Advertisement
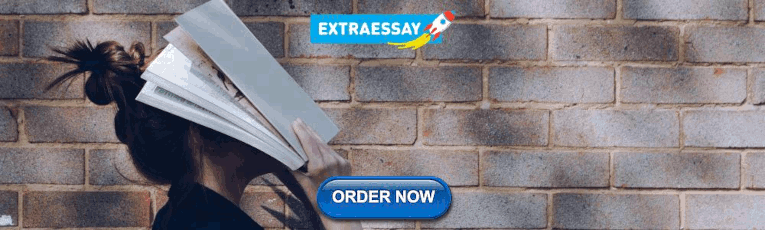
Transfersomes: a Revolutionary Nanosystem for Efficient Transdermal Drug Delivery
- Review Article
- Published: 01 December 2021
- Volume 23 , article number 7 , ( 2022 )
Cite this article
- Muhammad Waseem Akram 1 ,
- Humzah Jamshaid 1 ,
- Fiza Ur Rehman 1 ,
- Muhammad Zaeem 2 ,
- Jehan zeb Khan 1 &
- Ahmad Zeb 1
2878 Accesses
28 Citations
Explore all metrics
Transdermal delivery system has gained significance in drug delivery owing to its advantages over the conventional delivery systems. However, the barriers of stratum corneum along with skin irritation are its major limitations. Various physical and chemical techniques have been employed to alleviate these impediments. Among all these, transfersomes have shown potential for overcoming the associated limitations and successfully delivering therapeutic agents into systemic circulation. These amphipathic vesicles are composed of phospholipids and edge activators. Along with providing elasticity, edge activator also affects the vesicular size and entrapment efficiency of transfersomes. The mechanism behind the enhanced permeation of transfersomes through the skin involves their deformability and osmotic gradient across the application site. Permeation enhancers can further enhance their permeability. Biocompatibility; capacity for carrying hydrophilic, lipophilic as well as high molecular weight therapeutics; deformability; lesser toxicity; enhanced permeability; and scalability along with potential for surface modification, active targeting, and controlled release render them ideal designs for efficient drug delivery. The current review provides a brief account of the discovery, advantages, composition, synthesis, comparison with other cutaneous nano-drug delivery systems, applications, and recent developments in this area.
This is a preview of subscription content, log in via an institution to check access.
Access this article
Price includes VAT (Russian Federation)
Instant access to the full article PDF.
Rent this article via DeepDyve
Institutional subscriptions
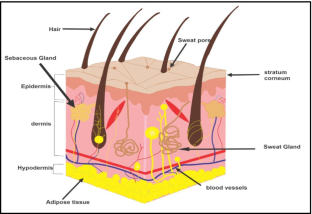
Similar content being viewed by others
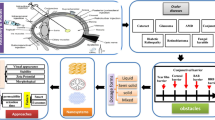
Ocular Drug Delivery: a Comprehensive Review
Sadek Ahmed, Maha M. Amin & Sinar Sayed
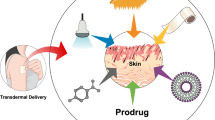
Enhancement strategies for transdermal drug delivery systems: current trends and applications
Delly Ramadon, Maeliosa T. C. McCrudden, … Ryan F. Donnelly
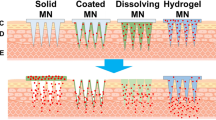
Microneedle for transdermal drug delivery: current trends and fabrication
Jae Hwan Jung & Sung Giu Jin
Thomas BJ, Finnin BC. The transdermal revolution. Drug Discovery Today. 2004;9(16):697–703.
Article CAS PubMed Google Scholar
Abd El-Alim SH, Kassem AA, Basha M, Salama A. Comparative study of liposomes, ethosomes and TFS as carriers for enhancing the transdermal delivery of diflunisal: in vitro and in vivo evaluation. Int J Pharm. 2019;563:293–303.
Marwah H, Garg T, Goyal AK, Rath G. Permeation enhancer strategies in transdermal drug delivery. Drug Delivery. 2016;23(2):564–78.
Ahad A, Al-Saleh AA, Al-Mohizea AM, Al-Jenoobi FI, Raish M, Yassin AEB, et al. Formulation and characterization of novel soft nanovesicles for enhanced transdermal delivery of eprosartan mesylate. Saudi Pharmaceutical Journal. 2017;25(7):1040–6.
Article PubMed PubMed Central Google Scholar
Dudhipala N, Phasha Mohammed R, Adel Ali Youssef A, Banala N. Effect of lipid and edge activator concentration on development of aceclofenac-loaded TFS gel for transdermal application: in vitro and ex vivo skin permeation. Drug development and industrial pharmacy. 2020;46(8):1334–44.
Khatoon K, Rizwanullah M, Amin S, Mir SR, Akhter S. Cilnidipine loaded TFS for transdermal application: formulation optimization, in-vitro and in-vivo study. Journal of Drug Delivery Science and Technology. 2019;54:101303.
Prausnitz MR, Langer R. Transdermal drug delivery. Nat Biotechnol. 2008;26(11):1261–8.
Article CAS PubMed PubMed Central Google Scholar
Desai JL, Pandya T, Patel A. Nanocarriers in Transdermal Drug Delivery. Nanocarriers: Drug Delivery System: Springer; 2021. p. 383–409.
Menon GK. New insights into skin structure: scratching the surface. Adv Drug Deliv Rev. 2002;54:S3–17.
Alonso MJ, Sánchez A. The potential of chitosan in ocular drug delivery. J Pharm Pharmacol. 2003;55(11):1451–63.
Benson HAE. Transdermal drug delivery: penetration enhancement techniques. Curr Drug Deliv. 2005;2(1):23–33.
Benson HAE, Namjoshi S. Proteins and peptides: strategies for delivery to and across the skin. J Pharm Sci. 2008;97(9):3591–610.
Morrow DIJ, McCarron PA, Woolfson AD, Donnelly RF. Innovative strategies for enhancing topical and transdermal drug delivery. The Open Drug Delivery Journal. 2007;1(1).
Shreya AB, Managuli RS, Menon J, Kondapalli L, Hegde AR, Avadhani K, et al. Nano-transfersomal formulations for transdermal delivery of asenapine maleate: in vitro and in vivo performance evaluations. J Liposome Res. 2016;26(3):221–32.
Chuang S-Y, Lin C-H, Huang T-H, Fang J-Y. Lipid-based nanoparticles as a potential delivery approach in the treatment of rheumatoid arthritis. Nanomaterials. 2018;8(1):42.
Article PubMed Central Google Scholar
Yu G, Ali Z, Khan AS, Ullah K, Jamshaid H, Zeb A, et al. Preparation, Pharmacokinetics, and Antitumor Potential of Miltefosine-Loaded Nanostructured Lipid Carriers. Int J Nanomed. 2021;16:3255.
Article Google Scholar
Abdellatif AAH, Tawfeek HM. Transfersomal nanoparticles for enhanced transdermal delivery of clindamycin. AAPS PharmSciTech. 2016;17(5):1067–74.
Jamshaid H, ud Din F, Khan GM. Nanotechnology based solutions for anti-leishmanial impediments: a detailed insight. Journal of Nanobiotechnology. 2021;19(1):1–51.
Cevc G. Lipid vesicles and other colloids as drug carriers on the skin. Adv Drug Deliv Rev. 2004;56(5):675–711.
Cevc G, Schätzlein A, Blume G. Transdermal drug carriers: basic properties, optimization and transfer efficiency in the case of epicutaneously applied peptides. J Control Release. 1995;36(1–2):3–16.
Article CAS Google Scholar
Jiang T, Wang T, Li T, Ma Y, Shen S, He B, et al. Enhanced transdermal drug delivery by transfersome-embedded oligopeptide hydrogel for topical chemotherapy of melanoma. ACS Nano. 2018;12(10):9693–701.
Ujjwal N, Sakshi S. TFS: novel approach for transdermal delivery. Eur J Pharm Med Res. 2015;2(3):218–33.
Google Scholar
Rai S, Pandey V, Rai G. TFS as versatile and flexible nano-vesicular carriers in skin cancer therapy: the state of the art. Nano reviews & experiments. 2017;8(1):1325708.
Aggarwal N, Goindi S. Preparation and evaluation of antifungal efficacy of griseofulvin loaded deformable membrane vesicles in optimized guinea pig model of Microsporum canis—Dermatophytosis. Int J Pharm. 2012;437(1–2):277–87.
Lei W, Yu C, Lin H, Zhou X. Development of tacrolimus-loaded TFS for deeper skin penetration enhancement and therapeutic effect improvement in vivo. Asian J Pharm Sci. 2013;8(6):336–45.
Rajan R, Jose S, Mukund VPB, Vasudevan DT. Transferosomes-a vesicular transdermal delivery system for enhanced drug permeation. Journal of advanced pharmaceutical Technology & Research. 2011;2(3):138.
Ahad A, Al-Saleh AA, Al-Mohizea AM, Al-Jenoobi FI, Raish M, Yassin AEB, et al. Formulation and characterization of Phospholipon 90 G and tween 80 based TFS for transdermal delivery of eprosartan mesylate. Pharm Dev Technol. 2018;23(8):787–93.
Dai Y, Zhou R, Liu L, Lu Y, Qi J, Wu W. Liposomes containing bile salts as novel ocular delivery systems for tacrolimus (FK506): in vitro characterization and improved corneal permeation. Int J Nanomed. 2013;8:1921.
Joshi A, Kaur J, Kulkarni R, Chaudhari R. In-vitro and ex-vivo evaluation of raloxifene hydrochloride delivery using nano-transfersome based formulations. Journal of Drug Delivery Science and Technology. 2018;45:151–8.
Garg V, Singh H, Bimbrawh S, Kumar Singh S, Gulati M, Vaidya Y, et al. Ethosomes and TFS: principles, perspectives and practices. Curr Drug Deliv. 2017;14(5):613–33.
Bragagni M, Mennini N, Maestrelli F, Cirri M, Mura P. Comparative study of liposomes, TFS and ethosomes as carriers for improving topical delivery of celecoxib. Drug Delivery. 2012;19(7):354–61.
Sana E, Zeeshan M, Ain QU, Khan AU, Hussain I, Khan S, et al. Topical delivery of curcumin-loaded TFS gel ameliorated rheumatoid arthritis by inhibiting NF-κβ pathway. Nanomedicine. 2021;16(10):819–37.
Ishii F, Nii T. Lipid emulsions and lipid vesicles prepared from various phospholipids as drug carriers. Colloid and interface science in pharmaceutical research and development: Elsevier; 2014. p. 469–501.
Zeb A, Qureshi OS, Kim H-S, Cha J-H, Kim H-S, Kim J-K. Improved skin permeation of methotrexate via nanosized ultradeformable liposomes. Int J Nanomed. 2016;11:3813.
El Zaafarany GM, Awad GAS, Holayel SM, Mortada ND. Role of edge activators and surface charge in developing ultradeformable vesicles with enhanced skin delivery. Int J Pharm. 2010;397(1–2):164–72.
Article PubMed Google Scholar
El-Gizawy SA, Nouh A, Saber S, Kira AY. Deferoxamine-loaded TFS accelerates healing of pressure ulcers in streptozotocin-induced diabetic rats. Journal of Drug Delivery Science and Technology. 2020;58:101732.
Balata GF, Faisal MM, Elghamry HA, Sabry SA. Preparation and characterization of ivabradine HCl TFS for enhanced transdermal delivery. Journal of Drug Delivery Science and Technology. 2020;60:101921.
Tawfeek HM, Abdellatif AAH, Abdel-Aleem JA, Hassan YA, Fathalla D. Transfersomal gel nanocarriers for enhancement the permeation of lornoxicam. Journal of Drug Delivery Science and Technology. 2020;56:101540.
Pawar AY. Transfersome: A novel technique which improves transdermal permeability. Asian Journal of Pharmaceutics (AJP): Free full text articles from Asian J Pharm. 2016;10(04).
Solanki D, Kushwah L, Motiwale M, Chouhan V. Transferosomes-a review. World Journal of Pharmacy and Pharmaceutical Sciences. 2016;5:435–49.
CAS Google Scholar
Lane ME. Skin penetration enhancers. Int J Pharm. 2013;447(1–2):12–21.
Williams AC, Barry BW. Penetration enhancers. Adv Drug Deliv Rev. 2012;64:128–37.
Sinha VR, Kaur MP. Permeation enhancers for transdermal drug delivery. Drug Dev Ind Pharm. 2000;26(11):1131–40.
Rajan R, Vasudevan DT. Effect of permeation enhancers on the penetration mechanism of transfersomal gel of ketoconazole. Journal of advanced pharmaceutical technology & research. 2012;3(2):112.
Omar MM, Hasan OA, El Sisi AM. Preparation and optimization of lidocaine transferosomal gel containing permeation enhancers: a promising approach for enhancement of skin permeation. Int J Nanomed. 2019;14:1551.
Shamma RN, Elsayed I. Transfersomal lyophilized gel of buspirone HCl: formulation, evaluation and statistical optimization. J Liposome Res. 2013;23(3):244–54.
Ahad A, Aqil M, Kohli K, Sultana Y, Mujeeb M. Design, formulation and optimization of valsartan transdermal gel containing iso-eucalyptol as novel permeation enhancer: preclinical assessment of pharmacokinetics in Wistar albino rats. Expert Opin Drug Deliv. 2014;11(8):1149–62.
Habib BA, Sayed S, Elsayed GM. Enhanced transdermal delivery of ondansetron using nanovesicular systems: fabrication, characterization, optimization and ex-vivo permeation study-Box-Cox transformation practical example. Eur J Pharm Sci. 2018;115:352–61.
Kateh Shamshiri M, Momtazi-Borojeni AA, Khodabandeh Shahraky M, Rahimi F. Lecithin soybean phospholipid nano-TFS as potential carriers for transdermal delivery of the human growth hormone. J Cell Biochem. 2019;120(6):9023–33.
Mura P, Faucci MT, Bramanti G, Corti P. Evaluation of transcutol as a clonazepam transdermal permeation enhancer from hydrophilic gel formulations. Eur J Pharm Sci. 2000;9(4):365–72.
Modi CD, Bharadia PD. TFS: new dominants for transdermal drug delivery. Am J Pharm Tech Res. 2012;2(3):71–91.
Utreja P, Jain S, Tiwary AK. Localized delivery of paclitaxel using elastic liposomes: formulation development and evaluation. Drug Delivery. 2011;18(5):367–76.
Khan MA, Pandit J, Sultana Y, Sultana S, Ali A, Aqil M, et al. Novel carbopol-based transfersomal gel of 5-fluorouracil for skin cancer treatment: in vitro characterization and in vivo study. Drug Delivery. 2015;22(6):795–802.
Walve JR, Bakliwal SR, Rane BR, Pawar SP. TFS: A surrogated carrier for transdermal drug delivery system. 2011.
Chen J, Lu W-L, Gu W, Lu S-S, Chen Z-P, Cai B-C. Skin permeation behavior of elastic liposomes: role of formulation ingredients. Expert Opin Drug Deliv. 2013;10(6):845–56.
Sachan R, Bajpai M. Transdermal drug delivery system: a review. International Journal of Research and Development in Pharmacy & Life Sciences. 2013;3(1):773–90.
Van Tran V, Moon J-Y, Lee Y-C. Liposomes for delivery of antioxidants in cosmeceuticals: Challenges and development strategies. J Control Release. 2019;300:114–40.
Alvi IA, Madan J, Kaushik D, Sardana S, Pandey RS, Ali A. Comparative study of TFS, liposomes, and niosomes for topical delivery of 5-fluorouracil to skin cancer cells: preparation, characterization, in-vitro release, and cytotoxicity analysis. Anticancer Drugs. 2011;22(8):774–82.
Sudhakar CK, Jain S, Charyulu RN. A comparison study of liposomes, TFS and ethosomes bearing lamivudine. Int J Pharm Sci Res. 2016;7(10):4214.
Duangjit S, Obata Y, Sano H, Onuki Y, Opanasopit P, Ngawhirunpat T, et al. Comparative study of novel ultradeformable liposomes: menthosomes, TFS and liposomes for enhancing skin permeation of meloxicam. Biological and pharmaceutical bulletin. 2014:b13–00576.
Cevc G, Schätzlein AG, Richardsen H, Vierl U. Overcoming semipermeable barriers, such as the skin, with ultradeformable mixed lipid vesicles, TFS, liposomes, or mixed lipid micelles. Langmuir. 2003;19(26):10753–63.
Hao Y, Li W, Zhou X, Yang F, Qian Z. Microneedles-based transdermal drug delivery systems: a review. J Biomed Nanotechnol. 2017;13(12):1581–97.
Waghule T, Singhvi G, Dubey SK, Pandey MM, Gupta G, Singh M, et al. Microneedles: A smart approach and increasing potential for transdermal drug delivery system. Biomed Pharmacother. 2019;109:1249–58.
Bahram M, Mohseni N, Moghtader M. An introduction to hydrogels and some recent applications. Emerging concepts in analysis and applications of hydrogels: IntechOpen; 2016.
Narayanaswamy R, Torchilin VP. Hydrogels and their applications in targeted drug delivery. Molecules. 2019;24(3):603.
Gaballa SA, El Garhy OH, Abdelkader H. Cubosomes: composition, preparation, and drug delivery applications. Journal of advanced Biomedical and Pharmaceutical Sciences. 2020;3(1):1–9.
Rattanapak T, Young K, Rades T, Hook S. Comparative study of liposomes, TFS, ethosomes and cubosomes for transcutaneous immunisation: characterisation and in vitro skin penetration. J Pharm Pharmacol. 2012;64(11):1560–9.
Barriga HMG, Holme MN, Stevens MM. Cubosomes: the next generation of smart lipid nanoparticles? Angew Chem Int Ed. 2019;58(10):2958–78.
Piumitali B, Neeraj U, Jyotivardhan J. TFS—A Nanoscience in Transdermal Drug Delivery and Its Clinical Advancements. Int J Nanosci. 2020;19(04):1950033.
Dhakar RC. TFS-a Novel Vesicular Carrier for Enhanced Transdermal Delivery of Stavudine: Development, Characterization and Performance Evaluation. Characterization and Performance Evaluation (January 28, 2021). 2021.
Ascenso A, Raposo S, Batista C, Cardoso P, Mendes T, Praça FG, et al. Development, characterization, and skin delivery studies of related ultradeformable vesicles: TFS, ethosomes, and transethosomes. Int J Nanomed. 2015;10:5837.
Garg V, Singh H, Bhatia A, Raza K, Singh SK, Singh B, et al. Systematic development of transethosomal gel system of piroxicam: formulation optimization, in vitro evaluation, and ex vivo assessment. AAPS PharmSciTech. 2017;18(1):58–71.
Shabreen MR, Sangeetha S. Ethosomes: A novel drug delivery system and their therapeutic applications-A review. Research Journal of Pharmacy and Technology. 2020;13(4):1970–8.
Kumar N, Dubey A, Mishra A, Tiwari P. Ethosomes: A Novel Approach in Transdermal Drug Delivery System. International Journal of Pharmacy & Life Sciences. 2020;11(5).
Has C, Sunthar P. A comprehensive review on recent preparation techniques of liposomes. J Liposome Res. 2020;30(4):336–65.
Abbasi H, Rahbar N, Kouchak M, Khalil Dezfuli P, Handali S. Functionalized liposomes as drug nanocarriers for active targeted cancer therapy: a systematic review. Journal of Liposome Research. 2021:1–16.
Cristiano MC, Froiio F, Spaccapelo R, Mancuso A, Nisticò SP, Udongo BP, et al. Sulforaphane-loaded ultradeformable vesicles as a potential natural nanomedicine for the treatment of skin cancer diseases. Pharmaceutics. 2020;12(1):6.
Gupta PN, Vyas SP, Vishwidyalaya HSG. TFS for vaccine delivery: a potential approach for topical immunization. Med Chem Res. 2004;13(6):414–26.
Lapinski MM, Castro-Forero A, Greiner AJ, Ofoli RY, Blanchard GJ. Comparison of liposomes formed by sonication and extrusion: rotational and translational diffusion of an embedded chromophore. Langmuir. 2007;23(23):11677–83.
Shaker S, Gardouh AR, Ghorab MM. Factors affecting liposomes particle size prepared by ethanol injection method. Research in pharmaceutical sciences. 2017;12(5):346.
Linos E, Katz KA, Colditz GA. Skin cancer—the importance of prevention. JAMA Intern Med. 2016;176(10):1435–6.
Orthaber K, Pristovnik M, Skok K, Perić B, Maver U. Skin cancer and its treatment: novel treatment approaches with emphasis on nanotechnology. Journal of nanomaterials. 2017;2017.
Yang H, Wu X, Zhou Z, Chen X, Kong M. Enhanced transdermal lymphatic delivery of doxorubicin via hyaluronic acid based TFS/microneedle complex for tumor metastasis therapy. Int J Biol Macromol. 2019;125:9–16.
Luiz MT, Viegas JSR, Abriata JP, Tofani LB, de Menezes Vaidergorn M, da Silva Emery F, et al. Docetaxel-loaded folate-modified TPGS-TFS for glioblastoma multiforme treatment. Materials Science and Engineering: C. 2021;124:112033.
Pena-Rodríguez E, Moreno MC, Blanco-Fernandez B, González J, Fernández-Campos F. Epidermal delivery of retinyl palmitate loaded TFS: Penetration and biodistribution studies. Pharmaceutics. 2020;12(2):112.
Zhang K, Xiong L, Li D-Y, Gao J-J, Liu Y-K, Ma Y-S. Preparation of Cangai oil TFS patches and its in vitro evaluation. Zhongguo Zhong yao za zhi= Zhongguo zhongyao zazhi= China journal of Chinese materia medica. 2020;45(4):854–60.
Pandit AP, Omase SB, Mute VM. A chitosan film containing quercetin-loaded TFS for treatment of secondary osteoporosis. Drug delivery and translational research. 2020:1–12.
Arora D, Khurana B, Nanda S. DoE directed optimization, development and evaluation of resveratrol loaded ultradeformable vesicular cream for topical antioxidant benefits. Drug Dev Ind Pharm. 2020;46(2):227–35.
Vasanth S, Dubey A, Ravi GS, Lewis SA, Ghate VM, El-Zahaby SA, et al. Development and investigation of vitamin C-enriched adapalene-loaded transfersome gel: a collegial approach for the treatment of acne vulgaris. AAPS PharmSciTech. 2020;21(2):1–17.
Dar MJ, McElroy CA, Khan MI, Satoskar AR, Khan GM. Development and evaluation of novel miltefosine-polyphenol co-loaded second generation nano-TFS for the topical treatment of cutaneous leishmaniasis. Expert Opin Drug Deliv. 2020;17(1):97–110.
Zheng H, Xu C, Fei Y, Wang J, Yang M, Fang L, et al. Monoterpenes-containing PEGylated TFS for enhancing joint cavity drug delivery evidenced by CLSM and double-sited microdialysis. Materials Science and Engineering: C. 2020;113:110929.
Zhang J, Froelich A, Michniak-Kohn B. Topical delivery of meloxicam using liposome and microemulsion formulation approaches. Pharmaceutics. 2020;12(3):282.
Article CAS PubMed Central Google Scholar
Almehmady AM, Elsisi AM. Development, optimization, and evaluation of tamsulosin nanoTFS to enhance its permeation and bioavailability. Journal of Drug Delivery Science and Technology. 2020;57:101667.
Langasco R, Fancello S, Rassu G, Cossu M, Cavalli R, Galleri G, et al. Increasing protective activity of genistein by loading into TFS: A new potential adjuvant in the oxidative stress-related neurodegenerative diseases? Phytomedicine. 2019;52:23–31.
Waheed A, Aqil M, Ahad A, Imam SS, Moolakkadath T, Iqbal Z, et al. Improved bioavailability of raloxifene hydrochloride using limonene containing transdermal nano-sized vesicles. Journal of Drug Delivery Science and Technology. 2019;52:468–76.
Mir-Palomo S, Nácher A, Busó MAOV, Caddeo C, Manca ML, Manconi M, et al. Baicalin and berberine ultradeformable vesicles as potential adjuvant in vitiligo therapy. Colloids Surf, B. 2019;175:654–62.
Eid HM, Elkomy MH, El Menshawe SF, Salem HF. Transfersomal nanovesicles for nose-to-brain delivery of ofloxacin for better management of bacterial meningitis: Formulation, optimization by Box-Behnken design, characterization and in vivo pharmacokinetic study. Journal of Drug Delivery Science and Technology. 2019;54:101304.
Shaji J, Lal M. Preparation, optimization and evaluation of transferosomal formulation for enhanced transdermal delivery of a COX-2 inhibitor. Int J Pharm Pharm Sci. 2014;6(1):467–77.
Jain S, Jain P, Umamaheshwari RB, Jain NK. TFS—a novel vesicular carrier for enhanced transdermal delivery: development, characterization, and performance evaluation. Drug Dev Ind Pharm. 2003;29(9):1013–26.
Zeb A, Qureshi OS, Yu C-H, Akram M, Kim H-S, Kim M-S, et al. Enhanced anti-rheumatic activity of methotrexate-entrapped ultradeformable liposomal gel in adjuvant-induced arthritis rat model. Int J Pharm. 2017;525(1):92–100.
Verma N, Saraf S. Development and optimization of mannosylated naringenin loaded TFS using response surface methodology for skin carcinoma. International journal of applied pharmaceutics. 2021:235–41.
El-Feky GS, Mona M, Mahmoud AA. Flexible nano-sized lipid vesicles for the transdermal delivery of colchicine; in vitro/in vivo investigation. Journal of Drug Delivery Science and Technology. 2019;49:24–34.
Azimi M, Khodabandeh M, Deezagi A, Rahimi F. Impact of the transfersome delivered human growth hormone on the dermal fibroblast cells. Curr Pharm Biotechnol. 2019;20(14):1194–202.
Janga KY, Tatke A, Dudhipala N, Balguri SP, Ibrahim MM, Maria DN, et al. Gellan gum based sol-to-gel transforming system of natamycin TFS improves topical ocular delivery. J Pharmacol Exp Ther. 2019;370(3):814–22.
Leonyza A, Surini S. Optimization of sodium deoxycholate-based TFS for percutaneous delivery of peptides and proteins. International Journal of Applied Pharmaceutics. 2019:329–32.
Ghanbarzadeh S, Arami S. Enhanced transdermal delivery of diclofenac sodium via conventional liposomes, ethosomes, and TFS. BioMed research international. 2013;2013.
Kahlenberg JM, Fox DA. Advances in the medical treatment of rheumatoid arthritis. Hand Clin. 2011;27(1):11–20.
Kumar MS. Development of CelecoxibTransfersomal gel for the Treatment of Rheumatoid Arthritis. Indian Journal of Pharmaceutical and Biological Research. 2014;2(02):07–13.
Duangjit S, Opanasopit P, Rojanarata T, Ngawhirunpat T. Characterization and in vitro skin permeation of meloxicam-loaded liposomes versus TFS. Journal of drug delivery. 2011;2011.
Sultana SS, Sailaja AK. Preparation and evaluation of naproxen sodium loaded liposomes, ethosomes and transferosomes. J Bionanosci. 2017;11(4):284–91.
Qushawy M, Nasr A, Abd-Alhaseeb M, Swidan S. Design, optimization and characterization of a transfersomal gel using miconazole nitrate for the treatment of candida skin infections. Pharmaceutics. 2018;10(1):26.
Abdallah MH. TFS as a transdermal drug delivery system for enhancement the antifungal activity of nystatin. LIPOSOME & NANOTECHNOLOGY. 2013:390.
Paul A, Cevc G, Bachhawat BK. Transdermal immunisation with an integral membrane component, gap junction protein, by means of ultradeformable drug carriers. TFS Vaccine. 1998;16(2–3):188–95.
Download references
Acknowledgements
All the authors are very thankful to Saulat Institute of Pharmaceutical Sciences, Quaid-i-Azam University, Islamabad.
Author information
Authors and affiliations.
Department of Pharmacy, Quaid-i-Azam University, Islamabad, 45320, Pakistan
Muhammad Waseem Akram, Humzah Jamshaid, Fiza Ur Rehman, Jehan zeb Khan & Ahmad Zeb
School of Pharmaceutical Sciences of Wenzhou Medical University, Wenzhou, People’s Republic of China
Muhammad Zaeem
You can also search for this author in PubMed Google Scholar
Contributions
Muhammad Waseem Akram, Humzah Jamshaid, and Fiza Ur Rehman conceived the idea of this review study and played a lead role in project designing. Muhammad Zaeem, Jehan zeb Khan, and Ahmad Zeb extracted the data. Muhammad Waseem Akram, Humzah Jamshaid, and Jehan zeb Khan analyzed the data. Draft writing was carried out by Muhammad Waseem Akram and Humzah Jamshaid. Humzah Jamshaid and Fiza Ur Rehman reviewed the final draft.
Corresponding authors
Correspondence to Humzah Jamshaid or Fiza Ur Rehman .
Ethics declarations
Conflict of interest.
The authors declare no competing interests.
Additional information
Publisher's note.
Springer Nature remains neutral with regard to jurisdictional claims in published maps and institutional affiliations.
Rights and permissions
Reprints and permissions
About this article
Akram, M.W., Jamshaid, H., Rehman, F.U. et al. Transfersomes: a Revolutionary Nanosystem for Efficient Transdermal Drug Delivery. AAPS PharmSciTech 23 , 7 (2022). https://doi.org/10.1208/s12249-021-02166-9
Download citation
Received : 21 July 2021
Accepted : 18 October 2021
Published : 01 December 2021
DOI : https://doi.org/10.1208/s12249-021-02166-9
Share this article
Anyone you share the following link with will be able to read this content:
Sorry, a shareable link is not currently available for this article.
Provided by the Springer Nature SharedIt content-sharing initiative
- Transfersomes
- Transdermal drug delivery
- Permeation enhancer
- Cutaneous nano-drug delivery systems
- Nanotechnology
- Find a journal
- Publish with us
- Track your research
Transfersomes: A Promising Nanoencapsulation Technique for Transdermal Drug Delivery
Affiliation.
- 1 Department of Pharmaceutics and Industrial Pharmacy, Faculty of Pharmaceutical Sciences, Chulalongkorn University, Bangkok 10330, Thailand.
- PMID: 32916782
- PMCID: PMC7559928
- DOI: 10.3390/pharmaceutics12090855
Transdermal delivery systems have gained much interest in recent years owing to their advantages compared to conventional oral and parenteral delivery systems. They are noninvasive and self-administered delivery systems that can improve patient compliance and provide a controlled release of the therapeutic agents. The greatest challenge of transdermal delivery systems is the barrier function of the skin's outermost layer. Molecules with molecular weights greater than 500 Da and ionized compounds generally do not pass through the skin. Therefore, only a limited number of drugs are capable of being administered by this route. Encapsulating the drugs in transfersomes are one of the potential approaches to overcome this problem. They have a bilayered structure that facilitates the encapsulation of lipophilic and hydrophilic, as well as amphiphilic, drug with higher permeation efficiencies compared to conventional liposomes. Transfersomes are elastic in nature, which can deform and squeeze themselves as an intact vesicle through narrow pores that are significantly smaller than its size. This review aims to describe the concept of transfersomes, the mechanism of action, different methods of preparation and characterization and factors affecting the properties of transfersomes, along with their recent applications in the transdermal administration of drugs.
Keywords: nanoencapsulation; transdermal drug delivery; transfersomes.
Publication types
Grants and funding.
- CRP6105022870/Agricultural Development Agency (Public Organization); ARDA
Thank you for visiting nature.com. You are using a browser version with limited support for CSS. To obtain the best experience, we recommend you use a more up to date browser (or turn off compatibility mode in Internet Explorer). In the meantime, to ensure continued support, we are displaying the site without styles and JavaScript.
- View all journals
- My Account Login
- Explore content
- About the journal
- Publish with us
- Sign up for alerts
- Open access
- Published: 17 November 2022
Formulation, characterization of glucosamine loaded transfersomes and in vivo evaluation using papain induced arthritis model
- Muhammad Shahzad Rasheed 1 ,
- Sameen Fatima Ansari 1 &
- Iram Shahzadi 1
Scientific Reports volume 12 , Article number: 19813 ( 2022 ) Cite this article
2261 Accesses
4 Citations
Metrics details
- Medical research
- Nanoscience and technology
The aim of current study was to develop the transdermal transfersomes of glucosamine for better drug delivery. Stretch ability and plasticity of transfersomes membranes mitigate the risk of vesicle rupture in the skin and allows the drug carrying transfersome to pass through the epidermis following the natural water gradient. Transdermal delivery of Glucosamine has an advantage over oral route, having greater local concentration and fewer systemic effects. Thin Film Rotary method was use to prepare transfersomes, and characterization was carried out physio-chemically using electron microscopic studies, zeta potential evaluation, entrapment efficiency studies. To add on in the stability, development of a secondary topical vehicle using Carbopol 940 was carried out to enhance the shelf life of transfersomes. Furthermore, in vivo studies on rabbits were also carried out using the papain induced arthritis model to support the effectiveness of treatment. The radiology studies of knee joint of rabbits proved the effectiveness of glucosamine loaded transfersomes in healing the osteoarthritis with the blood plasma analysis remain unaltered. In vitro characterization showed the successful development of nano-deformable entities with good entrapment efficiency but with little stability, therefore modified into a gel. In a nut shell this modified new dosage from can be best alternative to other conventional options that owe lot of demerits.
Similar content being viewed by others
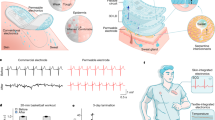
A three-dimensional liquid diode for soft, integrated permeable electronics
Binbin Zhang, Jiyu Li, … Xinge Yu
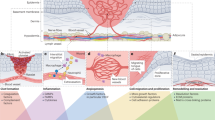
Cellular and molecular mechanisms of skin wound healing
Oscar A. Peña & Paul Martin
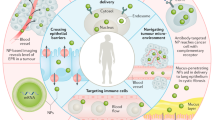
Engineering precision nanoparticles for drug delivery
Michael J. Mitchell, Margaret M. Billingsley, … Robert Langer
Introduction
Osteoarthritis a joint disease is the important cause of disability and immobilization among older peoples. Articular cartilage allow the free, friction free movement and smooth, white material that cover the end of bones, where the bones joint each other 1 . Usually 2 to 4 mm thick and is free of any nerve or blood vessel. Its composition include dense matrix containing specialized cells chondrocytes along with water, collagen, proteoglycans, and other non-collagenous proteins and glycoproteins to the lesser amount. Chondrocytes plays a vital role in the development and repair of cartilage and maintenance of matrix in which they are embedded. Chondrocytes originate from mesenchymal stem cells and making up to 2% of the total volume of articular cartilage 2 .
80% of the cartilage weighs water. Articular cartilage is separated by a synovial fluid composed of hyaluronic acid and lubricin, proteinases, and collagenases. This fluid is secreted by inner membrane of synovial joints and contains specialized cell synoviocytes.
Osteoarthritis generally involves the damage of articular cartilage and rubbing of bones ends causing pain and stiffness. The diagnosis is based on radiological visualization of joint and the cartilage integrity 3 .
Osteoarthritis can be diagnosed by three ways which are: Pathologically, Radiographically and Clinically. Most common method and simplest method if the visualization of radiological study. The visual inspection of the x-rays can give information about the any damage in the cartilage and any progression of the disease 4 .
Transfersomes are novel drug carriers or a drug delivery technology, highly deformable nature having ability to carry and transport large molecules across skin. Transfersomes are ultra-deformable nano vesicles or ultra-deformable liposomes, composed of phospholipids and edge activators such as Tween 80 or Span 80 that enable extreme flexibility, plasticity of the vesicle shape and lead to generation of elasticity 5 .
This carrier system is composed of at least one amphipathic entity (such as phospholipids like Phosphatidylcholine) which in the presence of aqueous solvents modify and adopt into lipid bilayer that closes into a simple lipid vesicle. Addition of one or a justified combination of bilayer softening components (such as a biocompatible edge activator or a surfactant or an amphiphile drug) lipid bilayer flexibility, deformability and permeability are increased 6 . The resulting nano deformable transfersome vesicles are capable to adapt its shape to requirement easily and rapidly, thus making the transfersomes different from similar morphology nano particles primarily by its flexible, easy adjustable artificial membrane 7 . Transfersomes have inner hydrophilic region for the entrapment of hydrophilic drug while hydrophobic region for water insoluble drug lying with the lipid layer.
The original composition of transfersome include phospholipids such as phosphatidylcholine or soya phosphatidyl choline and edge activators to bring elasticity 8 . Phospholipids are responsible for the formation of membrane of vesicle or transfersome. Polysorbate 80, Polysorbate 60, Span 80, Sodium Cholate etc. edge activators can be used in the formulation of transfersomes. These Edge activators are bilayer softening component, and responsible to bring flexibility and enhance permeability of vesicles 9 . Edge activators and surface charge thereof play a vital role in ensuring the stability of transfersome and achieving the desired deformability. Type and concentration greatly affect the physiochemical properties of transfersomes. Transfersomes made with cationic edge activators show better transdermal transportation in some cases and in some instances non-ionic transfersome are better transdermal drug carriers 10 .
Transfersome are drug mover system and work based upon two main factors: (a) High deformability (elasticity) of the vesicle bilayer and the (b) Osmotic gradient across the skin 11 .
Edge activator help to generate trans-epidermal osmotic gradient and squeezes into the stratum corneum, carries the drug across the skin. The difference in water content across the epidermis and stratum develops the osmotic gradient leading to ease the movement of transfersomes from higher to lower gradient and penetration of transfersomes carrying the drug across the skin 12 . The skin barriers are optimally overcome by the flexibility and deformability of the transfersomal vesicles, which can be harmonized by the relative ratio of surfactant.
The stability is assessed by the zeta potential. Zeta Potential is a measurement of the surface charge on the suspended particles. Charge on the particles generate repulsive forces and avoid particle aggregation. Particles with a zeta potential greater than ± 30 mV are considered to be stable. Stability of transfersome are usually overcome by making a secondary gel 13 . The formation of secondary gel enhances the stability and shelf life of transfersomal vesicles and also ease in application.
Glucosamine (C 6 H 13 NO 5 ) is an amino sugar and a precursor in the biochemical synthesis of glycosylated proteins and lipids. Glucosamine is part of the structure of the polysaccharides, chitosan, and chitin. Glucosamine is one of the most abundant monosaccharides. It is produced commercially by the hydrolysis of crustacean exoskeletons or, less commonly, by fermentation of a grain such as corn or wheat 14 .
Glucosamine sulphate is a precursor for glycosaminoglycans and glycosaminoglycans are a major component of cartilage, research has focused on the potential for supplemental glucosamine to beneficially influence cartilage structure and alleviate arthritis.
Studies have been conducted to evaluate the effectiveness and usefulness of glucosamine in treatment of Osteoarthritis, and many researchers in the past showed that it is equally or even better effective than NSAIDs, although its onset of action is slower. It is the structural part of glycosaminoglycans that constitute the cartilage, so ones it reaches the destination, its effectiveness is of no match thus delaying the progression of the disease 15 .
Materials and methods
Chemicals and reagents.
Analytical grade Chloroform of Merck and analytical grade Methanol of Sigma Andrich were used as organic solvents for the formulation of transfersome and liposomes. Absolute Hydrochloric acid (Merck) and Sodium Hydroxide (Sigma-Aldrich, Germany) were used for the quantitative analysis of Glucosamine sulfate as per official compendia method. Triethylamine procured from Merck was used for the formulation of hydrogel. Eugenol was purchased from Sigma Scientific Pvt limited. Phosphate Buffer saline (PBS) solution having PH. 7.0 was made from PBS tablets purchased from Sigma Scientific. Distilled water was taken from pharmaceutics lab, Department of Pharmacy, Riphah International University (Islamabad, Pakistan).
For the formulation of transfersome, Phospholipid 90G was generously gifted by Lipoid Germany, Glucosamine sulfate was gifted from Polyline ChemPharma Peshawar, Polyoxyethylene (20) sorbitan monooleate (Tween 80) and sorbitan monostearate (Span 60) were procured from Merck. For the induction of osteoarthritis in knee joint of rabbits l -Cysteine and Papain were procured from Sigma Adrich Germany. All the chemicals were of pure analytical grade.
Apparatus and equipment
Rotary evaporator (Eyela), Weighing balance (Sartorius), PH meter, Hot plate multi stirrer, Pharmaceutical Refrigerator, Beakers, funnel, Titration apparatus, centrifuge machine, sonicator, test tubes.
Synthetic procedure
Preparation of glucosamine sulphate loaded transfersomes.
Glucosamine loaded Transfersomes were prepared by using thin film hydration method with minor modifications 16 . Briefly, phospholipid and surfactant in defined ratio were dissolved in 1:1 mixture of organic solvents (Chloroform: Methanol), and the resultant mixture was film dried in Rotary evaporator at temperature just above the phase transition temperature of phospholipid (i.e., 40 °C) and at reduced pressure in a tilt position to allow complete evaporation, removal of solvent leaving behind the thin film around the inner wall of flask. The thin film was allowed to dry overnight to allow complete removal of organic solvent. The Phosphate buffer saline solution was made with the help of PBS tablets. One tablet gave 100 ml of PBS solution of 7.5 pH when dissolved in distilled water. The PBS solution was divided into two portions. In one portion 7% absolute ethanol was added to produce 7%v/v PBS solution and other portion was kept as such with no addition of ethanol. The drug was dissolved in PBS and 7% ethanolic PBS solution in the concentration of 5%w/v. The drug solution was sprinkled with the help of syringe in the flask containing the thin film. The flask was rotated at 60 rpm for 1 h at 60 °C to allow the complete hydration of film. The hydration of thin film resulted in formation of Transfersomes. The resulting formulation was centrifuged at 4000 rpm to separate the unentrapped drug by removing the supernatant and remaining formulation was kept at 4 °C for further analysis.
Formulation of glucosamine loaded liposomes
Liposomes of GS were made by the conventional rotary evaporation method. Briefly phospholipid:cholesterol:surfactant was dissolved in 1:1 of organic solvents (Methanol:Chloroform), the resultant solution was evaporated in rotary evaporator under reduced pressure and temperature above phase transition temperature. The resultant dry film along the inner wall of flask was left overnight to allow complete removal of organic solvent. The film was then hydrated with 5% solution of GS in PBS solution by rotating at 60 for 1 h. The resultant solution of liposome was then bath sonicated to produce small unilaminar vesicles.
Optimization of transfersomes
2 factorial design was used to optimize the formulation variables. The independent variables were the type of surfactant used (either the Tween 80 or Span 80), the Phospholipid to Surfactant ratio and nature of PBS solution (either 7% ethanolic PBS or simple PBS solution). All the three independent variables were assessed by keeping the environmental conditions stable and constant for each formulation. The vesicle size of Transfersomes and entrapment efficacy was assessed as dependent variables.
10 different formulations were formulated as per below table (Table 1 ) and assessed for various physiochemical variables.
(a) Vesicle size, PDI and zeta analysis All the 10 formulations were characterized in term of entrapment efficacy, polydispersity index (PDI), zeta potential and mean vesicle size, PDI, zeta potential and mean vesicle size were determined by zeta sizer on request at Quaid-e-Azam University Islamabad. The 10 µl of transfersomal dispersion was diluted with distilled water 90 µl and sent for analysis in effendoff tube of 1.5 ml.
(b) Entrapment efficiency analysis Centrifugation method was used for the determination of entrapment efficacy. Briefly the prepared formulations were centrifuges at 4000 rpm for 15 min, resulting in sedimentation of vesicles leaving behind the unentrapped drug as a supernatant. The supernatant was collected and quantitatively analyzed for the glucosamine sulfate via titration method using 0.01 M sodium hydroxide as a titrant with phenolphthalein as an indicator. The appearance of light pink color was considered as an end point and 3.028 mg of Glucosamine sulfate utilized 1 ml of 0.01 M NAOH as per British pharmacopoeia. Entrapment efficiency was figured by using below formula 13 ;
where A2 = total amount of drug added, A1 = drug un entrapped or un bound drug.
(c) Surface morphology studies The formulations were also analyzed for surface morphology using Scanning electron Microscopy (CRL lab University of Peshawar, Pakistan).
(d) Deformability of glucosamine loaded transfersomes The deformability was determined using the method as reported by 13 . Briefly transfersomal dispersion was extruded through 100 nm membrane filter (Filter-Bio ® ). After extrusion, the size of vesicles was analyzed along with weight of dispersion. The deformability index was then calculated by following formula 11 :
where D = deformability index: J = weight of dispersion Rv = vesicle size after extrusion; Rp = pore size of filter.
Preparation of glucosamine loaded transfersomal gel
The prepared optimized formulation was not viscous enough to be applied trans dermally and also not stable enough to be stored for long period. That’s why the transformable dispersion was formulated as gel by using the method as reported by 13 . with minor modification. Briefly, transfersomes were added into 1% Carbopol 940 gel to make it rheological acceptable. 1 g of Carbopol 940 was added slowly with continuous stirring with machine to distilled water along with addition of transfersomal dispersion drop wise, to make volume up to 100 ml and triethanolamine was added slowly drop wise until a clear gel was formed. The PH of the resulting gel was analyzed by taking 1 g of gel and dissolving it in distilled water to make 50 ml, the triethanolamine was added until a neutral gel was obtained.
Physiochemical evaluation of glucosamine loaded transfersomal gel
The blank gel and the Glu-loaded transfersomal gel were evaluated in term of physical appearance, clarity, pH. Ph is determined by dissolving the 1-g gel in 50 ml of distilled water and measuring with the help of portable pH meter. Appearance and Clarity of gel were determined by placing the two formulations under the light of 400 lx.
Characterization of glucosamine loaded liposomes
The formed liposomal dispersion was analyzed for entrapment efficiency by centrifugation and zeta analysis by the same method as adopted for transfersomal dispersion.
Preparation of glucosamine liposomal gel
The gel was made by dissolving the vesicles in 1% w/w of Carbopol 940 and pH was made neutralized with the help of triethanolamine. And resulting gel was evaluated for pH, clarity and appearance by the same method as adopted for transfersomal gel 13 .
Physiochemical evaluation of liposomal gel
Resulting gel was evaluated for pH, clarity and appearance by the same method as adopted for transfersomal gel.
In vivo studies
Selection of animal.
04 Healthy male rabbits, age 8 months were selected for in-vivo evaluation for effectiveness of therapy using glucosamine loaded transfersomes. Digital X-rays of all 4 rabbits knee joints were carried out at Pet and Vet clinic Islamabad. Moreover, radiological studies, CBC (Complete blood count) of rabbits were also performed to keep the data as a reference.
Rabbits were marked in numeric 1 to 4 using Cardinal Health Multi Ink Marker. 1st rabbit was kept as reference (control group), with no induction of osteoarthritis and treatment thereafter. All the remaining 3 rabbits, osteoarthritis was induced using the Bentley model and verified via radiological studies. The 2nd rabbit have been treated with liposomal formulation while 3rd and 4th been undergone treatment with transfersomal formulation for about 4 weeks.
Rabbits were kept in open space, fed with cereals, green vegetables, fruits with easy access to fresh drinking water, with no restriction to movement.
Preparation of papain: cysteine solution
For induction of osteoarthritis, Bentley model was adopted. By dissolving papain powder in water for injection (WFI) and co-administered along with cysteine solution to induce osteoarthritis in knee joint of rabbits, the solution of papain was prepared. Cysteine was added to act as activator 17 . pH of the resultant solution was checked.
Induction of osteoarthritis
Osteoarthritis was induced in 3 rabbits, with the average weight of 3 kg each. The initial x-rays were carried out at Pets and vet’s clinic Islamabad. The other 3 rabbits were injected using 25G B.Braun auto disable syringe with injection volume of papain: cysteine 0.2 ml: 0.1 ml at day 1. Injection was placed into the knee joint (intra-articular into the knee joint of right leg) of un-anesthetized rabbit avoiding the damage to the cartilage of the tibial plateau. The reference group (1st Rabbit) was injected with equal volume of normal saline solution. However, the left leg was not injected to allow free movement of rabbits. The same process was repeated on day 2.
After 6 days, the x-rays were carried out and show successful induction of osteoarthritis that is also visually apparent from movement of rabbits.
Application of formulated transfersomal and liposomal gel
After osteoarthritis development, as witnessed from the radiological studies, the rabbits were treated with transdermal application of prepared gel with mild rubbing. Previously the knee joints were shaved to sallow application of gel to the skin and avoiding sticking to the hairs. All the 3 rabbits were given the treatment initially for 2 weeks and x-rays were taken every week to access the healing process. Furthermore, the movement of rabbits were also noticed to witness the effect of gel. The second radiological studies were carried about after 2 weeks along with CBC analysis at pet and vet clinic Islamabad to record the observations.
Hematology and blood glucose studies
The blood samples were taken from all the rabbits prior to treatment with gel to determine the total blood count using the Medonic Hematology analyzer and blood glucose was determined by using Roche portable glucose meter. The same parameters were examined after the treatment to check the any change in blood composition after the application of drug.
Formulation of glucosamine sulfate loaded transfersomes
Total of 10 formulations were made using thin film hydration method as per table (Table 2 ).
6 formulations made using simple PBS solution were visually milky in texture while 4 formulations made using 7% ethanolic PBS solution tend to sediment soon after the 1 h cake formation occurred in formulations made using Span 80 as a surfactant, converting to homogeneous dispersion on shaking.
Thin film was successfully generated in all the cases and left overnight to allow complete remove of organic solvents, then hydrated with respective hydration medium while continuous stirring on the rotary evaporator.
Entrapment efficiency
Entrapment efficiency of all the 10 formulations were recorded using the centrifugation method followed by titration method as per British Pharmacopeia shown in Table 3 . Standard calibration curve of Glucosamine Sulphate was established by plotting concentration on x-axis vs volume used on y-axis as represented in Fig. 1 . According to British Pharmacopeia, 3.028 mg uses 01 ml of 0.01 molar NaOH.
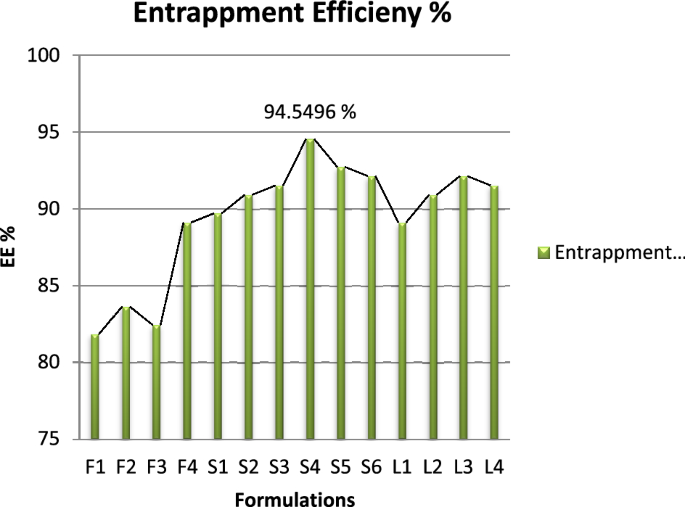
Graphical representation of EE results.
All the formulations were centrifuged at 4000 rpm for 15 min and supernatant was collected and analyzed for unentrapped drug.
Vesicle size, PDI and zeta potential
PDI, zeta potential and mean vehicle size shown in Fig. 2 were get analyzed from Quaid-i-Azam university Islamabad tabulated below Table 4 . The sample after dissolution were sent for analysis. PDI and zeta potential of Glu-Loaded formulations are displayed in Figs. 3 and 4 respectively. The following results were obtained:
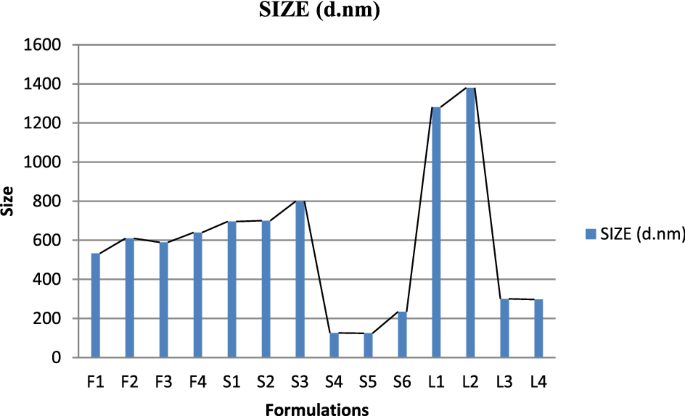
Graphical representation of vesicle size of Glu-loaded formulations.
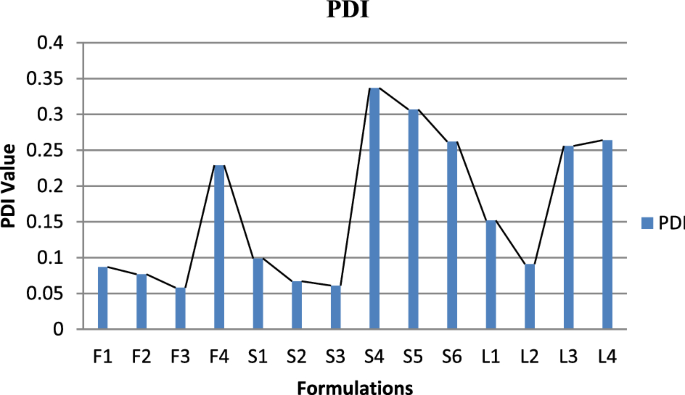
Graphical representation of PDI of Glu-loaded formulations.
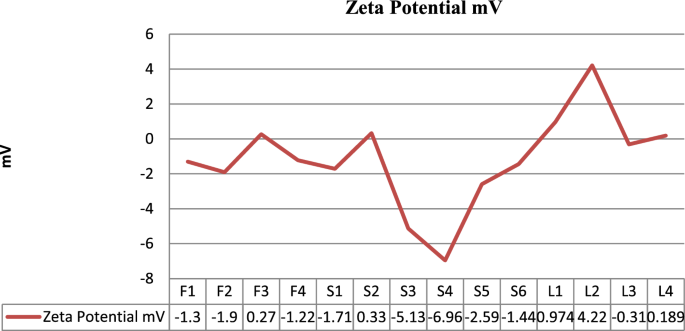
Graphical representation of zeta potential of Glu-loaded formulations.
SEM analysis (surface morphology study)
Morphology of transfersomes was observed as shown in Fig. 5 using scanning electron microscope 18 to determine the shape and lamellarity of transfersomes. Figure 6 shows Microscopic Evaluation of optimized Glu-Loaded Transfersome (S2 formulation) The following results were obtained:
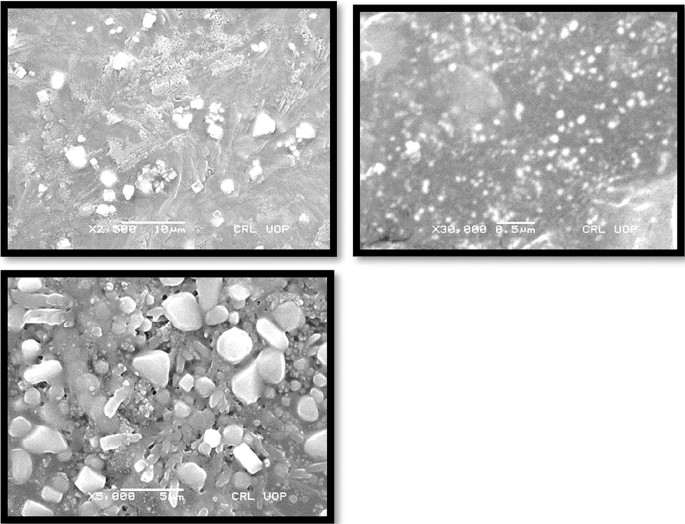
Microscopic evaluation of optimized Glu-loaded transfersome (S4 formulation).
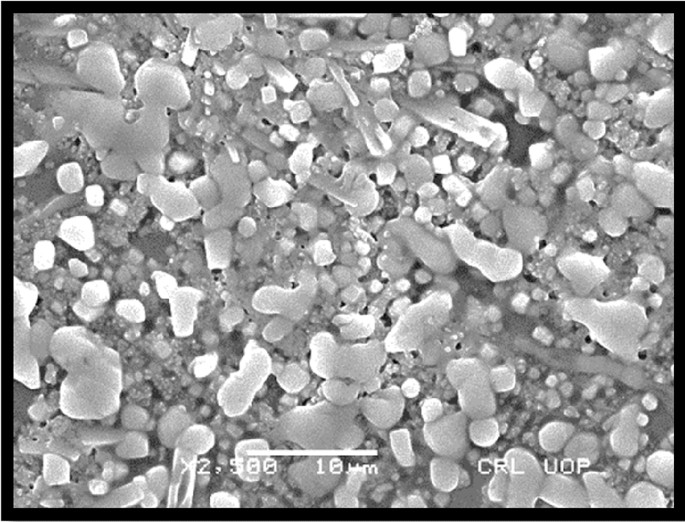
Microscopic evaluation of optimized Glu-loaded transfersome (S2 formulation).
Deformability of glucosamine loaded transfersomes
DI (deformability index) of optimized formulation was calculated and tabulated in Table 5 using extrusion method, the following observations were observed:
Formulation of transfersomal gel
The optimized transfersomes were not viscous enough to stay on application site for a reasonable time. Therefore, the transfersomes were modified into gel as per method explained above. The pH was adjusted with triethanolamine to the neutral as presented in Table 6 .
Physiochemical evaluation of gel
In vivo osteoarthritis healing capacity of the transfersomal gel.
4 rabbits were used in the test, initial radiological x-rays of both the knee joints were taken using Siemens Hybrid Digital X-ray machine and deformities are depicted in Fig. 7 .
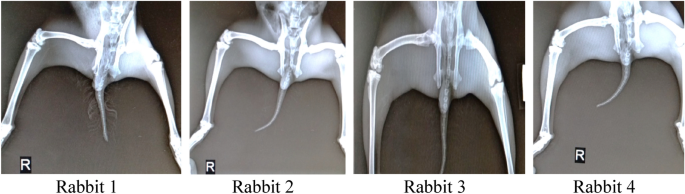
Initial x-rays of rabbits prior to induction of osteoarthritis.
In addition to radiological study, CBC (complete blood count) using the Medonic Hematology Analyzer was also carried out along the blood sugar level detection using AccuChek Glucometer.
The following observations were found and expressed in Table 7 :
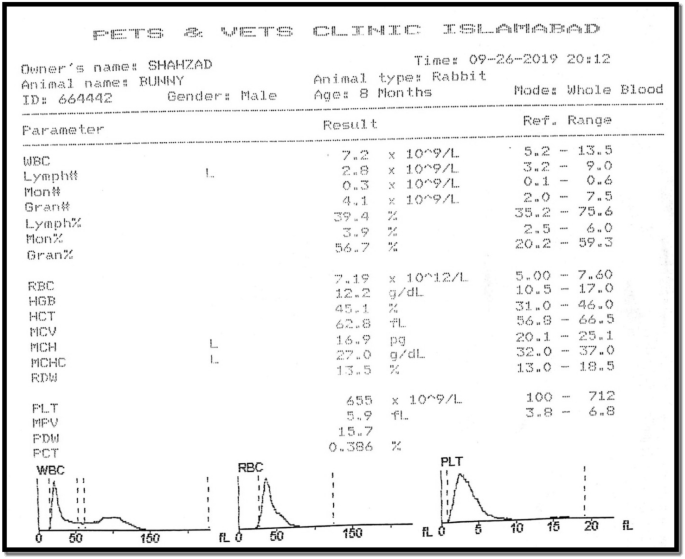
Blood glucose level was observed as follow:
4% solution of Papain and 0.03 M solution of Cysteine was prepared as per method described above and injected into right knee joint of 2nd, 3rd and 4th rabbit.
Radiological and hematological results
The hematology studies shown in Fig. 8 were again carried out the application of drug for 1 month and upon good movement response by the rabbits except the one treated with liposomal gel. The following findings were observed in Table 8 :
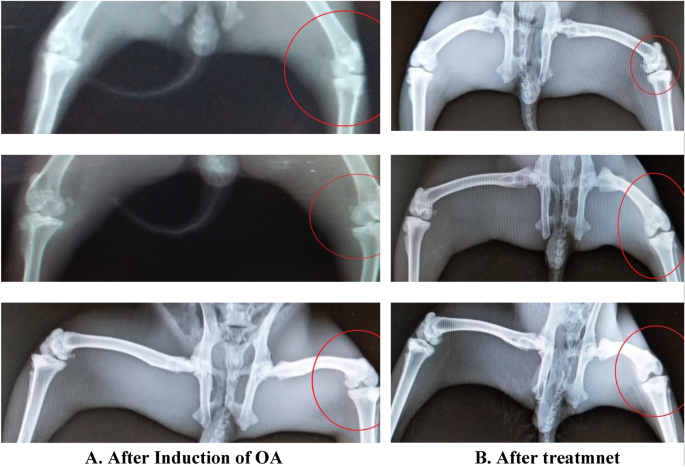
Radiological study results and observation.
Osteoarthritis is a common disease of joints and it is affecting a large number of people worldwide. Osteoarthritis is characterized by progressive destruction of cartilage. Cartilage is smooth elastic tissue, composed of specialized cell called chondrocytes. Cartilage protects the ends of joints and provide a cushion for free movement of bones against each other. In disease condition, this cartilage gets damaged because of many reasons thereby exposing the underneath bone. The exposed bones rubbed against each other and generate pain, inflammation, difficulty in movement. Apparently, the OA is not diagnosable but predictable form symptoms feel by patient like the pain, swollen joint, difficulty in movement etc. Radiological studies help to diagnose the prevalence of disease. Various treatment includes exercise and use of pain killer to relieve the pain. Different Physiotherapy techniques also help to reduce the severity but not bringing the betterment in the integrity of damaged cartilage.
Cartilage is devoid of the access of blood vessels therefore restricting the bioavailibity of precursor’s molecules at the site of action. Treatment include the injection of various molecules with proven efficacy and safety in regeneration of cartilage. Studies have investigated the proven role of Glucosamine sulphate in the treatment of osteoarthritis as it is principal precursor of glycosaminoglycan, a major component of cartilage. But glucosamine sulphate has low bioavailability, also the synovial joint is avesicular and without any blood supply, thus limiting the effectiveness of traditional usage of glucosamine sulphate. Additionally, there have not yet been any studies focused on the development of Glucosamine sulphate transfersome. Therefore, we try to encapsulate the glucosamine sulphate into transfersome, which may have potential application for healing the degenerative disease of osteoarthritis.
Transfersomes are highly elastic and ultra-deformable vesicles, very similar to liposomes and act as carrier for transportation of various molecules. Due to elastic nature, they can easily cross the skin carrier and reach the site of action. So, transfersomes are selected to get incorporated with Glucosamine sulphate, to allow painless and easy availability of salt at the site of action to initiate the process of cartilage regeneration. Glucosamine sulphate is an amino sugar with molar mass of 179.17 g/mol and supplemental glucosamine may help to rebuild cartilage and treat arthritis.
In this study, we successfully prepared transfersome using thin film hydration method. This method of preparation was chosen keeping in view the availability of required apparatus/equipment (Rotary Evaporator) and it is the most effective method of formulating transfersomes in which thin film is prepared hydrated and then brought to the desired size by sonication. All the preparation was carried out at controlled lab environmental conditions. Concluding the tested parameters, the best production condition was operating the rotary evaporator at the water bath temperature of 45 degree Celsius with 250 ml round bottom rotary flask at the angle 45 degree with the RPM of 60. Operating the apparatus at less than 60 RPM and below temperature of 45 degree Celsius resulted in gel like mass at the center of rotary flask, that is the mixture of phospholipid and surfactant due to poor evaporation of organic solvent.
The optimized formulation was 5% drug loaded in 90:10 ratio of Phospholipid: Tween 80 with hydration with PBS solution. Use of Ethanolic PBS solution didn’t result in satisfactory production of transfersome and resulted in sedimentation of vesicles. This might be due to rupturing of vesicles and non-entrapment of drug which settle at the bottom on the tube. Furthermore, these conditions were also used to compare the other edge activator i.e., Span 80.
Span 80 was also used to formulate different test formulation of glucosamine loaded transfersomes. The formulations made with Span-80 trend to be larger in vesicle size (with average of 750 nm) and also lead to creaming i.e., the formed vesicles get accumulated at the top of tube leaving below a clear liquid layer. According to the evaluation of particle size, entrapment efficiency and zeta potential, the optimal particle size of S4 formulation was 126 nm with entrapment efficiency 94.54% calculated using official compendial method. However, the zeta potential was not significantly good enough to claim the stable production of transfersomes. Therefore, the vesicles were entrapped in secondary gel of Carbopol 940. The formation of gel resulted in stable formulation with clear appearance, neutral pH and better viscosity to allow better application of skin. The transfersomal formulation was added drop wise near the shaft of mixer, avoiding the direct contact with shaft and dropping from too much height.
Scanning Electron Microscopy suggested the morphology of transfersome having pretty satisfactory shape and size to allow the penetration of transfersome across the skin barrier. The deformability index of optimized formulation was found good having the value of 10–12 g. The availability of drug at the site of action is the most desirable attribute of this study that was assessed by analyzing the radiological studies and blood glucose level of rabbits. Male healthy albino rabbits of 8 months age and approximately 3 kg weight were used in the study, feed on natural diet and without restricted movement.
In the in vivo analysis, osteoarthritis was first induced in the 3 rabbits following the Bentley Model and development of osteoarthritis was confirmed from radiological studies. The induction of osteoarthritis was also clearly visible from movements of rabbits. The rabbits with injections of papain: cysteine have less movement compared to the reference rabbit and they also fold the leg with injection to cop pain and osteoarthritis. After the induction of osteoarthritis, the effectiveness of prepared formulations was analyzed. The formulated drug in gel form was applied with mild rubbing and rabbits are left free to move. After one month of regular application of drug formulations, the 2 rabbits showed better regeneration of cartilage without any significant changes in the CBC (Complete blood count). However, the test animal treated with liposomal formulation failed to show any improvement. The treatment was kept continue for one month with periodic checks on radiological and hematological results. One of the rabbits is also treated with Liposomal formulation, didn’t show any improvement in osteoarthritis. That might be due to non-penetrability of liposomal formulation across the skin barrier.
Therefore, this result also indicated that the application of transfersome in the future might improve the bioavailability in many respects however the stability of the transfersomal formulation is an issue that can be overcome using the combination of different edge activators.
Transfersomes are proven the better drug delivery through the management and treatment of osteoarthritis. Glucosamine sulphate have a vital role in the treatment of osteoarthritis but effectiveness is restricted due to un-availability of drug at the site of action. Glucosamine sulphate is the principal precursor of glycosaminoglycan, which is the major component of cartilage.
Nano deformable transfersomes of Glucosamine sulphate were successfully formulated, optimized, physiochemically characterized and in vivo evaluated to support the ability of transfersomes in treatment and management of osteoarthritis. Transfersomal gel was formulated to increase the stability of vesicles carrying the drug and for easily application on skin.
The results obtained as per radiological study of rabbit’s knee joint strongly support the effectiveness and usefulness of transfersomes for the transdermal delivery of drug. The study helps to develop similar formulations for the management and treatment of various diseases, where the bioavailability of drug at target site is the issue.
The only limitations associated with this study is the shelf life of the prepared formulations which is not assessed for final gel formulation. By changing various parameters, it might be possible to obtain more stable formulation and real time assessment of stability study can bring further improvement in the study.
Data availability
All data relevant to the study are included in the article or no data is uploaded as supplementary information.
Glyn-Jones, S. et al. Osteoarthritis. Lancet 386 (9991), 376–387 (2015).
Article CAS PubMed Google Scholar
Poole, A. R. et al. Composition and structure of articular cartilage: A template for tissue repair. Clin. Orthop. Relat. Res. 391 , S26–S33 (2001).
Article Google Scholar
Martel-Pelletier, J. Pathophysiology of osteoarthritis. Osteoarthr. Cartil. 7 (4), 371–373 (1999).
Article CAS Google Scholar
Pogrund, H. et al. Osteoarthritis of the hip joint and osteoporosis: A radiological study in a random population sample in Jerusalem. Clin. Orthop. Relat. Res. 164 , 130–135 (1982).
González-Rodríguez, M. et al. Deformability properties of timolol-loaded transfersomes based on the extrusion mechanism. Statistical optimization of the process. Drug Dev. Ind. Pharm. 42 (10), 1683–1694 (2016).
Article PubMed Google Scholar
Karunanidhi, P. & Singh, S. A review on skin targeted delivery of bioactives as ultradeformable vesicles: Overcoming the penetration problem. Curr. Drug Targets 15 , 184–198 (2014).
Rai, S., Pandey, V. & Rai, G. Transfersomes as versatile and flexible nano-vesicular carriers in skin cancer therapy: The state of the art. Nano Rev. Exp. 8 (1), 1325708 (2017).
Article PubMed PubMed Central Google Scholar
Celia, C. et al. Ethosomes ® and transfersomes ® containing linoleic acid: Physicochemical and technological features of topical drug delivery carriers for the potential treatment of melasma disorders. Biomed. Microdevice 14 (1), 119–130 (2012).
Mamdouh, G. et al. Role of edge activators and surface charge in developing ultradeformable vesicles with enhanced skin delivery. Int. J. Pharm. 397 , 164–172 (2010).
Duangjit, S. et al. Evaluation of meloxicam-loaded cationic transfersomes as transdermal drug delivery carriers. AAPS PharmSciTech 14 (1), 133–140 (2013).
Jain, S. et al. Transfersomes—a novel vesicular carrier for enhanced transdermal delivery: Development, characterization, and performance evaluation. Drug Dev. Ind. Pharm. 29 (9), 1013–1026 (2003).
Benson, H. A. E. Transfersomes for transdermal drug delivery. Expert Opin. Drug Deliv. 3 (6), 727–737 (2006).
Dar, M. J., Din, F. U. & Khan, G. M. Sodium stibogluconate loaded nano-deformable liposomes for topical treatment of leishmaniasis: Macrophage as a target cell. Drug Deliv. 25 (1), 1595–1606 (2018).
Article CAS PubMed PubMed Central Google Scholar
Barclay, T. S., Tsourounis, C. & McCart, G. M. Glucosamine. Ann. Pharmacother. 32 (5), 574–579 (1998).
Towheed, T. et al. Glucosamine therapy for treating osteoarthritis. Cochrane Database Syst. Rev. (2) (2005).
Ahad, A. et al. Formulation and characterization of Phospholipon 90 G and tween 80 based transfersomes for transdermal delivery of eprosartan mesylate. Pharm. Dev. Technol. 23 (8), 787–793 (2018).
Bentley, G. Papain-induced degenerative arthritis of the hip in rabbits. J. Bone Joint Surg. Br. Vol. 53 (2), 324–337 (1971).
El-Alim, S. H. A. et al. Comparative study of liposomes, ethosomes and transfersomes as carriers for enhancing the transdermal delivery of diflunisal: In vitro and in vivo evaluation. Int. J. Pharm. 563 , 293–303 (2019).
Download references
Acknowledgements
The authors are thankful to Lipoid Germany and Polyfine ChemPharma Peshawar, Pakistan for providing Phospholipon 90G and Glucosamine sulfate as gift samples.
Author information
Authors and affiliations.
Riphah Institute of Pharmaceutical Sciences, Riphah International University, 7th Avenue, Sector G-7/4, Islamabad, Pakistan
Muhammad Shahzad Rasheed, Sameen Fatima Ansari & Iram Shahzadi
You can also search for this author in PubMed Google Scholar
Contributions
All authors contributed to the study conception and design. The experimental work and analysis were performed by M.S.R. The first draft of the manuscript was written by M.S.R. All authors read and approved the final manuscript.
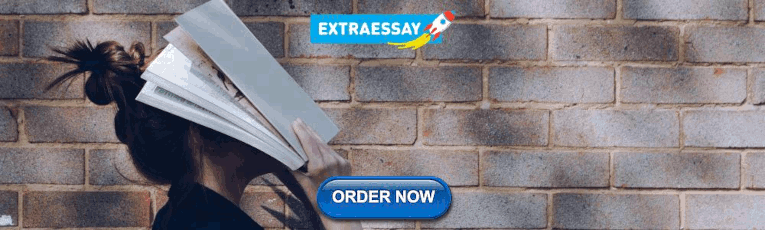
Corresponding author
Correspondence to Sameen Fatima Ansari .
Ethics declarations
Competing interests.
The authors declare no competing interests.
Additional information
Publisher's note.
Springer Nature remains neutral with regard to jurisdictional claims in published maps and institutional affiliations.
Rights and permissions
Open Access This article is licensed under a Creative Commons Attribution 4.0 International License, which permits use, sharing, adaptation, distribution and reproduction in any medium or format, as long as you give appropriate credit to the original author(s) and the source, provide a link to the Creative Commons licence, and indicate if changes were made. The images or other third party material in this article are included in the article's Creative Commons licence, unless indicated otherwise in a credit line to the material. If material is not included in the article's Creative Commons licence and your intended use is not permitted by statutory regulation or exceeds the permitted use, you will need to obtain permission directly from the copyright holder. To view a copy of this licence, visit http://creativecommons.org/licenses/by/4.0/ .
Reprints and permissions
About this article
Cite this article.
Rasheed, M.S., Ansari, S.F. & Shahzadi, I. Formulation, characterization of glucosamine loaded transfersomes and in vivo evaluation using papain induced arthritis model. Sci Rep 12 , 19813 (2022). https://doi.org/10.1038/s41598-022-23103-1
Download citation
Received : 28 April 2022
Accepted : 25 October 2022
Published : 17 November 2022
DOI : https://doi.org/10.1038/s41598-022-23103-1
Share this article
Anyone you share the following link with will be able to read this content:
Sorry, a shareable link is not currently available for this article.
Provided by the Springer Nature SharedIt content-sharing initiative
This article is cited by
Transfersome, an ultra-deformable lipid-based drug nanocarrier: an updated review with therapeutic applications.
- Abdul Hafeez
- Mishkaat Parveen Izhar
Naunyn-Schmiedeberg's Archives of Pharmacology (2024)
By submitting a comment you agree to abide by our Terms and Community Guidelines . If you find something abusive or that does not comply with our terms or guidelines please flag it as inappropriate.
Quick links
- Explore articles by subject
- Guide to authors
- Editorial policies
Sign up for the Nature Briefing newsletter — what matters in science, free to your inbox daily.

Journal of Pharmaceutical Research International

Published: 2021-10-06
DOI: 10.9734/jpri/2021/v33i45B32801
Page: 241-254
Issue: 2021 - Volume 33 [Issue 45B]
Review Article
Transferosomes as a Novel Therapeutic Delivery System: A Review
Risvana Iqubal
Department of Pharmaceutics, National College of Pharmacy, Manassery, Mukkam Post, Kozhikode, Kerala-673602, India.
Vimal Mathew *
Vinayaka Mission College of Pharmacy, Yercaud, Ghat Road, Kondappanaickenpatti, Salem, Tamil Nadu -636008, India.
Najiya Nasri K. V.
Safeetha Shamsudheen
Umamaheswari D.
*Author to whom correspondence should be addressed.
The poor penetration rate of the skin as a natural barrier makes transdermal drug delivery problematic. To increase transdermal dispersion of bioactives, electrophoresis, iontophoresis, chemical permeation enhancers, microneedles, sonophoresis, and vesicular systems such as liposomes, niosomes, elastic liposomes such as ethosomes, and transferosomes have all been used. Among these, transferosomes appear to be a promising option. Transferosomes are elastomeric or deformable vesicles that were originally discovered in the early 1990s. They're novel vesicular drug carrier system composed of phospholipid, surfactant, and water that improves transdermal drug delivery. Because of their low toxicity, biodegradability, ability to encapsulate both hydrophilic and lipophilic molecules, ability to prolong the drug's existence in the systemic circulation by encapsulation in vesicles, ability to target organs and tissues, and ability to reduce drug toxicity while increasing bioavailability, these vesicles are preferred over others. These vesicles undergo deformation, changes its shape and easily penetrates through the skin pores. There are two phases in any technique for preparing transferosomes. First, a thin film is hydrated before being sonicated to the required size; next, sonicated vesicles are homogenized by extrusion through a polycarbonate membrane. Transferosomes are evaluated for its entrapment efficiency, their drug content , in-vitro drug release, degree of deformability, turbidity, surface charge and morphology. Transferosomes are said to have a number of applications like delivery of vaccines,proteins, Anti-cancer drugs,anesthetics,herbal drugs and has better patient compliance,improved bio-availability and site-specific delivery and can serve as an emerging tool for transdermal delivery of almost all drugs and bio-actives.
Keywords: Transferosomes, deformable, transdermal delivery, bioavailability
How to Cite
- Download Citation
- Endnote/Zotero/Mendeley (RIS)
© Copyright 2010-Till Date, Journal of Pharmaceutical Research International. All rights reserved.
- Open access
- Published: 09 April 2024
Liposomes, transfersomes and niosomes: production methods and their applications in the vaccinal field
- Domenico Riccardi 1 ,
- Lucia Baldino ORCID: orcid.org/0000-0001-7015-0803 1 &
- Ernesto Reverchon 1
Journal of Translational Medicine volume 22 , Article number: 339 ( 2024 ) Cite this article
314 Accesses
1 Altmetric
Metrics details
One of the most effective strategies to fight viruses and handle health diseases is vaccination. Recent studies and current applications are moving on antigen, DNA and RNA-based vaccines to overcome the limitations related to the conventional vaccination strategies, such as low safety, necessity of multiple injection, and side effects. However, due to the instability of pristine antigen, RNA and DNA molecules, the use of nanocarriers is required. Among the different nanocarriers proposed for vaccinal applications, three types of nanovesicles were selected and analysed in this review: liposomes, transfersomes and niosomes. PubMed, Scopus and Google Scholar databases were used for searching recent papers on the most frequently used conventional and innovative methods of production of these nanovesicles. Weaknesses and limitations of conventional methods (i.e., multiple post-processing, solvent residue, batch-mode processes) can be overcome using innovative methods, in particular, the ones assisted by supercritical carbon dioxide. SuperSomes process emerged as a promising production technique of solvent-free nanovesicles, since it can be easily scaled-up, works in continuous-mode, and does not require further post-processing steps to obtain the desired products. As a result of the literature analysis, supercritical carbon dioxide assisted methods attracted a lot of interest for nanovesicles production in the vaccinal field. However, despite their numerous advantages, supercritical processes require further studies for the production of liposomes, transfersomes and niosomes with the aim of reaching well-defined technologies suitable for industrial applications and mass production of vaccines.
Introduction
Vaccination is one of the most effective and cheap strategies to handle health diseases and fight viruses. Different studies state that vaccines prevent every year more than 2.5 million deaths worldwide [ 1 ]. Recently, the vaccination strategy has been widely used to avoid hospitalization, severe symptoms and death caused by the SARS-CoV-2 virus, showing very high effectiveness and giving the possibility to overcome a world pandemic [ 2 ].
Vaccines are now sparking more interest, and new types and strategies of vaccines are going to be developed and used for different medical issues, such as autoimmune disorders and certain types of cancer [ 3 ].
De Gregorio et al. [ 1 ] reported that the first witness of immunity from a disease can be found in Thucydides’ “The History of the Peloponnesian War”, in which the plague that was affecting Athens is described, stating that “the disease was not able to infect twice the same subject”. Until 1796, the only way to spread immunity, in particular for the smallpox disease, was the variolation, which consisted of inoculating materials taken from an infected patient, inducing a mild form of the disease and, thus, preventing the person from being re-infected. This technique was not safe because the risk of contracting the disease in a fatal way was possible [ 4 ]. Edward Jenner, in 1796, used pustules from cowpox to induce smallpox immunity, having the same results of variolation; but with much less severe side effects [ 5 ]. This event is set as the birth of the first vaccine, opening the way to a new approach for their development. Subsequently, vaccines were based on weakening and attenuating viruses before inoculating them into the patient using oxygen or heat, as first performed by Louis Pasteur in 1880 for chicken cholera and anthrax [ 6 , 7 ]. Vaccines for diphtheria and tetanus were developed in 1923, when Alexander Glenny and Barbara Hopkins used formaldehyde to inactivate bacterial toxins [ 8 ]. Polysaccharides vaccines were introduced in 1960 for meningococcal prevention [ 9 ].
However, the introduction of new technologies became significantly important for the development of safer and more effective vaccines. Indeed, though the first phase of research on vaccine was useful for the development of conventional vaccines formed by inactivated microbes that were able to induce an immune response, multiple injections were needed and they tended to have a poor safety record [ 10 ]. To overcome these disadvantages, novel formulations of vaccine have been developed. Subunit vaccines do not contain the whole pathogen; but only the antigenic part of the pathogens, such as proteins, polysaccharides or peptides. Moreover, these types of vaccines present numerous advantages, such as application to immunocompromised patients and less chances of causing side effects [ 11 ]. On the other hand, they present limitations like reduced immunogenic action than the conventional vaccines and the necessity to add adjuvants to the formulation, that are substances that can enhance the immune response [ 12 , 13 ]. One of the ways to overcome this problem is to encapsulate antigens in nanometric particles that can also protect them from degradation [ 14 ].
The necessity of using nanometric particles as nanocarriers is also found for DNA and RNA-based vaccines due to their fast degradation when used in their ‘naked’ form. For this reason, advanced delivery vesicles, like liposomes, transfersomes and niosomes, have been proposed and used [ 15 ], to protect these macromolecules from degradation before and after cell transfections [ 16 , 17 ].
Nanovesicles, like liposomes, transfersomes and niosomes show an adjuvant ability, enhancing the immune response to antigens encapsulated in their aqueous core [ 14 , 18 ]. RNA-based vaccines, especially mRNA-based vaccines, have been recently developed and approved by the FDA to fight the COVID-19 pandemic [ 19 ]. mRNA in a cell can be translated by ribosomes in the cytoplasm forming the required protein (this process is called protein synthesis). In particular, for vaccine utilization, mRNA is synthesized to transport the information of only the antigen production; i.e., mRNA is recognised by ribosomes, the message is decrypted, and antigens are produced [ 20 ]. Antigens are exposed on the surface of APC cells (antigen-presenting cells, like dendritic cells) and, consequently, lymphocytes T cells, called helpers, induce the immunity response recognising the antigen as a threat to the organism, stimulating the formation of cytotoxic T lymphocytes that are able to kill the infected cells, and the formation of B lymphocytes cells that produce specific antibodies; B lymphocytes can evolve in memory B cells, which are able to preserve the capacity of recognise the virus, even after years from the first infection/vaccination, in order to re-activate in a fast and more efficiently way the specific immune response in case of infection [ 21 ].
Lipid-based nanovesicles are internalized by APCs throughout phagocytosis (Fig. 1 ) or receptor-mediated endocytosis: in particular, carriers smaller than 150 nm are internalized by endocytosis, whereas vesicles bigger than 150 nm are taken up by APCs through phagocytosis [ 22 ].
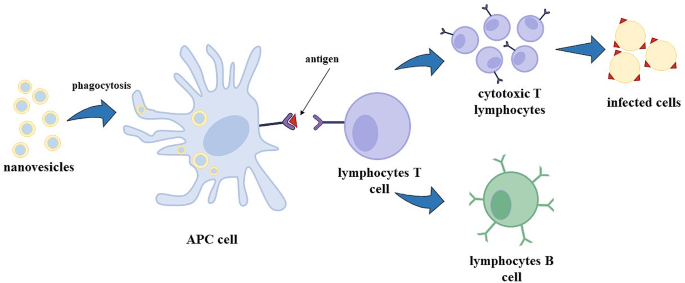
Mechanism of immune response
Therefore, this review is focused on the application of these nanovesicles in the vaccinal field, and the advantages/disadvantages of their main production techniques are critically discussed in view of the requirements of the industrial scale-up.
Typesof nanovesicles
Liposomes are vesicles characterized by an aqueous core surrounded by a double layer of phospholipids that are amphiphilic compounds with a hydrophilic head and hydrophobic tails [ 23 ]. Due to the amphipathic nature of the phospholipids, they can spontaneously rearrange their structure into a spherical geometry, in an aqueous environment (Fig. 2 ); moreover, they are able to carry both hydrophilic compounds in the inner core, and lipophilic compounds in the external double-layer [ 24 ]. Owing to this property, they are used, among the others, to deliver antioxidants, anti-inflammatory, antimicrobial and anticancer compounds [ 25 ].
Liposomes structure can also be modified to obtain transfersomes: they are known as ultra-deformable liposomes because of their flexible membrane that allows them to deform and penetrate the skin in an effective way [ 26 ]. Transfersomes can be obtained by adding to the lipidic layer compounds called penetration enhancers or edge activators that are surfactants, such as sodium deoxycholate, span and tween [ 27 ] (Fig. 2 ). These edge activators produce a discontinuity in the nanovesicle membrane allowing the deformation of the transfersomes. The advantage of using transfersomes is the easierway of drug administration throughout the skin; they have indeed the ability to permeate the skin and can encapsulate different types of compounds like small molecules, proteins, antioxidants, peptides and vaccines [ 28 ].
Niosomes are nanovesicles formed by non-ionic surfactants. They are similar to liposomes in terms of physical properties (Fig. 2 ); but, they show a longer physical-chemical stability (even at room conditions), lower toxicity because of their non-ionic nature, are biodegradable and non-immunogenic [ 29 ]. They have been used to encapsulate various compounds, such as chemotherapeutic drugs, antibiotics, antioxidants and vaccines [ 30 , 31 ].
According to the kind of phospholipids used, liposomes, transfersomes and niosomes can show a negatively or positively surface charge; generally cationic liposomes are preferred for vaccine delivery, because APCs are negatively charged, and the electrostatic interactions can lead to a better cellular adhesion of nanovesicles to APCs, resulting in an accelerated phagocytosis [ 32 , 33 , 34 ]. For this purpose, different cationic phospholipids are used such as N-[1-(2,3-dioleoyloxy) propyl]-N, N,N-triethylammonium chloride (DOTMA), 1,2-dioleoyl-sn-glycerol-3-phosphoethanolamine (DOPE), N1, N1-dimyristeroyloxyethyl-spermine, 1,2-dioleoyl-3-trimethylammonium-propane (DOTAP) and others that will be mentioned in the subsequent sections.
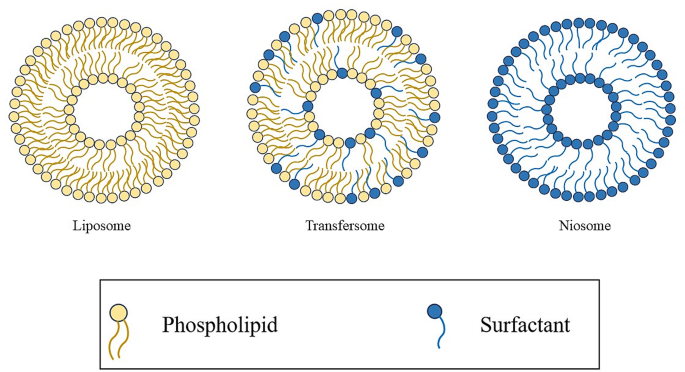
Liposome, transfersome and niosome structure
The geometry of liposomes, transfersomes and niosomes depends on the critical packing parameter (CPP), defined in Eq. 1 , where V is the volume of the non-polar group, l C is the critical length of the non-polar group, and a 0 is the area of the head polar group.
According to the CPP value, the following re-arranged geometry of phospholipids/surfactant can be obtained: spherical micelles for CPP ≤ 1/3, cylindrical micelles for 1/3 ≤ CPP ≤ 1/2, bilayers for 1/2 ≤ CPP ≤ 1 and inverse micelles for CPP > 1 (Fig. 3 ) [ 35 , 36 ].
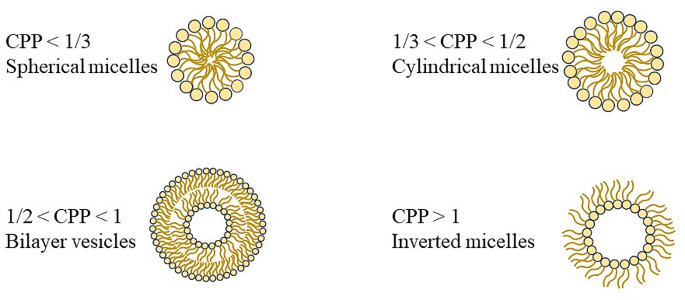
Arranged geometry of phospholipids based on CPP value
Production of nanovesicles
Different methods were proposed to produce liposomes, transfersomes and niosomes. They can be divided in conventional or traditional methods (thin film hydration method, spray-drying method, injection method, reverse phase evaporation, etc.) and innovative methods (microfluidic method, supercritical methods). In this review, the most frequently used conventional and innovative methods of production of these nanovesicles are discussed.
Conventional methods
Thin film hydration method.
Thin film hydration method, known as the Bangham method, has been the first production technique of liposomes. In this method, phospholipids are dissolved in an organic solvent, such as ethanol, chloroform or methanol, and the solvent is then evaporated forming a thin lipid layer film on the flask wall. Subsequently, the lipids are hydrated using an aqueous or phosphate buffer solution containing the hydrophilic drug, with the consequent formation of a vesicle suspension. This production method shows different disadvantages: the solvent removal is a time consuming step, and it can take several hours of exposure to vacuum; moreover, this method does not allow a good control on vesicles size and size distribution. For this reason, a sonication step is required to obtain nanovesicles instead of microvesicles. Furthermore, the encapsulation efficiency is generally low, and the process works in a batch-mode [ 37 , 38 , 39 ].
Spray-drying method
In this method, phospholipids are dissolved in an organic solvent or in a mixture of organic solvents, such as methanol and chloroform, and mixed using a magnetic stirrer; the encapsulating compound is added to the mixture, first sonicated and then it is spray-dried at different conditions of temperature (even at 120 °C) [ 40 , 41 ]. The obtained dried powder is hydrated using water or a phosphate buffer solution. Using this method, large vesicles are generally obtained (ranging from 300 to 600 nm) [ 42 , 43 ]; therefore, membrane extrusion and/or sonication are used after their production [ 41 , 42 ].
Injection method
In this method, an ethanolic solution containing phospholipids is prepared and, then, it is injected into a large volume of water or phosphate buffer; in this way, phospholipids tend to arrange in a bi-layer fragment. After the precipitation of these fragments in the aqueous phase, they tend to fuse to achieve a minimal length to finally form a close system and both, large and small unilamellar vesicles, can be obtained. The main disadvantages related to this method are the removal of residual ethanol, especially after azeotrope formation, low encapsulation efficiency and the obtained liposomal suspension is generally diluted [ 44 ]. Indeed, the volume of ethanol represents a key parameter to the formation of nanovesicles: if it is not higher than 7.5% v/v of the final volume of suspension and it is injected slowly, large and small unilamellar vesicles are formed. If the injection step is fast, multilamellar vesicles are formed [ 45 ]. Another method of injection uses ether: in this case, the problem of solvent removal can be overcome because ether and water are not miscible and can be easily removed by rotary evaporation. Encapsulation efficiency is higher than the one obtained in the ethanol injection method; but, vesicle morphology is not well controlled and mainly large unilamellar vesicles are formed [ 46 ]. Moreover, active compounds during this process are exposed to organic solvents at high temperature (ranging from 55 to 65 °C), and this can compromise the safety and the stability of the formulation [ 47 ].
Reverse phase evaporation
The lipid mixture, formed by phospholipids and an organic solvent, such as ethanol, diethyl ether or isopropyl ether, is inserted in a round flask and the solvent is evaporated under reduced pressure using a rotary evaporator; the lipids are then re-dissolved in the organic phase to form inverted micelles and the aqueous solution containing the drug is added, while the system is sonicated and kept under a nitrogen environment. The obtained mixture is evaporated to remove the residual organic solvent and the system becomes first a gel structure and, then, the structure collapses forming the suspension. In this method, a large amount of solvent is used; therefore, the problem of residual solvent in the suspension is particularly relevant [ 48 ].
Innovative methods
Despite the batch conventional methods mentioned before are very easy to perform and do not require specific and elaborated equipments, they all present several limitations and further downstream steps of homogenization of the products are required [ 49 ]. For these reasons, innovative methods of production were investigated over the years to meet industrial requirements, such as the microfluidic method and methods assisted by supercritical CO 2 .
Microfluidic method
This method is similar to the traditional injection method; but a controlled mixing between the lipid phase and the aqueous phase is possible using a microfluidic channel having a 300 μm dimension width and 130–150– 200 μm dimension height [ 50 , 51 , 52 ], with different geometries [ 36 ]. Drug encapsulation efficiency can be over 70% [ 52 ]; but, disadvantages, such as the complexity of the microfluidic design, probability of channel clogging and dilute sample concentrations, can occur [ 49 , 53 ].
Supercritical methods
More innovative methods are based on the utilization of CO 2 at supercritical conditions (SC-CO 2 ). Different processes were proposed during the year such as depressurization of an expanded solution into an aqueous medium (DESAM) that consists of the solubilization of lipids in an organic solvent, pressurization of the solution by adding CO 2 in a dense state to obtain an expanded liquid and lastly the injection of the expanded liquid in an aqueous media. The gas and the solvent can be separated and recycled; the droplets formed during the depressurization step help in the formation of homogeneous liposomes and the dense gas is also used as agitation to improve size and homogeneity of the vesicles produced [ 54 ]. This process can produce liposomes characterized by diameters ranging from about 120 nm to 387 nm; however, in some cases, multimodal distributions are obtained.
Rapid expansion of a supercritical solution (RESS) consists of a two steps preparation: a solution containing lipids is dissolved in an organic solvent and put in contact with SC-CO 2 in a vessel; subsequently, a depressurization step throughout a nozzle favours the formation of a lipid layer around the droplets, with consequent formation of liposomes [ 55 ].
Another process assisted by SC-CO 2 is the supercritical reverse phase evaporation (SCRPE) [ 56 ]. A solution formed by phospholipids and ethanol is loaded in a variable volume cell and carbon dioxide is introduced in it. Then, pressure is reduced to completely release CO 2 , obtaining a liposomal dispersion [ 57 ].
A recently proposed supercritical process is SuperSomes. In this case, an ethanolic solution containing phospholipids, and eventually a hydrophobic drug, is fed to a saturator that is a high-pressure static mixer, using a high-precision pressure pump; CO 2 is also pumped to the saturator. High-pressure mixing in the saturator produces a gas expanded liquid that is fed to the chamber throughout an injector. The aqueous phase, containing the hydrophilic drug, is fed to this chamber using a micrometric injector to produce small water droplets. In the chamber, inverted micelles are formed, since the hydrophilic heads of phospholipids arrange themselves around the sub-micron water droplets; whereas, on the bottom of the chamber, the presence of a water bulk allows the re-arrangement of phospholipids around the inverted micelles. The liposomal suspension is accumulated into a reservoir located downstream of the chamber and, opening at fixed time intervals an on-off valve, the suspension can be collected. The process can work in a continuous-mode by adding the ethanolic and aqueous solution and withdrawing the vesicle suspension. Moreover, throughout a depressurization line, the organic solvent can be completely removed and recovered in a separator vessel because of the solubility of ethanol in SC-CO 2 [ 58 , 59 ]. This one-shot process allows the production of large volumes of vesicle suspension and can be used to form liposomes [ 58 , 59 ], niosomes [ 60 , 61 ] and transfersomes [ 62 ] characterized by high drug encapsulation efficiency, even more than 90%, good control on vesicle size, size distribution and morphology, and does not require further downstream steps of particle reduction or purification [ 63 ].
Nanovesicles-based vaccines
Liposomes-based vaccines.
Different formulations of liposomes are in trials for therapeutic vaccines against malaria, influenza, tuberculosis, and HIV. Other formulations are already commercially available against infection by human papillomavirus (HPV), influenza virus, hepatitis A virus [ 64 ], and COVID-19 [ 65 ].
One of the characteristics influencing the adjuvant efficacy of liposomes is the superficial charge: cationic liposomes exhibit a major interaction with the mucosal surfaces, prolonging the exposure time of the antigen, and show the ability to form complexes together with biomacromolecules, promoting the delivery of antigens to APCs [ 66 ]. For this reason, numerous studies and formulations using cationic liposomes have been proposed.
Heuts et al. [ 67 ] prepared cationic liposomes for antigenic peptides delivery using DOTAP (1,2-dioleoyl-3-trimethylammonium-propane) and DOPC (1,2-dioleoyl-sn-glycero-3-phosphocholine) lipids by the thin film hydration method. They found that cationic liposomes were suitable for encapsulating peptides used in therapeutic cancer vaccines, increasing the immune response. In particular, 15 peptides were encapsulated in different formulations, obtaining diameters from 125 nm up to 180 nm, values of Zeta potential from + 20 mV to + 35 mV and an encapsulation efficiency up to 79%. In vitro tests were performed to determine the immunological properties of the vesicles; five formulations showed levels of T cells activation similar to the free peptides introduced in the cell line, whereas the remaining 10 formulations showed a better action than free peptides.
Surface modification of liposomes, such as the insertion of hydrophilic polymers, like polyethylene glycol (PEG), chitosan and others, is a practice used to produce the so called “stealth” liposomes that cannot be detected by macrophages and opsonized, to better deliver the encapsulated compounds. Zhuang et al. [ 68 ] produced DOTAP PEGylated (DSPE-PEG 2000 ) liposomes using the thin film hydration method, encapsulating ovalbumin (OVA), a key reference protein for vaccination experimentations. They demonstrated that the addition of 1 mol% DSPE-PEG 2000 was able to enhance the uptake of APCs up to almost 40% with respect to non-pegylated liposomes; whereas, the addition of 5 mol% PEG produced a decrease of the uptake percentage (about 5%). Moreover, they demonstrated that PEGylation enhanced passive lymph nodes (where APCs were present) targeting of liposomes. Shimizu et al. [ 69 ] produced a system encapsulating antigen (Ag) to splenic marginal zone via PEGylated liposomes. The formulation induced an efficient immune response; however, several treatments of immunizations were required to achieve a strong antitumor effect. For this reason, the liposomal structure was modified by adding an adjuvant (α-galactosylceramide, a synthetic glycolipid with a strong immunostimulant action [ 70 ]), obtaining a strong response from the immune system. Furthermore, the formulation showed a prevention action and an efficient suppression of tumour growth. Yuba et al. [ 71 ] modified the superficial charge and structure of liposomes to obtain a larger immune response; they added a cation lipid to obtain adjuvant properties because of the consequent increase of the cellular association of liposomes to dendritic cells. In addition, cationic lipids helped to modify the superficial structure with β-glucan-based pH-responsive polysaccharides that contained anionic carboxy groups; they were added to the formulation because of their adjuvant properties of promoting cytokines production that are polypeptide mediators and play an important role in the communication among immune system cells. Moreover, they show cytodestructive effects on cancer cells or infectious agents [ 72 , 73 ].
Summarizing, PEGylation of liposomes can enhance, as previously described, the residence time in the blood circulation of the nanovesicles; PEG can reduce protein absorption of nanovesicles by proteins like opsonins and lipoproteins. Despite these advantages, Schmidt et al. [ 74 ] reported a reduced binding and uptake phenomenon of liposomes by APCs, causing a loss of vaccine; in particular, the uptake level of PEGylated liposomes was reduced when PEG grafting density increased. Moreover, recent studies showed that human body is able to produce anti-PEG immunoglobulin (IgM); in particular, the production of anti-PEG IgM increased when the lipid dose increased [ 75 ]. However, studies on the recent vaccine for COVID-19 treatment demonstrated that the increase of anti-PEG IgM was recorded only after the first dose and no severe adverse reactions were observed in subjects that presented high levels of anti-PEG IgM even before the first dose administration [ 76 ]. Accelerated blood clearance (ABC) phenomenon is induced especially when repeated injections occur and in the future can affect the technique of PEGylation because of the increase of PEGylated products in the pharmaceutical field; indeed, the pre-existing anti-PEG IgM level has increased from 0.2 to 70% in the last 40 years [ 77 ]. PEG alternatives have been proposed, such the insertion of polyglycerol (PG), poly(oligo(ethylene glycol)methyl ether methacrylate) (POEGMA) and zwitterionic polymers [ 78 ]. Lila et al. [ 79 ] investigated the behaviour of PG-coated with respect to the ABC phenomenon. They produced both PEGylated liposomes (using mPEG 2000 -DSPE) and PG-liposomes (PG-DSPE) using the thin film hydration method, and encapsulated doxorubicin as model compound for cancer treatment. In vivo experiments highlighted that, after a first dose, the blood-circulating time was higher in the case of PEGylated liposomes with respect to PG-liposomes; but, after a second dose, the subjects showed a rapid clearance from the blood (4 h) and the uptake by the liver and spleen increased. Instead, subjects that received PG-coated liposomes as second dose did not show difference in blood concentrations, underlying that ABC phenomenon did not take place using PG-coated liposomes. Table 1 reports a summary of the drug encapsulated, kind of application, production technique and characteristics of liposomes discussed in this paragraph.
Transfersomes-based vaccines
Transdermal route is an easier way to deliver vaccines with respect to subcutaneous or intramuscular ones; moreover, transcutaneous immunization shows better immunogenicity [ 80 ] and has particular interest in using nanoparticles, with a diameter of about 100 nm, since they can move into hair follicles and deliver antibodies to APCs, like epidermal Langerhans cells. Particles-based vaccines for transdermal route can need a skin preparation to improve the permeability of the particles, such as: electroporation, sonophoresis, laser ablation and skin abrasion; but this can be a very aggressive and uncommon practice. To avoid the use of these invasive methods, transfersomes can be used for transdermal vaccine delivery; they are able to change their shape during the drug delivery and transportation throughout the skin (Fig. 4 ) [ 81 ]. The transport of transfersomes through the skin is due to the osmotic force or to the hydration. Mammalian skin can control hydration by generating a gradient between the epidermis and the dry stratum corneum; when the transfersome suspension is applied on the skin, a partial dehydration of vesicles occurs that makes them able to deform and pass through skin pores because of the hydration gradient: i.e., they penetrate in the more hydrated skin layer [ 82 ].
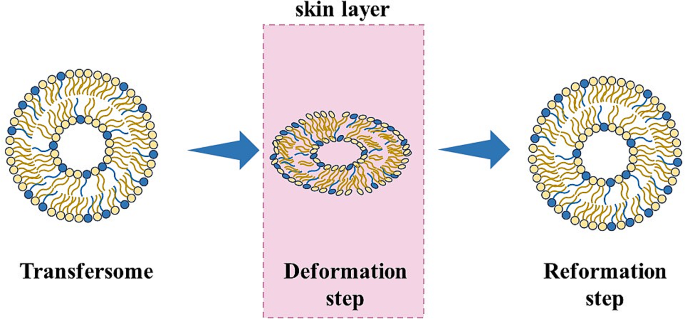
Mechanism of transfersomes permeation through the skin layer
Gupta et al. [ 83 ] produced transfersomes formed by soya phosphatidylcholine (PC) as lipid and sodium deoxycholate as surfactant, using the thin film method, to be used for a non-invasive delivery system of tetanus toxoid. The obtained vesicle suspension was extruded through different polycarbonate membranes (dimension less than 0.45 μm) to reduce the vesicles dimension, although part of the suspension was inevitably lost. Different ratios of PC: surfactant were investigated to increase the deformability of the vesicles. In this work, elasticity of transfersomes was expressed throughout the deformability index, measured by extruding the vesicles through a 50 nm pore membrane and it was proportional to the amount of suspension extruded in a certain time (J), the radius of the transfersomes (r v ) and the size of the membrane pore (r p ) (Eq. 2 ):
This is not the only way to define the deformability index; other definitions are widely used in the literature [ 84 , 85 ]. Membranes are generally used since, to pass the mammalian skin, transfersomes must be capable to cross pores with a diameter less than 50 nm [ 86 ]. The results of this work showed that increasing the surfactant concentration over a limit value did not give advantages in terms of deformability index; the optimum ratio PC: surfactant was equal to 85:15. Fluorescence microscopy, using 6-carboxyfluorescein loaded transfersomes was performed using a rat skin and showed that the transfersomes penetrated even in a deeper skin layer. In vivo study demonstrated the effective immune response of the transfersomes that was comparable with the traditional intramuscular administration.
The efficacy of immune response can be affected by the kind of phospholipids used; as discussed before for liposomes, cation lipids were used to have an effective delivery of the antigen to the APCs. Also in this case, cation transfersomes are preferred, even for a better interaction with the negatively charged skin stratum corneum [ 26 ]. Mahor et al. [ 87 ] used cation lipid DOTMA (N-[1-(2,3-dioleyloxy)-propyl]-N, N,N-trimethylammonium chloride) and sodium deoxycholate as a surfactant, to produce vaccines against hepatitis B. Transfersomes were obtained by a modified reverse phase evaporation technique and the final suspension was extruded using a 100 nm polycarbonate filter. Subsequently, these transfersomes were introduced in a suspension containing plasmid DNA to permit the adsorption of negatively charged plasmid DNA on the transfersomes surface. These authors obtained nanovesicles characterized by an average diameter between 85 and 91 nm, and a drug encapsulation efficiency from 69% up to 88%.
However, the literature reports that the only application of transfersomes do not increase immunogenicity, and a skin preparation with microneedles is needed [ 80 ]. Microneedles are used to create a route for nanoparticles to penetrate the skin in a painless way [ 88 ]. In this regard, Wu et al. [ 89 ] prepared cationic and anionic transfersomes integrated with microneedles, encapsulating ovalbumin (OVA), as a model antigen. Egg yolk phosphatidylcholine was used as a lipid, and sodium cholate (SC) and stearylamine (SA) were used as surfactants, to obtain anionic and cationic transfersomes, respectively. They were produced using the thin film hydration method, and multilayer nanovesicles with dimensions ranging from about 122 nm to 199 nm, and a drug encapsulation efficiency of about 78%, were obtained in the case of cationic transfersomes. Microneedles were prepared using hyaluronic acid (HA) at different molecular weights in a micromold and dried at room temperature. To prepare transfersomes loaded microneedles, the HA powder was first dissolved in the nanovesicles suspension and, then, put in the mold and dried. Microneedle patches showed a complete dissolution after 20 min of application on rat skin, proving a high dissolving capacity in the skin. In contrast with the literature, in this case, a larger uptake from dendritic cells was reached by the anionic transfersomes; but it could be related to HA modification. Table 2 reports a summary of the drug encapsulated, kind of application, production technique and characteristics of transfersomes discussed in this paragraph.
Niosomes-based vaccines
Niosomes represent another system for drug delivery and skin immunization; thanks to the surfactant characteristics, they are able to improve their fluidity throughout the stratum corneum [ 90 ]. They can be formed by the same surfactant or different surfactants and the most used ones are Span, Tween and Brij. Differently from liposomes, niosomes present a longer stability and are cheaper because of the use of surfactants [ 91 ].
The adjuvant activity of niosomes was studied for the first time by Brewer et al. [ 92 ], when they found the enhancing activity of niosomes in antibody production against bovine serum albumin (BSA); in particular, these authors found that niosomes were stimulators in type 1 T cells helper (Th1) response. Vyas et al. [ 93 ] presented a study where liposomes and niosomes encapsulating DNA encoding hepatitis B surface antigen (HbsAg) were produced and their immune actions were compared. Both liposomes and niosomes were obtained by the reverse phase evaporation method and were characterized by a mean size of 2.8 μm and 2.3 μm, respectively. The drug encapsulation was about 49% for liposomes and 45% for niosomes. The immune response was investigated, and niosomes showed a larger action than liposomes, with a larger production of specific antibodies (anti-HbsAg) in serum after topical application. Moreover, even intramuscular HbsAg was injected, showing a better response for the first 4 weeks; but, after that period, antibodies decreased in serum, whereas, in the case of liposomes and niosomes, the initial immune response was lower and increased after 8 weeks from the topical administration. Obeid et al. [ 94 ] showed that the immune response can be influenced by the nanocarrier preparation method. They produced niosomes using the thin film hydration method and microfluidic, to encapsulate influenza antigen. Thin film hydration method showed a drug entrapment efficiency of about 48%; whereas, it was about 57% in the case of niosomes produced via microfluidic. Moreover, larger nanovesicles were produced using the thin film hydration method (Z-average = 388.8 nm); whereas, a narrower size distribution and a smaller Z-average (122.1 nm) were obtained by microfluidic. The difference in size and encapsulation efficiency can affect the action of produced nanovesicles. Both vesicles did not show a significative difference with the free antigen in Th2 response, and this could be related to the low encapsulation efficiency obtained. Niosomes produced by the thin film hydration showed instead a larger Th1 response and this result was related to the mean dimension of the vesicles [ 92 , 95 ]. In a recent work of Hassouna et al. [ 96 ], niosomes were prepared via thin film hydration method, using cholesterol and Span 60, and loaded with toxoplasma lysate antigen (TLA), to be used for the treatment of rheumatoid arthritis. Negatively charged nanovesicles (Z-average 164.12 nm) were obtained, and a 75% drug encapsulation efficiency was measured.
As discussed previously for transfersomes, the permeation properties of niosomes can be enhanced using microneedle patches that can be also auto-administrated [ 97 ]. Boonada et al. [ 98 ] prepared cationic niosomes to enhance skin immunization via hollow microneedle. Cationic niosomes formed by Span 20, cholesterol and a cationic lipid (N1, N1-dimyristeroyloxyethyl-spermine), in a molar ratio 2.5:2.5:0.5 mM, were produced using the thin film hydration method, and plasmid DNA-encoding ovalbumin was encapsulated. After sonication, particles were still of large dimensions because of the adsorption of anionic plasmid DNA on the cationic niosomes surface. In vivo studies were performed to determine the efficacy of the nanovesicles produced; high levels of IgG antibodies in all mice vaccinated with the microneedles were obtained. Moreover, no infections or bleeding were observed on the skin area where the patch was used. Zhang et al. [ 99 ] produced carbomer hydrogel niosomes for transcutaneous vaccine delivery. Niosomes (using Span 80, cholesterol and sterylamine) were produced by the thin film hydration method and were characterized by a mean diameter of about 300 nm and an ovalbumin encapsulation efficiency of about 49%. Then, the niosomal suspension was added to a solution of Carbopol 934 in PBS to obtain a loaded hydrogel, whereas the niosomal gel was obtained by adding niosomal suspensions to a solution of Carbopol in 20% ethanol/PBS. Hydrophobic and hydrophilic membranes were used to simulate the skin layer. The hydrophilic layer presented more resistance to the penetration of the niosomal gel; whereas, the niosomal hydrogel delivered antigens even into the deeper hydrophilic layer. Table 3 reports a summary of the drug encapsulated, kind of application, production technique and characteristics of niosomes discussed in this paragraph.
A comparative study for non-invasive vaccine delivery
A comparative study to better understand which non-invasive delivery strategy could be the best one among liposomes, transfersomes and niosomes, was proposed by Gupta et al. [ 100 ]. Liposomes and niosomes were produced by the reverse phase evaporation method; whereas transfersomes were produced by the thin film evaporation method. In all the cases, the obtained suspension was extruded throughout polycarbonate filters of different porosities to control the dimensions of the nanovesicles; the size measured for transfersomes, liposomes and niosomes was 196 nm, 221 nm and 214 nm, respectively. Encapsulation efficiency and deformability were higher only for transfersomes (72% drug encapsulation of transfersomes against 42% and 41% for liposomes and niosomes, respectively, and a deformability index of one order of magnitude higher than the one calculated for liposomes and niosomes). In vivo results highlighted the superior action of transfersomes in the case of topical administration with respect to niosomes and liposomes; indeed, transfersomes exhibited an immune response similar to that of intramuscular administration of naked antigen, whereas niosomes and liposomes showed a weaker immune response. The fact that, in this study, transfersomes did not give a larger immune response than the injection administration can be related to the method of preparation, as well as the weaker action of niosomes can be related to the low encapsulation efficiency of the antigen. For these reasons, transfersomes can be considered the best choice for transdermal delivery: they can enhance the permeation of both low and high molecular weight compounds [ 101 ]; however, also niosomes, as reported before, could be used for transdermal delivery, but more studies and optimized formulations are needed to improve their elasticity. Liposomes do not present deformable properties because of their nonelastic features [ 102 ]; therefore, they can be preferred in the case of intramuscular administration. Nevertheless, further studies have to be performed and compared to verify and validate this conclusion.
Conclusions and perspectives
Liposomes, transfersomes and niosomes used to deliver mRNA and DNA-based vaccines show larger efficacy and reduced side effects than the traditional vaccine formulations. In particular, transfersomes can be the best choice for transdermal delivery because of their elasticity and deformability properties.
The most used traditional methods for nanovesicles production are the thin film hydration method and the reverse phase evaporation; but some limitations occur due to their intrinsic batch-mode configuration, post-processing steps of sonication or extrusion to reduce the vesicle size, and purification procedures to remove organic solvent residues.
Supercritical CO 2 -based processes can overcome some of these problems. In particular, SuperSomes is a continuous and one-shot technique that was proposed to produce liposomes, transfersomes and niosomes encapsulating both hydrophilic and lipophilic drugs. Adopting this approach, the industrial requirements can more readily be satisfied; but, further studies have to be performed to validate the application of this process to the treatment of high added value molecules, like RNA and DNA.
Data availability
Not applicable.
Abbreviations
Accelerated blood clearance
Antigen presenting cells
Critical packing parameter
1,2-dioleoyl-sn-glycero-3-phosphocholine
1,2-dioleoyl-3-trimethylammonium-propane
Hyaluronic acid
Hepatitis B surface antigen
Hydrogenated egg phosphatidylcholine
Immunoglobulin
Phosphatidylcholine
Polyethylene glycol
Polyglycerol
Poly(oligo(ethylene glycol)methyl ether methacrylate)
Sterylamine
Sodium cholate
Type 1 T helper
Type 2 T helper
De Gregorio E, Rappuoli R. From empiricism to rational design: a personal perspective of the evolution of vaccine development. Nat Rev Immunol. 2014;14(7):505–14. https://doi.org/10.1038/nri3694 .
Article CAS PubMed PubMed Central Google Scholar
Firouzabadi N, Ghasemiyeh P, Moradishooli F, Mohammadi-Samani S. Update on the effectiveness of COVID-19 vaccines on different variants of SARS-CoV-2. Int Immunopharmacol. 2023;117:109968. https://doi.org/10.1016/j.intimp.2023.109968 .
Tojjari A, Saeed A, Singh M, Cavalcante L, Sahin IH, Saeed A. A comprehensive review on cancer vaccines and vaccine strategies in hepatocellular carcinoma. Vaccines. 2023;11:1357. https://doi.org/10.3390/vaccines11081357 .
Esparza J. Three different paths to introduce the smallpox vaccine in early 19th century United States. Vaccine. 2020;38(12):2741–5. https://doi.org/10.1016/j.vaccine.2020.01.077 .
Article PubMed Google Scholar
Jenner E. An inquiry into the causes and effects of the variolæ vaccinæ, a disease discovered in some of the western counties of England, particularly Gloucestershire, and known by the name of the cow pox. Dawsons Pall Mall; 1966.
Smith KA. Louis Pasteur, the father of immunology? Front Immunol. 2012;3:68. https://doi.org/10.3389/fimmu.2012.00068 .
Article PubMed PubMed Central Google Scholar
Pasteur L. De L’attenuation Du virus Du Cholera Des poules. CR Acad Sci Paris. 1880;91:673–80.
Google Scholar
Glenny AT, Hopkins BE. Diphtheria Toxoid as an Immunising Agent. Br J Exp Pathol. 1923;4(5):283–8.
CAS PubMed Central Google Scholar
Varsha S, Joshi, Ishwar BB, Shrikant AS, Rekha SS, Kennedy JF. Meningococcal polysaccharide vaccines: a review. Carbohydr Polym. 2009;75(4):553–65. https://doi.org/10.1016/j.carbpol.2008.09.032 .
Article CAS Google Scholar
Bouazzaoui A, Abdellatif AAH, Al-Allaf FA, Bogari NM, Al-Dehlawi S, Qari SH. Strategies for vaccination: conventional vaccine approaches Versus New-Generation Strategies in Combination with adjuvants. Pharmaceutics. 2021;13(2):140. https://doi.org/10.3390/pharmaceutics13020140 .
Bayani F, Hashkavaei NS, Arjmand S, et al. An overview of the vaccine platforms to combat COVID-19 with a focus on the subunit vaccines. Prog Biophys Mol Biol. 2023;178:32–49. https://doi.org/10.1016/j.pbiomolbio.2023.02.004 .
Perrie Y, Mohammed AR, Kirby DJ, McNeil SE, Bramwell VW. Vaccine adjuvant systems: enhancing the efficacy of sub-unit protein antigens. Int J Pharm. 2008;364(2):272–80. https://doi.org/10.1016/j.ijpharm.2008.04.036 .
Article CAS PubMed Google Scholar
Shi S, Zhu H, Xia X, Liang Z, Ma X, Sun B. Vaccine adjuvants: understanding the structure and mechanism of adjuvanticity. Vaccine. 2019;37(24):3167–78. https://doi.org/10.1016/j.vaccine.2019.04.055 .
Tretiakova DS, Vodovozova EL. Liposomes as adjuvants and Vaccine Delivery systems. Biochem (Mosc) suppl ser A membr. Cell Biol. 2022;16(1):1–20. https://doi.org/10.1134/S1990747822020076 .
Sayour EJ, Mendez-Gomez HR, Mitchell DA. Cancer Vaccine Immunotherapy with RNA-Loaded liposomes. Int J Mol Sci. 2018;19(10):2890. https://doi.org/10.3390/ijms19102890 .
Xue H, Guo P, Wen WC, Wong H. Lipid-based nanocarriers for RNA delivery. Curr Pharm Des. 2015;21(22):3140–7. https://doi.org/10.2174/1381612821666150531164540 .
Zhi D, Zhang S, Wang B, Zhao Y, Yang B, Yu S. Transfection Efficiency of Cationic Lipids with different hydrophobic domains in Gene Delivery. Bioconjug Chem. 2010;21(4):563–77. https://doi.org/10.1021/bc900393r .
Allison AG, Gregoriadis G. Liposomes as immunological adjuvants. Nature. 1974;252(5480):252. https://doi.org/10.1038/252252a0 .
Ye Z, Harmon JF, Ni W, Li Y, Wich D, Xu Q. The mRNA Vaccine Revolution: COVID-19 has launched the future of Vaccinology. ACS Nano. 2023;17(16):15231–53. https://doi.org/10.1021/acsnano.2c12584 .
Al Fayez N, Nassar MS, Alshehri AA, et al. Recent Advancement in mRNA Vaccine Development and Applications. Pharmaceutics. 2023;15(7):1972. https://doi.org/10.3390/pharmaceutics15071972 .
Bettini E, Locci M. SARS-CoV-2 mRNA vaccines: immunological mechanism and Beyond. Vaccines (Basel). 2021;9(2):147. https://doi.org/10.3390/vaccines9020147 .
Xiang SD, Scholzen A, Minigo G, Davi C, Apostolopoulos V, Mottram PL, Plebanski M. Pathogen recognition and development of particulate vaccines: does size matter? Methods. 2006;40(1):1–9. https://doi.org/10.1016/j.ymeth.2006.05.016 .
Tiwari G, Tiwari R, Sriwastawa B, et al. Drug delivery systems: an updated review. Int J Pharm Investig. 2012;2(1):2–11. https://doi.org/10.4103/2230-973X.96920 .
Perkins WR, Minchey SR, Ahl PL, Janoff AS. The determination of liposome captured volume. Chem Phys Lipids. 1993;64(1–3):197–217. https://doi.org/10.1016/0009-3084(93)90066-C .
Dymek M, Sikora E. Liposomes as biocompatible and smart delivery systems– the current state. Adv Colloid Interface Sci. 2022;309:102757. https://doi.org/10.1016/j.cis.2022.102757 .
Li Y, Tai Z, Ma J, Miao F, Xin R, Shen C, et al. Lycorine transfersomes modified with cell-penetrating peptides for topical treatment of cutaneous squamous cell carcinoma. J Nanobiotechnol. 2023;21(1):139. https://doi.org/10.1186/s12951-023-01877-4 .
Nayak D, Tippavajhala V. A Comprehensive Review on Preparation, evaluation and applications of deformable liposomes. Iran J Pharm Res. 2021;20(1):186–205. https://doi.org/10.22037/ijpr.2020.112878.13997 .
Benson HA. Transfersomes for transdermal drug delivery. Expert Opin Drug Deliv. 2006;3(6):727–37. https://doi.org/10.1517/17425247.3.6.727 .
Moghassemi S, Hadjizadeh A. Nano-Niosomes as Nanoscale drug delivery systems: an illustrated review. J Control Release. 2014;185:22–36. https://doi.org/10.1016/j.jconrel.2014.04.015 .
Marianecci C, Di Marzio L, Rinaldi F, et al. Niosomes from 80s to present: the state of the art. Adv Colloid Interface Sci. 2014;205:187–206. https://doi.org/10.1016/j.cis.2013.11.018 .
Chen S, Hanning S, Falconer J, Locke M, Wen J. Recent advances in non-ionic surfactant vesicles (niosomes): fabrication, characterization, pharmaceutical and cosmetic applications. Eur J Pharm Biopharm. 2019;144:18–39. https://doi.org/10.1016/j.ejpb.2019.08.015 .
Ahmad MZ, Ahmad J, Alasmary MY, Abdel-Wahab BA, Warsi MH, Haque A, Chaubey P. Emerging advances in cationic liposomal cancer nanovaccines: opportunities and challenges. Immunotherapy. 2021;13(6):491–507. https://doi.org/10.2217/imt-2020-0258 .
Bolhassani A. Lipid-based Delivery systems in Development of Genetic and Subunit vaccines. Mol Biotechnol. 2022. https://doi.org/10.1007/s12033-022-00624-8 .
Henriksen-Lacey M, Christensen D, Bramwell VW, Lindenstrøm T, Agger EM, Andersen P, Perrie Y. Liposomal cationic charge and antigen adsorption are important properties for the efficient deposition of antigen at the injection site and ability of the vaccine to induce a CMI response. J Controlled Release. 2010;145(2):102–8. https://doi.org/10.1016/j.jconrel.2010.03.027 .
Yasamineh S, Yasamineh P, Ghafouri Kalajahi H, et al. A state-of-the-art review on the recent advances of niosomes as a targeted drug delivery system. Int J Pharm. 2022;624:121878. https://doi.org/10.1016/j.ijpharm.2022.121878 .
Has C, Sunthar P. A comprehensive review on recent preparation techniques of liposomes. J Liposome Res. 2019;30(4):336–65. https://doi.org/10.1080/08982104.2019.1668010 .
Bangham AD. Surrogate cells or trojan horses. The discovery of liposomes. BioEssays. 1995;17(12):1081–8. https://doi.org/10.1002/bies.950171213 .
Meure LA, Foster NR, Dehghani F. Conventional and dense gas techniques for the production of liposomes: a review. AAPS PharmSciTech. 2008;9(3):798–809. https://doi.org/10.1208/s12249-008-9097-x .
Dua JS, Rana AC, Bhandari AK. Liposome: methods of preparation and applications.Int. J Pharm Stud Res. 2010;3(2):14–20.
Maniyar MG, Kokare CR. Formulation and evaluation of spray dried liposomes of lopinavir for topical application. J Pharm Invest. 2018;49(1):259–70. https://doi.org/10.1007/s40005-018-0403-7 .
van den Hoven JM, Metselaar JM, Storm G, Beijnen JH, Nuijen B. Cyclodextrin as membrane protectant in spray-drying and freeze-drying of PEGylated liposomes. Int J Pharm. 2012;438:209–16.
Skalko-Basnet N, Pavelic Z, Becirevic-Lacan M. Liposomes containing drug and cyclodextrin prepared by the one-step spray-drying method. Drug Dev Ind Pharm. 2000;26(12):1279–84. https://doi.org/10.1081/ddc-100102309 .
Patil-Gadhe A, Pokharkar V. Single step spray drying method to develop proliposomes for inhalation: a systematic study based on quality by design approach. Pulm Pharmacol Ther. 2014;27:197–207.
Gouda A, Sakr OS, Nasr M, Sammour O. Ethanol injection technique for liposomes formulation: an insight into development, influencing factors, challenges and applications. J Drug Delivery Sci Technol. 2021;61:102174. https://doi.org/10.1016/j.jddst.2020.102174 .
Gouda A, Sakr OS, Nasr M, Sammour O. Ethanol injection technique for liposomes formulation: an insight into development, influencing factors, challenges and applications. J Drug Delivery Sci Technol. 2021;61:102174.
Šturm L, Poklar Ulrih N. Basic methods for Preparation of liposomes and studying their interactions with different compounds, with the emphasis on polyphenols. Int J Mol Sci. 2021;22(12):6547. https://doi.org/10.3390/ijms22126547 .
Lombardo D, Kiselev MA. Methods of liposomes Preparation: formation and Control Factors of Versatile Nanocarriers for Biomedical and Nanomedicine Application. Pharmaceutics. 2022;14:543.
Choudhury A, Sonowal K, Laskar RE, Deka D, Dey BK. Liposome: a carrier for effective drug delivery. J Appl Pharm Res. 2020;8(1):22–8. https://doi.org/10.18231/j.joapr.2019.v.8.i.1.003 .
Kotouček J, Hubatka F, Mašek J, et al. Preparation of nanoliposomes by microfluidic mixing in herring-bone channel and the role of membrane fluidity in liposomes formation. Sci Rep. 2020;10(1):5595. https://doi.org/10.1038/s41598-020-62500-2 .
Yuka MS, et al. Controlling lamellarity and physicochemical properties of liposomes prepared using a microfluidic device. Biomater Sci. 2023;11(7):2419–26. https://doi.org/10.1039/D2BM01703B .
Article Google Scholar
Xiu X, Ma S, Li Z, Li Z, Li X, Ruan Y, Ma F. Novel 3D integrated microfluidic device: design, construction, and application to the preparation of liposomes for vaccine delivery. J Drug Delivery Sci Technol. 2024;91:105191–1. https://doi.org/10.1016/j.jddst.2023.105191 .
Delama A, Teixeira MI, Dorati R, Genta I, Conti B, Lamprou DA. Microfluidic encapsulation method to produce stable liposomes containing iohexol. J Drug Delivery Sci Technol. 2019;54:101340. https://doi.org/10.1016/j.jddst.2019.101340 .
Shepherd SJ, Issadore D, Mitchell MJ. Microfluidic formulation of nanoparticles for biomedical applications. Biomaterials. 2021;274:120826. https://doi.org/10.1016/j.biomaterials.2021.120826 .
Meure LA, Knott R, Foster NR, Dehghani F. The depressurization of an expanded solution into aqueous media for the bulk production of liposomes. Langmuir. 2008;25(1):326–37. https://doi.org/10.1021/la802511a .
Soh SH, Lee LY. Microencapsulation and Nanoencapsulation using supercritical fluid (SCF) techniques. Pharmaceutics. 2019;11(1):21. https://doi.org/10.3390/pharmaceutics11010021 .
Imura T, Otake K, Hashimoto S, Gotoh T, Yuasa M, Yokoyama S, Sakai H, Rathman JF, Abe M. Preparation and physicochemical properties of various soybean lecithin liposomes using supercritical reverse phase evaporation method. Coll Surf B Biointerfaces. 2003;27:133–40. https://doi.org/10.1016/S0927-7765(02)00048-6 .
Otake K, Imura T, Sakai H, Abe M. Development of a New Preparation Method of liposomes using supercritical Carbon Dioxide. Langmuir. 2001;17(13):3898–901. https://doi.org/10.1021/la010122k .
Chaves MA, Baldino L, Pinho SC, Reverchon E. Supercritical CO 2 assisted process for the production of mixed phospholipid nanoliposomes: unloaded and vitamin D 3 loaded vesicles. J Food Eng. 2022;316. https://doi.org/10.1016/j.jfoodeng.2021.110851 .
Chaves MA, Baldino L, Pinho SC, Reverchon E. Co-encapsulation of curcumin and vitamin D 3 in mixed phospholipid nanoliposomes using a continuous supercritical CO 2 assisted process. J Taiwan Inst Chem Eng. 2022;132. https://doi.org/10.1016/j.jtice.2021.10.020 .
Baldino L, Reverchon E. Niosomes formation using a continuous supercritical CO2 assisted process. J CO2 Util. 2021;52:101669. https://doi.org/10.1016/j.jcou.2021.101669 .
Baldino L, Reverchon E. Continuous supercritical CO2 assisted process for the production of nano-niosomes loaded with a second-generation antibiotic for ocular therapy. J Supercrit Fluids. 2022;188:105673. https://doi.org/10.1016/j.supflu.2022.105673 .
Squittieri R, Baldino L, Reverchon E. Production of antioxidant transfersomes by a supercritical CO2 assisted process for Transdermal Delivery Applications. Nanomaterials (Basel). 2023;13(12):1812. https://doi.org/10.3390/nano13121812 .
Trucillo P, Campardelli R, Scognamiglio M, Reverchon E. Control of liposomes diameter at micrometric and nanometric level using a supercritical assisted technique. J CO2 Util. 2019;32:119–27. https://doi.org/10.1016/j.jcou.2019.04.014 .
Nisini R, Poerio N, Mariotti S, De Santis F, Fraziano M. The Multirole of liposomes in Therapy and Prevention of Infectious diseases. Front Immunol. 2018;9:155. https://doi.org/10.3389/fimmu.2018.00155 .
Gregoriadis G. Liposomes and mRNA: two technologies together create a COVID-19 vaccine. Med Drug Discov. 2021;12:100104. https://doi.org/10.1016/j.medidd.2021.100104 .
Article CAS PubMed Central Google Scholar
Gao J, Ochyl LJ, Yang E, Moon J. Cationic liposomes promote antigen cross-presentation in dendritic cells by alkalizing the lysosomal pH and limiting the degradation of antigens. Int J Nanomed. 2017;12:1251–64. https://doi.org/10.2147/IJN.S125866 .
Heuts J, Varypataki EM, van der Maaden K, et al. Cationic liposomes: a flexible vaccine delivery system for Physicochemically Diverse antigenic peptides. Pharm Res. 2018;35(11):207. https://doi.org/10.1007/s11095-018-2490-6 .
Zhuang Y, Ma Y, Wang C, et al. PEGylated cationic liposomes robustly augment vaccine-induced immune responses: role of lymphatic trafficking and biodistribution. J Control Release. 2012;159(1):135–42. https://doi.org/10.1016/j.jconrel.2011.12.017 .
Shimizu T, Abu Lila AS, Kawaguchi Y, et al. A Novel platform for Cancer vaccines: Antigen-Selective delivery to splenic marginal zone B cells via repeated injections of PEGylated liposomes. J Immunol. 2018;201(10):2969–76. https://doi.org/10.4049/jimmunol.1701351 .
Rampuria P, Lang ML. Regulation of Humoral immunity by CD1d-Restricted Natural Killer T Cells. Immunology. Acad Press. 2018;55–73. https://doi.org/10.1016/B978-0-12-809819-6.00005-8 .
Yuba E, Kado Y, Kasho N, Harada A. Cationic lipid potentiated the adjuvanticity of polysaccharide derivative-modified liposome vaccines. J Control Release. 2023;362:767–76. https://doi.org/10.1016/j.jconrel.2022.10.016 .
Cohen MC, Cohen S. Cytokine function: a study in biologic diversity. Am J Clin Pathol. 1996;105(5):589–98. https://doi.org/10.1093/ajcp/105.5.589 .
Saxton RA, Glassman CR, Garcia KC. Emerging principles of cytokine pharmacology and therapeutics. Nat Rev Drug Discov. 2023;22(1):21–37. https://doi.org/10.1038/s41573-022-00557-6 .
Schmidt ST, Olsen CL, Franzyk H, et al. Comparison of two different PEGylation strategies for the liposomal adjuvant CAF09: towards induction of CTL responses upon subcutaneous vaccine administration. Eur J Pharm Biopharm. 2019;140:29–39. https://doi.org/10.1016/j.ejpb.2019.04.020 .
Takata H, Shimizu T, Yamade R, et al. Anti-PEG IgM production induced by PEGylated liposomes as a function of administration route. J Control Release. 2023;360:285–92. https://doi.org/10.1016/j.jconrel.2023.06.027 .
Bavli Y, Chen BM, Gross G, et al. Anti-PEG antibodies before and after a first dose of Comirnaty® (mRNA-LNP-based SARS-CoV-2 vaccine). J Control Release. 2023;354:316–22. https://doi.org/10.1016/j.jconrel.2022.12.039 .
Lou C. PEgylation, the glorious past, present dilemma and future. Highlights Sci Eng Technol. 2023;40:338–44. https://doi.org/10.54097/hset.v40i.6702 .
Knop K, Hoogenboom R, Fischer D, Schubert U. Poly(ethylene glycol) in drug delivery: pros and cons as Well as potential Alternatives. Angew Chem Int Ed. 2010;49:6288–308. https://doi.org/10.1002/anie.200902672 .
Lila AS, Nawata K, Shimizu T, Ishida T, Kiwada H. Use of polyglycerol (PG), instead of polyethylene glycol (PEG), prevents induction of the accelerated blood clearance phenomenon against long-circulating liposomes upon repeated administration. Int J Pharm. 2013;456(1):235–42. https://doi.org/10.1016/j.ijpharm.2013.07.059 .
Dewangan HK, Tomar S. Nanovaccine for transdermal delivery system. J Drug Delivery Sci Technol. 2022;67:102988. https://doi.org/10.1016/j.jddst.2021.102988 .
Simrah HA, Usmani SA, Izhar MP. Transfersome, an ultra-deformable lipid-based drug nanocarrier: an updated review with therapeutic applications. Naunyn Schmiedebergs Arch Pharmacol. 2023. https://doi.org/10.1007/s00210-023-02670-8 .
Piumitali B, Neeraj U, Jyotivardhan J. Transfersome– a nanoscience in transdermal drug delivery and its clinical advancements. Int J Nanosci. 2020;19(4):1950033. https://doi.org/10.1142/S0219581X19500339 .
Gupta PN, Mishra V, Singh P, et al. Tetanus toxoid-loaded transfersomes for topical immunization. J Pharm Pharmacol. 2005;57(3):295–301. https://doi.org/10.1211/0022357055515 .
Shuwaili AHAL, Rasool BKA, Abdulrasool AA. Optimization of elastic transfersomes formulations for transdermal delivery of pentoxifylline. Eur J Pharm Biopharm. 2016;102. https://doi.org/10.1016/j.ejpb.2016.02.013 . 101– 14.
Potisuwan S, Apichatwatana N, Rujivipat S. Improved skin permeation of transferosomes containing Eulophia macrobulbon extract. Colloids Surf B. 2023;229:113474. https://doi.org/10.1016/j.colsurfb.2023.113474 .
Cevc G, Schätzlein AG, Blume GD. Transdermal drug carriers– basic properties, optimization and transfer efficiency in the case of epicutaneously applied peptides. J Controlled Release. 1995;36(1–2):3–16. https://doi.org/10.1016/0168-3659(95)00056-E .
Mahor S, Rawat A, Dubey PK, et al. Cationic transfersomes based topical genetic vaccine against hepatitis B. Int J Pharm. 2007;340(1–2):13–9. https://doi.org/10.1016/j.ijpharm.2007.03.006 .
Sun X, Zeng L, Huang Y. Transcutaneous delivery of DNA/mRNA for cancer therapeutic vaccination. J Gene Med. 2019;21(7):3089. https://doi.org/10.1002/jgm.3089 .
Wu X, Li Y, Chen X, Zhou Z, Pang J, Luo X, Kong M. A surface charge dependent enhanced Th1 antigen-specific immune response in lymph nodes by transfersome-based nanovaccine-loaded dissolving microneedle-assisted transdermal immunization. J Mater Chem B. 2019;7(31):4854–66. https://doi.org/10.1039/C9TB00448C .
Polaka S, Makwana V, Vasdev N, et al. Engineering immunity via skin-directed drug delivery devices. J Control Release. 2022;345:385–404. https://doi.org/10.1016/j.jconrel.2022.03.013 .
Ge X, Wei M, He S, Yuan WE. Advances of non-ionic surfactant vesicles (Niosomes) and their application in Drug Delivery. Pharmaceutics. 2019;11(2):55. https://doi.org/10.3390/pharmaceutics11020055 .
Brewer JM, Alexander J. The adjuvant activity of non-ionic surfactant vesicles (niosomes) on the BALB/c humoral response to bovine serum albumin. Immunology. 1992;75(4):570–5.
CAS PubMed PubMed Central Google Scholar
Vyas SP, Singh RP, Jain S, et al. Non-ionic surfactant based vesicles (niosomes) for non-invasive topical genetic immunization against hepatitis B. Int J Pharm. 2005;296(1–2):80–6. https://doi.org/10.1016/j.ijpharm.2005.02.016 .
Obeid MA, Teeravatcharoenchai T, Connell D, et al. Examination of the effect of niosome preparation methods in encapsulating model antigens on the vesicle characteristics and their ability to induce immune responses. J Liposome Res. 2021;31(2):195–202. https://doi.org/10.1080/08982104.2020.1768110 .
Mann JF, Shakir E, Carter KC, Mullen AB, Alexander J, Ferro VA. Lipid vesicle size of an oral influenza vaccine delivery vehicle influences the Th1/Th2 bias in the immune response and protection against infection. Vaccine. 2009;27(27):3643–9. https://doi.org/10.1016/j.vaccine.2009.03.040 .
Hassouna SS, Allam EA, Sheta E, et al. Vaccination with Toxoplasma lysate antigen or its encapsulated niosomes form immunomodulates adjuvant-induced arthritis through JAK3 downregulation. Inflammopharmacology. 2023;31(6):3101–14. https://doi.org/10.1007/s10787-023-01267-0 .
Menon I, Bagwe P, Gomes KB, et al. Microneedles: a New Generation Vaccine Delivery System. Micromachines (Basel). 2021;12(4):435. https://doi.org/10.3390/mi12040435 .
Pamornpathomkul B, Niyomtham N, Yingyongnarongkul BE, et al. Cationic niosomes for enhanced skin immunization of plasmid DNA-Encoding Ovalbumin via Hollow Microneedles. AAPS PharmSciTech. 2018;19(1):481–8. https://doi.org/10.1208/s12249-017-0855-5 .
Zhang Y, Cao F, Ullah A. A comparative study of niosomal and elastic niosomal carbomer hydrogel for transcutaneous vaccine delivery. Mater Today Commun. 2022;31:103738. https://doi.org/10.1016/j.mtcomm.2022.103738 .
Gupta PN, Mishra V, Rawat A, et al. Non-invasive vaccine delivery in transfersomes, niosomes and liposomes: a comparative study. Int J Pharm. 2005;293(1–2):73–82. https://doi.org/10.1016/j.ijpharm.2004.12.022 .
Rayate YT, Yadav AR, Mohite SK. Novel Drug Delivery systems and its future prospects. World J Appl Pharm. 2023;1(1):14–9.
Takechi-Haraya Y, Sakai-Kato K, Abe Y, Kawanishi T, Okuda H, Goda Y. Atomic force microscopic analysis of the effect of lipid composition on liposome membrane rigidity. Langmuir. 2016;32(24):6074–82. https://doi.org/10.1021/acs.langmuir.6b00741 .
Download references
Acknowledgments
This work was supported by the EU National Recovery and Resilience Plan CN3/Spoke 8 “National Center for Gene Therapy and Drugs based on RNA Technology” (Project #CN00000041).
Author information
Authors and affiliations.
Department of Industrial Engineering, University of Salerno, Via Giovanni Paolo II, 132, 84084, Fisciano, SA, Italy
Domenico Riccardi, Lucia Baldino & Ernesto Reverchon
You can also search for this author in PubMed Google Scholar
Contributions
Conceptualization: L.B. and D.R.; articles search: D.R.; writing: D.R.; reviewing and editing: L.B. and E.R.; All the authors read and approved the manuscript.
Corresponding author
Correspondence to Lucia Baldino .
Ethics declarations
Ethics approval and consent to participate, competing interests.
All the authors declare no competing interests.
Additional information
Publisher’s note.
Springer Nature remains neutral with regard to jurisdictional claims in published maps and institutional affiliations.
Rights and permissions
Open Access This article is licensed under a Creative Commons Attribution 4.0 International License, which permits use, sharing, adaptation, distribution and reproduction in any medium or format, as long as you give appropriate credit to the original author(s) and the source, provide a link to the Creative Commons licence, and indicate if changes were made. The images or other third party material in this article are included in the article’s Creative Commons licence, unless indicated otherwise in a credit line to the material. If material is not included in the article’s Creative Commons licence and your intended use is not permitted by statutory regulation or exceeds the permitted use, you will need to obtain permission directly from the copyright holder. To view a copy of this licence, visit http://creativecommons.org/licenses/by/4.0/ . The Creative Commons Public Domain Dedication waiver ( http://creativecommons.org/publicdomain/zero/1.0/ ) applies to the data made available in this article, unless otherwise stated in a credit line to the data.
Reprints and permissions
About this article
Cite this article.
Riccardi, D., Baldino, L. & Reverchon, E. Liposomes, transfersomes and niosomes: production methods and their applications in the vaccinal field. J Transl Med 22 , 339 (2024). https://doi.org/10.1186/s12967-024-05160-4
Download citation
Received : 30 December 2023
Accepted : 03 April 2024
Published : 09 April 2024
DOI : https://doi.org/10.1186/s12967-024-05160-4
Share this article
Anyone you share the following link with will be able to read this content:
Sorry, a shareable link is not currently available for this article.
Provided by the Springer Nature SharedIt content-sharing initiative
- Transfersomes
- Immune response
- Supercritical carbon dioxide
Journal of Translational Medicine
ISSN: 1479-5876
- Submission enquiries: Access here and click Contact Us
- General enquiries: [email protected]
Help | Advanced Search
Computer Science > Computation and Language
Title: researchagent: iterative research idea generation over scientific literature with large language models.
Abstract: Scientific Research, vital for improving human life, is hindered by its inherent complexity, slow pace, and the need for specialized experts. To enhance its productivity, we propose a ResearchAgent, a large language model-powered research idea writing agent, which automatically generates problems, methods, and experiment designs while iteratively refining them based on scientific literature. Specifically, starting with a core paper as the primary focus to generate ideas, our ResearchAgent is augmented not only with relevant publications through connecting information over an academic graph but also entities retrieved from an entity-centric knowledge store based on their underlying concepts, mined and shared across numerous papers. In addition, mirroring the human approach to iteratively improving ideas with peer discussions, we leverage multiple ReviewingAgents that provide reviews and feedback iteratively. Further, they are instantiated with human preference-aligned large language models whose criteria for evaluation are derived from actual human judgments. We experimentally validate our ResearchAgent on scientific publications across multiple disciplines, showcasing its effectiveness in generating novel, clear, and valid research ideas based on human and model-based evaluation results.
Submission history
Access paper:.
- Other Formats
References & Citations
- Google Scholar
- Semantic Scholar
BibTeX formatted citation

Bibliographic and Citation Tools
Code, data and media associated with this article, recommenders and search tools.
- Institution
arXivLabs: experimental projects with community collaborators
arXivLabs is a framework that allows collaborators to develop and share new arXiv features directly on our website.
Both individuals and organizations that work with arXivLabs have embraced and accepted our values of openness, community, excellence, and user data privacy. arXiv is committed to these values and only works with partners that adhere to them.
Have an idea for a project that will add value for arXiv's community? Learn more about arXivLabs .

An official website of the United States government
The .gov means it’s official. Federal government websites often end in .gov or .mil. Before sharing sensitive information, make sure you’re on a federal government site.
The site is secure. The https:// ensures that you are connecting to the official website and that any information you provide is encrypted and transmitted securely.
- Publications
- Account settings
Preview improvements coming to the PMC website in October 2024. Learn More or Try it out now .
- Advanced Search
- Journal List
- J Pharm Bioallied Sci
- v.4(Suppl 1); 2012 Mar
Preparation and formulation of transferosomes containing an antifungal agent for transdermal delivery: Application of Plackett-Burman design to identify significant factors influencing vesicle size
Rutu b. patel.
Ramanbhai Patel College of Pharmacy, Charotar University of Science and Technology, Changa, Gujarat, India
R. H. Parikh
Transferosomes containing an anti-fungal agent were prepared by Rotary Flask Evaporation –Sonication method. Eight batches were prepared in triplicate and vesicle size of each batch was determined. Plackett-Burman Design was employed to identify significant formulation and process parameters affecting vesicle size. The amount of lipid and surfactant, volume of ethanol and hydration medium as well as hydration time significantly affect the vesicle size.
Transferosomes are also known as Ultradeformable vesicles, Ultradeformable Liposomes or Elastic Vesicles. Transfersome can squeeze itself through a skin pore which is many times smaller than its size owing to its elasticity. Thus, they can increase the skin penetration when applied non-occlusively. The objective of the present study was to identify significant factors influencing vesicle size through the use of Plackett-Burman Design.
Materials and Methods
Preparation of transferosomes.
They were prepared by Rotary Flask Evaporation –Sonication Method reported by Gregor et al .[ 1 ] Soya phosphatidyl choline, Tween80 and antifungal agent were dissolved in Ethanol. Ethanol was evaporated by flask rotation to deposit the film on the wall of the round bottom flask. The film was hydrated at 45°C using Saline phosphate buffer, pH 6.4 with mild agitation. The Transferosomes formed were allowed to swell for 2 hour at room temperature. Vesicles were subsequently sonicated for 30minutes using bath sonicator. (Model: EN-60US,Make: ELECTROQUIP). Eight batches were prepared as per the Plackett-Burman Design [ Table 1 ].
Composition of transferosomes of different batches and their vesicle size

Measurement of vesicle size
Vesicle size was determined using particle size analyzer employing Dynamic Light Scattering Technique (Model: Nano S90, Make: Malvern, USA). Data analysis was carried out using DOE ++ Software (Version 1.0.7.2 ID –DS-1, ReliaSoft Corporation, USA).
Result and Discussion
Using Pareto chart [ Figure 1 ] significant and non-significant factors influencing vesicle size were identified. It is evident from the Pareto Chart [ Figure 1 ] as well as P value [ Table 1 ] with respect to vesicle size associated with every variable that the amount of Lipid, amount of Surfactant, volume of Ethanol, volume of Hydration medium and Hydration Time significantly affect the vesicle size.

Pareto chart showing effects of variables on vesicle size
From large number of formulation and process variables involved in making of transferosomes, Plackett-Burman Design can help in identifying significant factors influencing vesicle size. These significant factors may be optimized by employing full factorial design. While employing full factorial design, non-significant factors may be fixed at appropriate level (-1 or +1) employed during Plackett-Burman Design.
Acknowledgement
We are very thankful to Halcyon Labs Pvt. Ltd., Ahmedabad for providing an ANTIFUNGAL AGENT as a gratis sample.
Source of Support: Nil
Conflict of Interest: None declared.
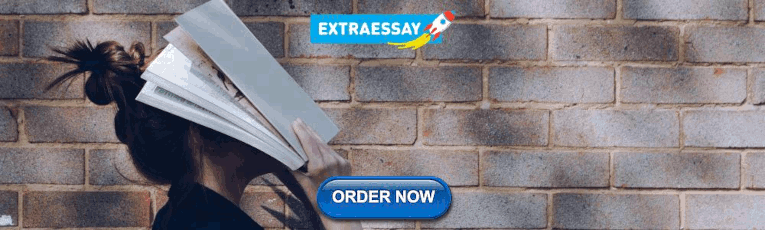
IMAGES
VIDEO
COMMENTS
Transfersomes are ultra-deformable carriers that facilitate the delivery of a diverse array of drug molecules across the skin barrier with superior efficacy compared to the conventional vesicular systems. The osmotic gradient is the main driving force for the transport of transfersomes into the deeper skin layers.
PDF | On May 22, 2013, Preetesh Ashokkumar Mishra and others published TRANSFERSOMES: A PROMISING APPROACH FOR TRANSDERMAL DRUG DELIVERY SYSTEM | Find, read and cite all the research you need on ...
Open Access Review Art icle. Transfersomes: a novel technique for tr ansdermal drug delivery. Priyanka Chaurasiya 1*, Eisha Ganju, Neeraj Upm anyu, Sudhir Kumar Ray, Prabhat Jain. School of ...
Transdermal delivery system has gained significance in drug delivery owing to its advantages over the conventional delivery systems. However, the barriers of stratum corneum along with skin irritation are its major limitations. Various physical and chemical techniques have been employed to alleviate these impediments. Among all these, transfersomes have shown potential for overcoming the ...
Transferosomes are said to have a number of applications like delivery of vaccines,proteins, Anti-cancer drugs,anesthetics,herbal drugs and has better patient compliance,improved bio-availability ...
Transdermal delivery systems have gained much interest in recent years owing to their advantages compared to conventional oral and parenteral delivery systems. They are noninvasive and self-administered delivery systems that can improve patient compliance and provide a controlled release of the therapeutic agents. The greatest challenge of transdermal delivery systems is the barrier function ...
A new type of carrier system—namely, transfersomes—were introduced by Cevc et al. in the 1990s. Transfersomes are composed of phospholipids and edge activator (EA), which is a membrane-softening agent (such as Tween 80, Span 80 and sodium cholate) that facilitates the ultra-deformable property of the transfersomes.
Transfersomes are elastic in nature, which can deform and squeeze themselves as an intact vesicle through narrow pores that are significantly smaller than its size. This review aims to describe the concept of transfersomes, the mechanism of action, different methods of preparation and characterization and factors affecting the properties of ...
Transferosomes, also known as transfersomes, are ultradeformable vesicles for transdermal applications consisting of a lipid bilayer with phospholipids and an edge activator and an ethanol/aqueous core. Depending on the lipophilicity of the active substance, it can be encapsulated within the core or amongst the lipid bilayer. Compared to liposomes, transferosomes are able to reach intact ...
The aim of current study was to develop the transdermal transfersomes of glucosamine for better drug delivery. Stretch ability and plasticity of transfersomes membranes mitigate the risk of ...
Areas covered: This article covers information such as merits/demerits of transfersomes, regulatory aspects of materials used in preparation, different methods of preparation, mechanism of action, review of clinical investigations performed, marketed preparations available, research reports, and patent reports related to transfersomes. Expert ...
Areas covered: This article covers information such as merits/demerits of transfersomes, regulatory aspects of materials used in preparation, different methods of preparation, mechanism of action, review of clinical investigations performed, marketed preparations available, research reports, and patent reports related to transfersomes. Expert ...
Article Views are the COUNTER-compliant sum of full text article downloads since November 2008 (both PDF and HTML) across all institutions and individuals. These metrics are regularly updated to reflect usage leading up to the last few days. Citations are the number of other articles citing this article, calculated by Crossref and updated daily.
Article Development of Stable Nano-Sized Transfersomes as a Rectal Colloid for Enhanced Delivery of Cannabidiol Thope Moqejwa, Thashree Marimuthu , Pierre P. D. Kondiah and Yahya E. Choonara * Wits Advanced Drug Delivery Platform Research Unit, Department of Pharmacy and Pharmacology, Faculty of
View PDF PDF; Abstract. The main object of this current research was to examine transferosomes as a transdermal delivery system for insulin, to overwhelm the difficulties related with its subcutaneous delivery. ... Formulation of insulin loaded transfersomes. Transferosomes were fabricated by using conventional rotary evaporation sonication ...
Transfersome is a ter m registered as a trademark by the. German company IDE A AG, and used b y it to refer to. its proprietary drug delivery technology. The name. means "carrying body", and ...
Transfersomes display deformable properties that improve the stability, penetration, elasticity, and delivery of active pharmaceutical ingredients such as phytochemicals, vaccines, and antineoplastic agents to deeper layers of the skin. The primary factor for the transfer of transfersomes into the inner epidermal layers is the osmotic gradient.
Transferosomes are said to have a number of applications like delivery of vaccines,proteins, Anti-cancer drugs,anesthetics,herbal drugs and has better patient compliance,improved bio-availability and site-specific delivery and can serve as an emerging tool for transdermal delivery of almost all drugs and bio-actives.
transferosomal drug deliver y systems are discussed i n this paper. Conclusion: Transferosomes have several importance over other vesicular systems, including. greater deformabilit y, greater ...
One of the most effective strategies to fight viruses and handle health diseases is vaccination. Recent studies and current applications are moving on antigen, DNA and RNA-based vaccines to overcome the limitations related to the conventional vaccination strategies, such as low safety, necessity of multiple injection, and side effects. However, due to the instability of pristine antigen, RNA ...
View PDF Abstract: Scientific Research, vital for improving human life, is hindered by its inherent complexity, slow pace, and the need for specialized experts. To enhance its productivity, we propose a ResearchAgent, a large language model-powered research idea writing agent, which automatically generates problems, methods, and experiment designs while iteratively refining them based on ...
2012 Article in press. [Google Scholar] 2. Singh Hardevinder Pal, P.U, Tiwary Ashok Kumar, Jain Subheet. ... Transfersomes. Biochimica et Biophysica Acta. 2001; 1514:191-205. [Google Scholar] Articles from Journal of Pharmacy & Bioallied Sciences are provided here courtesy of Wolters Kluwer -- Medknow Publications ...
Transfersomes are particularly optimized, ultradeformable (ultraflexible) lipid supramolecular aggregates, which are able to penetrate the mammalian skin intact. Transfersome is a type of carrier ...