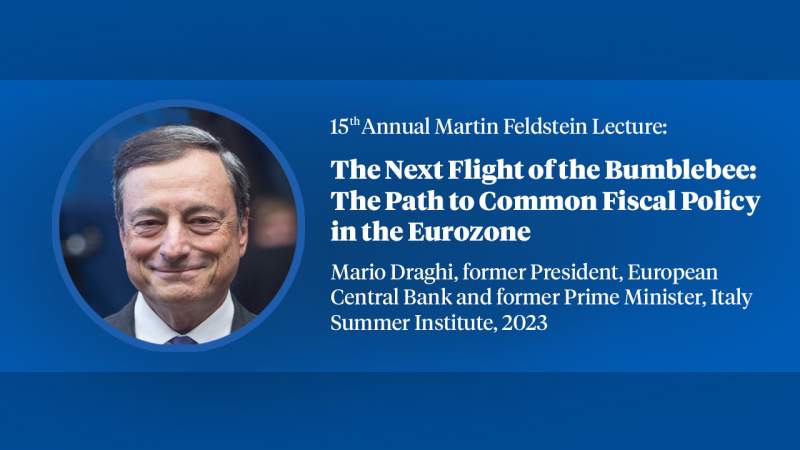
Thank you for visiting nature.com. You are using a browser version with limited support for CSS. To obtain the best experience, we recommend you use a more up to date browser (or turn off compatibility mode in Internet Explorer). In the meantime, to ensure continued support, we are displaying the site without styles and JavaScript.
- View all journals
- My Account Login
- Explore content
- About the journal
- Publish with us
- Sign up for alerts
- Open access
- Published: 13 April 2024
The role of correlates of protection in overcoming barriers to vaccine development and demonstrating efficacy
- Deborah F. King ORCID: orcid.org/0009-0006-3514-9245 1 ,
- Helen Groves 1 &
- Charlotte Weller 1
npj Vaccines volume 9 , Article number: 78 ( 2024 ) Cite this article
268 Accesses
5 Altmetric
Metrics details
- Infectious diseases
Barriers to vaccine development
Vaccine development requires the conduct of clinical trials to generate the necessary safety, quality and efficacy data needed for licensure 1 . Starting initially with small trials to demonstrate safety, and progressively scaling up to look at immunogenicity and finally efficacy, this process has resulted in the development of vaccines against 25 diseases, with more in the pipeline 2 which have significantly reduced morbidity and mortality since their introduction 3 .
Vaccine development takes on average 10–15 years and costs at least $500 m to bring a new product to market, with probability of success estimated to be as low as 10% 4 The high level of investment of both time and money needed to progress vaccine candidates combined with high risk of failure can disincentivize development of some products. The feasibility of clinical development and the likelihood of regulatory approval are key drivers of developer decision-making when considering which products to progress, especially as vaccines move towards pivotal efficacy studies which require significant investment 5 . Whilst quality and safety standards are well defined, establishing efficacy can be challenging.
Given that infectious diseases still kill millions of people worldwide, particularly in Africa, some parts of south-east Asia and south America 6 alternative strategies must be considered to address the challenges of progressing vaccines through late-stage development.
These challenges can be technical, such as low disease incidence, diagnostic or enrolment challenges, or lack of understanding of protective immunity in target populations. Challenges can also be financial, with candidate vaccines failing to attract the necessary level of investment to conduct efficacy trials if market demand or return on investment are uncertain, falling into “the second valley of death” between late-stage clinical development and licensure 7 .
The majority of vaccines licenced to date have been assessed for efficacy against clinical disease endpoints in randomised controlled trials (RCT’s), this approach is viewed as the gold standard method to demonstrate the efficacy data required by regulators for licensure. However, this traditional approach is not always feasible in certain situations.
The outbreak of Sudan ebolavirus (SUDV) in Uganda in 2022 highlights this issue for emerging infectious diseases. When the outbreak of SUDV was declared in Uganda 8 , there were no licenced vaccines, but there were 3 vaccine candidates in development 9 Plans were rapidly drawn up by Ugandan authorities and the WHO, to conduct a “ring vaccination” study using WHO’s SOLIDARITY trials core protocol to assess the effect of a single vaccine dose in protecting recent contacts of newly confirmed cases of SUVD against lab-confirmed SUVD 10 The first doses of candidate vaccines arrived in Uganda just 79 days after the outbreak was declared. However, before the trial started, the outbreak was declared over. Given the deadly nature of ebolavirus disease (this outbreak recorded 142 confirmed cases and 55 confirmed deaths) medical counter measures to control outbreaks are still urgently needed. Whilst the control and eventual ending of the outbreak was achieved through leadership, teamwork, contact tracing testing and control measures such as quarantines and lockdowns, such measures are not without their significant downsides, particularly for the poorest people in societies where not working means no income to support their families 11 .
This issue does not only affect emerging infectious diseases, other examples include:
Where disease incidence is low e.g. a cluster-randomised ring vaccination trial for a Nipah virus vaccine was estimated to take 516 years and over 163,000 vaccine doses under current epidemic conditions 12 .
Where large trials are required e.g. licensure of a maternal GBS vaccine to prevent neonatal disease require enrolment of up to 80,000 pregnant women 13 , 14 , or prevention of enteric fever caused by S. paratyphi A, where low attack rates mean efficacy studies would require 100,000–250,000 participants 15 .
Where unpredictable market demand and return on investment mean products fail to attract necessary investment e.g. new TB vaccines 16 , 17 .
The need to understand protective immunity: the case for correlates of protection
Identification of correlates of protection, (immune responses associated with protection from disease), that can act as predictors of efficacy has the potential to unlock the development of safe, technically promising and potentially life-saving vaccines. Supporting research into discovery and use CoP data has the potential to improve go/no-go decision making in clinical development, allow rationale design of new or improved vaccines, reduce the time and cost of phase 3 testing by informing clinical trial design and provide a pathway to continue development when clinical efficacy studies are unfeasible. Coupling CoP-based approaches with post-authorisation studies to demonstrate effectiveness could lead to licensure when clinical efficacy cannot be feasibly achieved.
Barriers to the use of correlates of protection, and solutions: The 4 C’s
In September 2022 Wellcome convened vaccine developers, regulators and policy makers to determine how to embed the identification and validation of correlates of protection early in the clinical development process and enable their use throughout.
Gaps in consistency of data collection and analysis, lack of collaboration and co-ordination between stakeholders and clear communication of evidence gaps and how to address these were all identified as challenges (workshop report in press).
Conclusions
Licenced vaccines have traditionally been designed empirically, and achieved authorisation through RCT’s based on clinical endpoints to demonstrate efficacy. However, such approaches are not always feasible and have not been successful against more technically challenging targets such as HIV. Alternative approaches to inform rationale vaccine design, demonstrating effectiveness and increasing probabilities of success are needed to stop new products stalling in development, and leaving vulnerable populations at risk of morbidity and mortality caused by infectious diseases.
Developing new methods to define protective immune responses, coupled with commitment to post-introduction studies to ensure safety and effectiveness, has the potential to improve our understanding of protective immunity in target populations which in turn will inform vaccine design, development and use.
Without new approaches to develop vaccines, many communities will continue to bear the burden of infectious diseases and are exposed to the risk of infectious diseases escalating. The COVID-19 pandemic reminded us all of the devastating impact infectious diseases can have on our lives, and whilst multiple vaccines were rapidly developed for this disease, new vaccines are still needed to prevent death and disability caused by endemic diseases such as TB, and to be prepared for future outbreaks. Given the growing threats to controlling infectious diseases such as climate change and antimicrobial resistance, it is important to act now to ensure that we are better prepared to tackle infectious diseases that affect everyone now and in the future.
Singh, K. & Mehta, S. The clinical development process for a novel preventive vaccine: An overview. J. Postgrad. Med. 62 , 4 (2016).
Article CAS PubMed PubMed Central Google Scholar
Immunization, Vaccines and Biologicals, https://www.who.int/teams/immunization-vaccines-and-biologicals/diseases (2024).
Immunization, https://www.who.int/news-room/facts-in-pictures/detail/immunization (2024).
MacPherson, A. et al. Probability of success and timelines for the development of vaccines for emerging and reemerged viral infectious diseases. Ann Intern Med . 174, 326–334 (2021).
A vaccine ecosystem equipped to meet the challenges of future infectious disease. Wellcome, https://wellcome.org/reports/effective-vaccine-ecosystem-equipped-meet-challenges-future-infectious-disease-threats (2024).
Death rate from infectious diseases, 2019, https://ourworldindata.org/grapher/infectious-disease-death-rates-gbd (2024).
Kaslow, D. C. et al. Vaccine candidates for poor nations are going to waste. Nature 564 , 337–339 (2021).
Article Google Scholar
Ebola outbreak 2022 - Uganda, https://www.who.int/emergencies/situations/ebola-uganda-2022 (2024).
WHO Technical Advisory Group on candidate vaccine prioritization. Summary of the evaluations and recommendations on the three Sudan ebolavirus vaccines that are candidates for inclusion in the planned ring vaccination trial in Uganda (“Tokomeza Ebola”), https://www.who.int/publications/m/item/who-vaccine-prioritization-working-group.--summary-of-the-evaluations-and-recommendations-on-the-three-sudan-ebolavirus-vaccines-that-are-candidates-for-inclusion-in-the-planned-ring-vaccination-trial-in-uganda-(-tokomeza-ebola) (2024).
Solidarity Trials Core Protocol - Ring vaccination trial to evaluate the efficacy and safety of a Sudan ebolavirus vaccine in Uganda, https://www.who.int/publications/m/item/solidarity-trials-core-protocol---ring-vaccination-trial-to-evaluate-the-efficacy-and-safety-of-a-sudan-ebolavirus-vaccine-in-uganda (2024).
Ebola in Uganda: lessons from COVID show that heavy-handed lockdowns may be a bad idea. Gavi, the Vaccine Alliance, https://www.gavi.org/vaccineswork/ebola-uganda-lessons-covid-show-heavy-handed-lockdowns-may-be-bad-idea (2024).
Nikolay, B. et al. Assessing the feasibility of Nipah vaccine efficacy trials based on previous outbreaks in Bangladesh. Vaccine 39 , 5600–5606 (2021).
Article CAS PubMed Google Scholar
Absalon, J. et al. Advances towards licensure of a maternal vaccine for the prevention of invasive group B streptococcus disease in infants: a discussion of different approaches. Hum Vaccin Immunother . 18 , https://www.tandfonline.com/doi/abs/10.1080/21645515.2022.2037350 (2022).
Madhi, S. A. et al. Considerations for a phase-III trial to evaluate a group B Streptococcus polysaccharide-protein conjugate vaccine in pregnant women for the prevention of early- and late-onset invasive disease in young-infants. Vaccine 31 , D52–D57 (2013).
Martin, L. B. et al. Vaccine value profile for Salmonella enterica serovar Paratyphi A. Vaccine 41 , S114–S133 (2023).
Article PubMed Google Scholar
Cobelens, F. et al. Accelerating research and development of new vaccines against tuberculosis: a global roadmap. Lancet Infect. Dis. 22 , e108 (2022).
Article PubMed PubMed Central Google Scholar
Kochhar, S. et al. Building the concept for WHO Evidence Considerations for Vaccine Policy (ECVP): Tuberculosis vaccines intended for adults and adolescents as a test case. Vaccine 40 , 1681 (2022).
Download references
Acknowledgements
D.K., H.G. and C.W. are all employees of Wellcome. All views expressed are those of the authors and do not necessarily reflect those of the organisation.
Author information
Authors and affiliations.
Infection Disease Strategic Programme, Wellcome, 215 Euston Road, London, UK
Deborah F. King, Helen Groves & Charlotte Weller
You can also search for this author in PubMed Google Scholar
Contributions
D.F.K., H.G. and C.W. developed the Comment outline, D.F.K. drafted the article. All authors reviewed the article and agreed the submitted version.
Corresponding author
Correspondence to Deborah F. King .
Ethics declarations
Competing interests.
The authors declare no competing interests.
Additional information
Publisher’s note Springer Nature remains neutral with regard to jurisdictional claims in published maps and institutional affiliations.
Rights and permissions
Open Access This article is licensed under a Creative Commons Attribution 4.0 International License, which permits use, sharing, adaptation, distribution and reproduction in any medium or format, as long as you give appropriate credit to the original author(s) and the source, provide a link to the Creative Commons licence, and indicate if changes were made. The images or other third party material in this article are included in the article’s Creative Commons licence, unless indicated otherwise in a credit line to the material. If material is not included in the article’s Creative Commons licence and your intended use is not permitted by statutory regulation or exceeds the permitted use, you will need to obtain permission directly from the copyright holder. To view a copy of this licence, visit http://creativecommons.org/licenses/by/4.0/ .
Reprints and permissions
About this article
Cite this article.
King, D.F., Groves, H. & Weller, C. The role of correlates of protection in overcoming barriers to vaccine development and demonstrating efficacy. npj Vaccines 9 , 78 (2024). https://doi.org/10.1038/s41541-024-00873-5
Download citation
Received : 03 November 2023
Accepted : 28 March 2024
Published : 13 April 2024
DOI : https://doi.org/10.1038/s41541-024-00873-5
Share this article
Anyone you share the following link with will be able to read this content:
Sorry, a shareable link is not currently available for this article.
Provided by the Springer Nature SharedIt content-sharing initiative
Quick links
- Explore articles by subject
- Guide to authors
- Editorial policies
Sign up for the Nature Briefing: Microbiology newsletter — what matters in microbiology research, free to your inbox weekly.

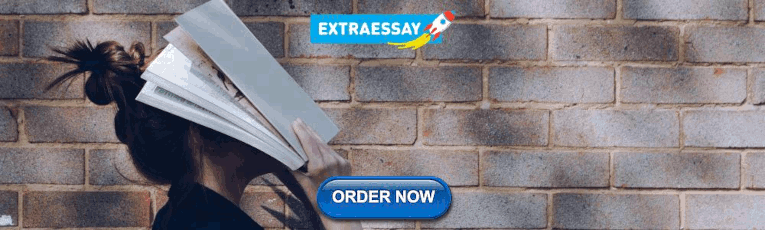
IMAGES
VIDEO
COMMENTS
Metrics. Vaccines have revolutionized modern medicine by preventing infectious diseases and safeguarding public health. This Collection showcases cutting-edge research on advancements in vaccine ...
Introduction. The coronavirus disease 2019 (COVID-19) pandemic caused by the severe acute respiratory syndrome coronavirus 2 (SARS-CoV-2) has resulted in over 192 million cases and 4.1 million deaths as of July 22, 2021. 1 This pandemic has brought along a massive burden in morbidity and mortality in the healthcare systems. Despite the implementation of stringent public health measures, there ...
A recent test-negative design study in Canada showed positive findings regarding vaccine effectiveness with the BNT162b2, mRNA-1273, and ChAdOx1 nCoV-19 vaccines against symptomatic disease with ...
VOL. 387 NO. 11. The coronavirus disease 2019 (Covid-19) pandemic has claimed an estimated 15 million lives, including more than 1 million lives in the United States alone. The rapid development ...
A two-dose regimen of BNT162b2 (30 μg per dose, given 21 days apart) was found to be safe and 95% effective against Covid-19. The vaccine met both primary efficacy end points, with more than a 99 ...
To date, coronavirus disease 2019 (COVID-19) becomes increasingly fierce due to the emergence of variants. Rapid herd immunity through vaccination is needed to block the mutation and prevent the emergence of variants that can completely escape the immune surveillance. We aimed to systematically evaluate the effectiveness and safety of COVID-19 vaccines in the real world and to establish a ...
Our analyses indicate that vaccine effectiveness generally decreases over time against SARS-CoV-2 infections, hospitalisations, and mortality. The baseline vaccine effectiveness levels for the omicron variant were notably lower than for other variants. Therefore, other preventive measures (eg, face-mask wearing and physical distancing) might be necessary to manage the pandemic in the long term.
2020 has been a difficult year for all, but has seen 58 vaccines against severe acute respiratory syndrome coronavirus 2 (SARS-CoV-2) be developed and in clinical trials,1 with some vaccines reportedly having more than 90% efficacy against COVID-19 in clinical trials. This remarkable achievement is much-needed good news as COVID-19 cases are currently at their highest daily levels globally.2 ...
There is no question that the current vaccines are effective and safe. The risk of severe reaction to a COVID-19 jab, say researchers, is outweighed by the protection it offers against the deadly ...
Vaccines are a clinical product that is composed of live or dead material from an infectious agent - bacterium, virus, fungus or parasite - that elicit protective immunity against the pathogen ...
Brief History of Vaccine Development. Human use of preparations to prevent specific infections have been described since 1500 AD, beginning in China (Needham, 2000) where smallpox was prevented by variolation, which is the introduction of material from scabs into the skin.In 1796 in the United Kingdom, Edward Jenner observed the immunity to smallpox of milkmaids having previously had natural ...
The total average number of citations per year was 24.7 in 2020, 11.5 in 2021, 5.8 in 2022, and 1.2 in 2023. Figure 2 (A) Annual related publication from 2019 to 2023 per year, and (B) average article and average article citations from 2019 to 2023 in COVID-19 RNA vaccine-related research.
Keywords: COVID-19, SARS-CoV-2, vaccines, CoronaVac, review Citation: Alzate-Ángel JC, Avilés-Vergara PA, Arango-Londoño D, Concha-Eastman A, Garcés-Hurtado A, López-Carvajal L, Minotta IL, Ortega-Lenis D, Quintero G, Reina-Bolaños S, Reina-Bolaños CA, Roa P, Sánchez-Orozco M, Tovar-Acero C and Arbeláez-Montoya MP (2024) How has research on the effectiveness and safety of COVID-19 ...
Vaccines not only afford the best protection against infectious disease but can serve as strong deterrence factors as well. From a bioterrorist perspective, vaccine-resistant agents are more difficult to engineer than drug-resistant agents. But the potential market has been too small and uncertain to encourage the vaccine industry to make large investments in research, development, and ...
Vaccination Behavior Research Topics. Unraveling demand and supply effects on the up-take of influenza vaccinations. Point out new approaches to the seasonal flu vaccine. Exploring the impact of vaccination. Investigating patient experience with, and the use of, an electronic monitoring system to assess vaccination responses.
Vaccines are one of the greatest public health advancements of all time, resulting in the control, elimination, or near-elimination of numerous infectious diseases that were once pervasive and often fatal. Pfizer has a rich history in vaccine research and development. Over the years, we've played a pivotal role in eliminating or nearly ...
The Coronavirus Efficacy (COVE) phase 3 trial was launched in late July 2020 to assess the safety and efficacy of the mRNA-1273 vaccine in preventing SARS-CoV-2 infection. An independent data and ...
Americans' Largely Positive Views of Childhood Vaccines Hold Steady. About nine-in-ten (88%) Americans say, overall, the benefits of childhood vaccines for measles, mumps and rubella outweigh the risks, identical to the share who said this before the coronavirus outbreak. U.S. adults are less confident in COVID-19 vaccines: Fewer than half ...
Vaccine platforms and vaccines for emerging infectious diseases. Vaccines are the cornerstone of the management of infectious disease outbreaks and are the surest means to defuse pandemic and ...
As science advances and new information becomes available, this system will continue to improve. Vaccine safety research: Ensures the benefits of vaccines approved in the U.S. outweigh the risks. Defines which groups should not receive certain vaccines. Describes side effects and adverse events reported after vaccination.
WASHINGTON — A new report from the National Academies of Sciences, Engineering, and Medicine reviews evidence for 19 potential harms of the COVID-19 vaccines, and for nine potential shoulder injuries from intramuscular administration of vaccines more broadly. The committee that conducted the review identified sufficient evidence to draw 20 conclusions about whether these vaccines could cause ...
A Feature Paper should be a substantial original Article that involves several techniques or approaches, provides an outlook for future research directions and describes possible research applications. Feature papers are submitted upon individual invitation or recommendation by the scientific editors and must receive positive feedback from the ...
Vaccination mandates in the U.S. date back to the 19th century, when many cities and states started requiring children to be immunized against smallpox; the Supreme Court upheld such mandates in a landmark 1905 decision. A combination vaccine against diphtheria, tetanus and pertussis became available in 1948, and it was quickly added as a ...
The achievements of vaccine research and development bring a hope to our societies that we may cope with the COVID‐19 pandemic. There are two aspects that should be maintained in balance: the immediate necessity for speed of vaccine research and the inherent need for protection of research subjects, which is the foremost concern of research ethics.
Scientific Research, vital for improving human life, is hindered by its inherent complexity, slow pace, and the need for specialized experts. To enhance its productivity, we propose a ResearchAgent, a large language model-powered research idea writing agent, which automatically generates problems, methods, and experiment designs while iteratively refining them based on scientific literature ...
The measles vaccine is very effective, according to the CDC. Two doses of an MMR vaccine are about 97% effective at preventing measles if exposed to the virus. One dose is about 93% effective ...
Leave No Context Behind: Efficient Infinite Context Transformers with Infini-attention. This work introduces an efficient method to scale Transformer-based Large Language Models (LLMs) to infinitely long inputs with bounded memory and computation. A key component in our proposed approach is a new attention technique dubbed Infini-attention.
S.B. HalsteadN Engl J Med 2024;390:464-465. In 2019, the four serotypes of the mosquito-borne dengue virus (DENV) caused an estimated 56 million cases of disease and 5000 to 40,000 deaths in a ...
This paper provides the most detailed and accurate portrait to date of the level and persistence of material disadvantage faced by this population, including the first national estimates of income, employment, and safety net participation based on administrative data.
Supporting research into discovery and use CoP data has the potential to improve go/no-go decision making in clinical development, allow rationale design of new or improved vaccines, reduce the ...