- Research article
- Open access
- Published: 26 July 2019
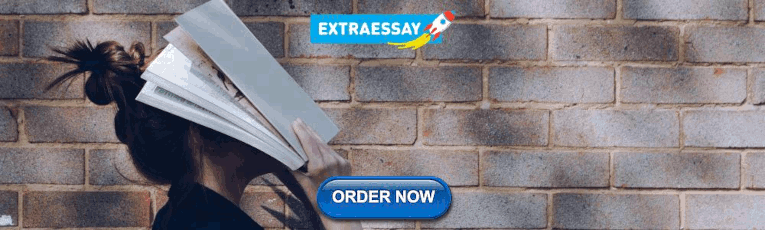
A prospective study of clinical characteristics and outcomes of acute kidney injury in a tertiary care Centre
- Su Hooi Teo 1 ,
- Kian-Guan Lee 1 ,
- Riece Koniman 1 ,
- Alvin Ren Kwang Tng 1 ,
- Zhong Hong Liew 1 ,
- Thin Thiri Naing 2 ,
- Huihua Li 2 , 3 ,
- Ru Yu Tan 1 ,
- Han Khim Tan 1 ,
- Hui Lin Choong 1 ,
- W. Y. Marjorie Foo 1 &
- Manish Kaushik 1
BMC Nephrology volume 20 , Article number: 282 ( 2019 ) Cite this article
5349 Accesses
13 Citations
1 Altmetric
Metrics details
Acute kidney injury (AKI) is a major global health problem. We aim to evaluate the epidemiology, risk factors and outcomes of AKI episodes in our single centre.
Methodology
We prospectively identified 422 AKI and acute on chronic kidney disease episodes in 404 patients meeting KDIGO definitions using electronic medical records and clinical data from 15th July to 22nd October 2016, excluding patients with baseline estimated GFR (eGFR) of < 15 mL/min. Patients were followed up till 6 months after AKI diagnosis.
The mean age was 65.8 ± 14.1. Majority of patients were male (58.2%) of Chinese ethnicity (68.8%). One hundred and thirty-two patients (32.6%) were diagnosed in acute care units. Seventy-five percent of patients developed AKI during admission in a non-Renal specialty. Mean baseline eGFR was 50.2 ± 27.7 mL/min. Mean creatinine at AKI diagnosis was 297 ± 161 μmol/L. Renal consultations were initiated at KDIGO Stages 1, 2 and 3 in 58.9, 24.5 and 16.6% of patients, respectively. Three hundred and ten (76.7%) patients had a single etiology of AKI with the 3 most common etiologies of AKI being pre-renal (27.7%), sepsis-associated (25.5%) and ischemic acute tubular necrosis (15.3%). One hundred and nine (27%) patients received acute renal replacement therapy. In-hospital mortality was 20.3%. Six-month mortality post-AKI event was 9.4%. On survival analysis, patients with KDIGO Stage 3 AKI had significantly shorter survival than other stages.
AKI is associated with significant in-hospital to 6-month mortality. This signifies the pressing need for AKI prevention, early detection and intervention in mitigating reversible risk factors in order to optimize clinical outcomes.
Peer Review reports
Acute kidney injury is one of the major complications in acutely ill patients and imposes significant mortality and morbidity globally [ 1 , 2 , 3 , 4 , 5 ]. AKI may be present on admission to hospital or develop during the course of hospitalization [ 6 ]. Based on the Kidney Disease, Improving Global Outcomes (KDIGO) report, the incidence of AKI in hospitalized patients ranges from 17 to 31% [ 7 , 8 , 9 ]. AKI-related inpatient care is also associated with increased healthcare costs due to prolonged hospitalizations, additional investigations and the development of complications such as the need for renal replacement therapy (RRT), cardiovascular complications and re-admissions [ 10 , 11 , 12 ]. The 2009 National Confidential Enquiry into Patient Outcomes and Death (NCEPOD) reported that 50% of patients who died from AKI received suboptimal care and 14% of AKI was avoidable [ 13 ]. In 2013, the International Society of Nephrology launched the 0by25 initiative of improving timely diagnosis and treatment of AKI globally with an aim to eliminate preventable deaths from AKI worldwide by 2025 [ 8 ]. In view of this, a considerably greater attention has been paid to Asian countries. The risk factors, myriad of etiologies and consequences of AKI have been well-delineated [ 6 , 14 , 15 ]. Given the diversity in culture, ethnicity, climate and socioeconomic status, it is not surprising that a difference in etiology, incidence and risk factors of AKI exists in various parts of Asia. The pooled- incidences of AKI in hospitalized patients in Asia vary from 9.0% in Central Asia to 31.0% in Southeastern Asia [ 7 ]. The development of AKI has been shown to progress to chronic kidney disease (CKD) and end stage renal disease (ESRD). To date, the data on acute kidney injury in Singapore is scattered. Therefore, we aimed to (i) analyze the distribution of AKI in different clinical units of adults admitted to a hospital in Singapore, and (ii) describe the clinical characteristics, risk profiles and outcomes of AKI.
Study population
Data on patients referred to the Nephrology Department in Singapore General Hospital (a 1785-bedded tertiary hospital) and diagnosed with AKI by KDIGO (2012) criteria from 15th July to 22nd October 2016 were prospectively collected from electronic medical records and clinical notes. Patients with estimated glomerular filtration rate (eGFR) of ≤15 mL/min were excluded. The study protocol was approved by the SingHealth Centralized Institutional Review Board (IRB). Patients were followed up till 6 months after AKI diagnosis.
We studied AKI according to the KDIGO 2012 AKI criteria, (i) increase in serum creatinine ≥26.5 μmol/L within 48 h, (ii) increase in serum creatinine ≥1.5x from baseline serum creatinine within the prior 7 days. Baseline serum creatinine was defined as the result on admission or the latest available serum creatinine within the preceding 12 months prior to admission, whichever available. Patients were included if serum creatinine fulfilled criteria for minimum KDIGO 2012 stage within 24 h of admission. Hypotension preceding diagnosis of AKI was defined as mean arterial pressure (MAP) of less than 70 mmHg or the use of inotropes or vasopressors.
Statistical analysis
Mean and standard deviation (SD) were reported for continuous variables, while frequency and proportion were reported for categorical data. Overall survival was defined from the date of AKI diagnosis to the date of death, or last follow-up date for censored cases. Overall survival was estimated by the Kaplan–Meier method. Log-rank test was used to compare survival curves. Univariable Cox regression was carried out to evaluate the effects of potential factors on overall survival. All the variables with p -value of < 0.2 by univariable Cox regression were included in the multivariable analysis. Multivariable model was built up by means of reduced model selection using Akaike’s information criterion (AIC). For all analyses, p value is taken as statistically significant when it is < 0.05. R 3.4.2 ( https://www.r-project.org ) was used for analysis.
Clinical characteristics of AKI patients
A total of 422 episodes of AKI in 404 patients were identified to have AKI. The clinical characteristics of the patients are shown in Table 1 . The severity of AKI was classified as KDIGO AKI Stage 1 in 238 (58.9%) patients; Stage 2 in 99 (24.5%) patients and Stage 3 in 67 (16.6%) patients. Among critically ill patients, 89 (22%) patients with AKI were detected whilst in Intensive Care Unit (ICU) and 43 (10.6%) in Intermediate Care Area (ICA) or High Dependency Units. Two hundred seventy-two (67.3%) patients developed AKI in the general wards, of which 67% were in Stage 1. In our study, 147 (36.1%) patients with AKI were detected in the medical departments, while 81 (20%) were detected in the cardiac units and 78 (19.3%) in the surgical departments. Ninety-eight (24.3%) patients with AKI were identified in the nephrology unit.
Of the 404 patients, 235 (58.2%) were male and 169 (41.8%) were female. The mean age was 65.8 ± 14.1 years, with 58.9% older than 65 years. The ethnicity distribution reflected that of our hospital population as a whole. Mean baseline serum creatinine was 150 ± 71 μmol/L, with a corresponding eGFR of 50 ± 27.7 mL/min. With regards to comorbidities, 302 (74.8%) had hypertension, 230 (56.9%) had diabetes mellitus, and 187 (46.3%) had ischemic heart disease. Seventy-eight cases (19.3%) of AKI occurred in the background of underlying malignancy. Mean serum creatinine at AKI diagnosis was 297.5 ± 160.7 μmol/L. The most frequent cause of AKI was noted to be pre-renal cause, with an occurrence in 112 (27.7%) patients, followed by sepsis-associated AKI and ischemic acute tubular necrosis (ATN) occurring in 105 (26%) and 62 (15.3%) patients, respectively. Dialysis was carried out in 109 (27%) of our AKI patients, of which 62 (56.9%) received continuous renal replacement therapy (CRRT).
Univariable analysis
The univariate analysis of risk factors associated with mortality for patients with AKI is shown in Table 2 . Elderly patients had a statistically significant shorter survival (HR 1.54, 95% 1.07–2.22, p = 0.0201). Malay ethnicity was noted to have a lower risk of mortality (HR 0.55, 95% CI 0.33–0.94, p = 0.0272). Baseline eGFR of > 60 mL/min was associated with a higher risk of mortality (HR 1.54, 95% CI 1.08–2.21, p = 0.0180). Renal transplant recipients had a lower risk of death (HR 0.11, 95% CI 0.03–0.46, p = 0.0023). Paradoxically, hypertension was found to be associated with a lower mortality in AKI (HR 0.5, 95% CI 0.35–0.72, p = 0.0002). Multifactorial AKI was also found to be associated with higher mortality (HR 1.81, 95% CI 1.25–2.61, p = 0.0017). Patients with hypernatremia had a lower survival (HR 2.31, 95% CI 1.21–4.38, p = 0.0180). Presence of hypotension in the preceding 48 h prior to occurrence of AKI was strongly associated with mortality (HR 3.35, 95% 2.18–5.13, p < 0.0001). There was almost a twofold increased risk of mortality in AKI Stage 3 (HR 1.88, 95% CI 1.23–2.88, p = 0.0034). AKI patients who required renal replacement therapy had a significantly higher mortality (HR 2.74, 95% CI 1.93–3.89, p < 0.001).
Multivariable analysis
In the multivariable analysis shown in Table 3 , the independent baseline variable that was significantly associated with mortality was age more than 65 (HR 1.46, 95% CI 1.00–2.13, p = 0.0483). Interestingly, AKI in the setting of renal transplant recipients (HR 0.17, 95% CI 0.04–0.70, p = 0.0143) and hypertension (HR 0.53, 95% CI 0.37–0.78, p = 0.0010) were significantly associated with a lower risk of mortality. Presence of hypotension in the preceding 48 h (HR 2.57, 95% CI 1.63–4.07, p = 0.0001) prior to development of AKI and AKI requiring dialysis (HR 1.67, 95% CI 1.14–2.44, p = 0.0084) were significantly associated with death.
Risk factors associated with mortality based on one episode of AKI per patient are presented in the Additional file 1 : Table S1 and Table S2.
Outcomes of AKI
The outcomes associated with AKI are shown in Table 4 . Median serum creatinine at discharge was 176 μmol/L (IQR 114-278 μmol/L). Upon discharge, 16 (4%) patients still required dialysis support. In-hospital mortality rate was 20.3% while 6-month mortality was 9.4%.
The survival rates at 3- and 6-months post-AKI diagnosis according to severity of AKI, are shown in Table 5 . AKI was associated with decreased survival according to severity of AKI stages at 3 months post-AKI episode (75.9, 95% CI 70.7–81.5% for Stage 1; 73.7, 95% CI 65.3–83.1% for Stage 2, and 57.4, 95% CI 46.7–70.4% for Stage 3). There were significant differences in survival between the severity of AKI stages (log-rank test p -value 0.0091).
At 6 months, AKI was associated with decreased survival with worsening severity of AKI stages: 71.4% (95% CI 65.9–77.3%) for Stage 1, 70.5% (95% CI 61.9–80.3%) for Stage 2, 54.4% (95% CI 43.8–67.6%) for Stage 3 (log-rank test p-value 0.0091). Fig. 1 shows the Kaplan-Meier estimated survival according to AKI stages. On survival analysis, patients with KDIGO Stage 1 and 2 AKI had significantly better survival than Stage 3 AKI.
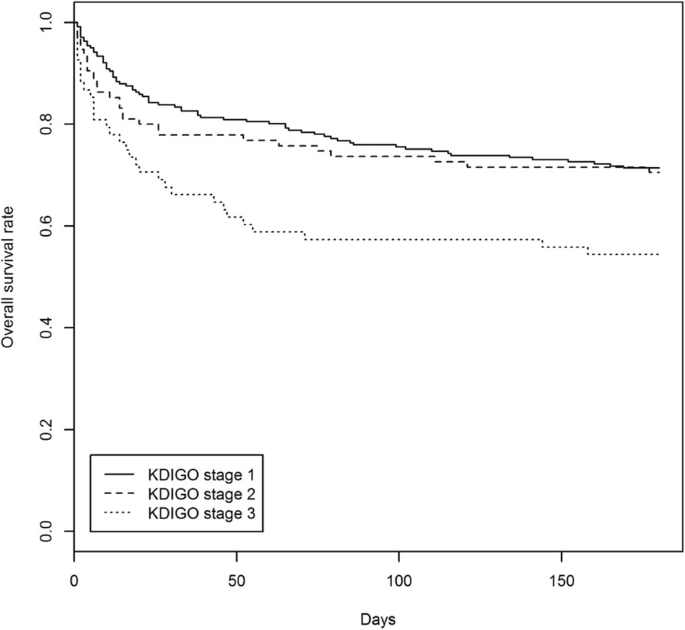
Kaplan-Meier estimated survival for severity of AKI. Log-rank test p-value = 0.0091
This study investigated a cohort of 404 patients admitted to a tertiary hospital over 100 days. Patients with AKI were predominantly identified in non-nephrology specialties, and nephrologists’ involvement started after consultation was requested. Sixty percent (60%) of referrals for AKI were at Stage 1 AKI at point of nephrology consult. We have demonstrated that AKI developed in 22% of critically ill patients. This is consistent with other studies showing incidence rate of AKI during ICU stays varying from 22 to 67% [ 16 , 17 , 18 , 19 ]. The epidemiological data, clinical features and etiology on AKI in Asian countries differ from that of what we found in our data, especially with regards to precipitants of AKI. To-date, there is a paucity of data from Singapore on clinical characteristics, etiologies and outcomes of patients with AKI. Our study was intended to give us an insight into this. Locally, Chua et al. evaluated 207 patients with septic AKI and mortality in Singapore and reported that a 1-year mortality rate of 40%, with high daily fluid balance and frusemide administration being modifiable risk factors [ 20 ]. In terms of cardiac surgery-associated AKI in South-East Asian population, Chew et al. reported that 35.3% of patients developed AKI after cardiac surgery, with Indian and Malay ethnicity having a higher risk than Chinese ethnicity [ 21 ]. Our study provides novel facts on the major affected clinical settings of AKI, clinical characteristics, risk factors and outcomes associated with AKI across different etiological insults.
We identified pre-renal cause as a precipitant of AKI in 27.7%, a lower proportion compared to a study by Tang et al. reporting pre-renal cause of AKI of 49.1% from the medical departments [ 22 ]. Volume resuscitation to replace ongoing losses and restoration to baseline volume status is crucial in the event of true extracellular fluid volume depletion. Knowledge of baseline weight, careful attention to intake and output and ongoing serial weight measurement and volume status assessment directs the strategy for resuscitation [ 23 , 24 ].
In our centre, 27% of AKI patients received acute renal replacement therapy (RRT), of which 56.9% received CRRT. Our finding was relatively consistent with a study by Yang et al. reporting that 22.5% of patients received RRT [ 25 ]. The initiation of RRT in patients with severe AKI contributes to healthcare costs and is a measure of complexity of care in critically ill patients. The practice of prescription of CRRT therapy varies widely across different continents. In a retrospective analysis of 261 patients in 2 ICUs in Canada estimating the cost comparison between CRRT and intermittent hemodialysis, the weekly cost ranged from 3,486 to 5,117 Canadian dollars (depending on modality choice and anticoagulation) [ 26 ]. This cost was significantly more expensive than intermittent hemodialysis at a weekly cost of 1,342 Canadian dollars. The frequency of dialysis requirement in AKI ranges from 6.9% in Asia to 71% in the study by Beginning and Ending Supportive Therapy for the Kidney (BEST Kidney) Investigators [ 19 ] [ 27 ],
Acute kidney injury remains a common clinical problem, particularly in the elderly. Liano et al. reported a mean age of 64 years in their study involving AKI episodes occurring in adult patients admitted to any of the 13 tertiary care hospitals in Madrid [ 28 ]. The mean age of patients was 73 in two UK district hospitals reported by Meran et al [ 29 ] and 64.7 in a separate Canadian-based ICU study [ 30 ]. Similarly, patients in our study had a median age of 65.8 years. We demonstrated that patients with AKI who were 65 years old and above were associated with higher risks of mortality. Age older than 65 is not only a risk factor for impaired recovery from AKI and progression to advanced-stage CKD, but the long-term survival of patients with AKI worsens with increasing age, even in non-dialysis requiring AKI [ 31 , 32 , 33 ]. In the setting of reduced renal reserve in the elderly [ 34 ], the higher frequency of nephrotoxins usage such as non-steroidal anti-inflammatory drugs predisposes these patients to AKI [ 34 ]. The incidence of AKI in the elderly population is expected to be on the rise given the challenges of the elderly population in the nation, along with an interplay of polypharmacy and susceptibility to nephrotoxic agents in the aged population.
In previously reported studies on transplant AKI epidemiology, the diagnosis of AKI was based on RIFLE criteria [ 35 ]. In our study, we analyzed the incidence of AKI meeting the definition in KDIGO 2012. Mehrotra et al. included 27, 232 kidney transplant recipients of which 11.3% developed AKI during the study period and it was reported that patients who developed AKI had an increased risk of death (HR 2.36, 92% CI 2.41–2.60) [ 36 ]. However, hazard ratios for both outcomes of graft loss and death were inversely related to the severity of CKD. In our study, AKI in renal transplant recipients was associated with a lower risk of mortality paradoxically. The subgroup of the renal transplant recipients in our study was younger and had a baseline eGFR > 30 mL/min. Sepsis-associated AKI (SA-AKI) was the leading precipitant of AKI in renal transplant recipients, with the majority not requiring dialysis. Care of renal transplant recipients in our institution is provided by a dedicated renal transplant team, and any SA-AKI was aggressively managed with input from transplant infectious disease specialists. These factors may potentially contribute to a significantly lower risk of mortality in this group of AKI patients but have yet to be validated.
The identification of risk factors predicting risk of mortality is imperative so that early mitigating factors can be implemented. Many studies have attempted to identify prognostic factors in predicting AKI in critically ill patients [ 37 , 38 ]. Previous reports have demonstrated that oliguria, pre-existing CKD, AKI attributable to nephrotoxic agents, AKI severity, and multi-organ failure were significant independent risk factors for death in AKI patients. In the Madrid Acute Renal Failure Study Group by Liano et al., oliguria, sustained hypotension, assisted respiration and icterus were associated with higher mortality [ 28 ]. Interestingly in our study, an underlying comorbidity of hypertension was not significantly associated with mortality. This finding may potentially be related to the counter-effect of hypertension against ischemic insult during an episode of AKI and it remains to be validated in future studies.
Hemodynamic instability is one of the most common causes of acute kidney injury. Our understanding of kidneys receiving about 25% of our cardiac output, allows us to target adequate renal perfusion as a potential strategy to modify the risk of developing AKI. Although the mean arterial pressure (MAP) target of ≥65 mmHg was defined in the Surviving Sepsis Campaign Guideline 2018, a recent study by Saito and colleagues had measured hemodynamic pressure-related parameters comparing between patients with progression of AKI versus those without AKI progression [ 39 ]. The authors measured hemodynamic pressure-related parameters including systolic arterial pressure (SAP), diastolic arterial pressure (DAP), MAP and central venous pressure (CVP), mean perfusion pressure (MPP) and diastolic perfusion pressure (DPP) and calculated deficits in the above values. The study observed a significant difference in the DPP, MPP and DAP in the patients with AKI progression, and suggested that these deficits may potentially be modifiable risk factors for the prevention of AKI progression, particularly in the patients who had undergone cardiac surgery. In our study, we found that AKI patients with hypotension in the preceding 48 h prior to development of AKI were reported to be strongly associated with mortality. Silva et al. reported a similar finding of hypotension being an independent risk factor for death in the intensive care units [ 40 ].
The risk of mortality with severe AKI requiring RRT remains high, particularly in the setting of critical illness, estimated to be approaching 60% [ 41 , 42 ]. Factors such as AKI stage, severity of acute non-renal organ dysfunction and underlying diagnosis were associated with increased risk for mortality after AKI [ 43 ]. Our study showed that 109 patients (27%) required RRT during admission. Hsu et al. also demonstrated that the incidence of dialysis-requiring AKI had been escalating rapidly, averaging at 10% annually in the United States with similar trends observed worldwide [ 44 , 45 ]. An episode of dialysis-requiring AKI was a strong independent risk factor for long-term risk of progressive CKD and mortality [ 46 ]. Our finding of AKI-requiring dialysis as an independent predictor for mortality is consistent with previous literature [ 46 , 47 , 48 ].
The major strengths of our study are the detailed evaluation of the risk factors and the distribution of AKI within clinical departments. However, our findings should be interpreted in light of the following limitations. The definition of AKI used in our study was based on serum creatinine change unaccompanied by urinary output, hence leading to underestimation of the detection rate of AKI. Secondly, the study may run an inherent risk of sampling bias as it was conducted over a snapshot period. Thirdly, as the baseline serum creatinine method has not been well-unified, method such as using the minimum value of preadmission serum creatinine as a baseline creatinine has been shown to identify more patients with AKI and yield better predictive ability for 60-day mortality [ 49 ]. Multiple AKI biomarkers that are measured in the urine or plasma of patients with AKI have been discovered, including the neutrophil gelatinase-associated lipocalin (NGAL), kidney injury molecule 1 (KIM-1), liver-type fatty acid-binding protein (L-FABP), interleukin 18 (IL-18), calprotectin, urine angiotensinogen (AGT), urine microRNAs and the recently FDA-approved insulin-like growth factor-binding protein 7 x tissue inhibitor of metalloproteinase 2 in the USA [ 50 ]. Biomarkers for AKI diagnosis are not currently being used routinely in our local clinical practice, hence our study did not include any novel biomarkers for AKI diagnosis. In our future research, we hope to leverage the relationship of biomarkers in diagnosing AKI and predicting short and long-term outcomes of acute kidney injury in different patient care settings, given the heterogeneity of this condition. Finally, this study did not include the long-term outcomes of patient survival and the risk of ESRD after 6 months.
In conclusion, our study shows that AKI resulted in an in-hospital mortality of 20.3%. Additionally, the AKI survivors had a mortality risk of 9.4% at 6 months. Risk factors including age above 65, presence of hypotension in the preceding 48 h prior to the development of AKI and AKI requiring dialysis were significantly associated with mortality. The data we have presented will enable policies to be drawn and healthcare costs to be quantified. Thus, these findings highlight the urgent need to develop effective treatments, explore educational opportunities pertaining to AKI, and improve hospital-based care processes aimed at early identification to prevent devastating outcomes.
Availability of data and materials
All datasets generated and/or analysed during the current study are not publicly available due to confidentiality of the data but are available from the corresponding author on reasonable request.
Abbreviations
Akaike’s information criterion
Acute Kidney Injury
Acute tubular necrosis
Confidence interval
Chronic kidney disease
Continuous renal replacement therapy
Diastolic arterial pressure
Diastolic perfusion pressure
Estimated glomerular filtration rate
End stage renal disease
Hazard ratio
Intermediate Care Area
Intensive Care Unit
Institutional Review Board
Kidney Disease, Improving Global Outcomes
Mean arterial pressure
Mean perfusion pressure
National Confidential Enquiry into Patient Outcomes and Death
Risk Injury, Failure, Loss of kidney function, End-stage kidney disease
Renal replacement therapy
Sepsis-associated acute kidney injury
Systolic arterial pressure
Standard deviation
Chawla LS, Eggers PW, Star RA, Kimmel PL. Acute kidney injury and chronic kidney disease as interconnected syndromes. N Engl J Med. 2014;371(1):58–66.
Article Google Scholar
Bellomo R, Kellum JA, Ronco C. Acute kidney injury. Lancet. 2012;380(9843):756–66.
Ali T, Khan I, Simpson W, Prescott G, Townend J, Smith W, et al. Incidence and outcomes in Acute kidney injury: a comprehensive population-based study. J Am Soc Nephrol. 2007;18(4):1292–8.
Article CAS Google Scholar
Wald R, Quinn RR, Adhikari NK, Burns KE, Friedrich JO, Garg AX, et al. Risk of chronic Dialysis and death following Acute kidney injury. Am J Med. 2012;125(6):585–93.
Hoste EAJ, Bagshaw SM, Bellomo R, Cely CM, Colman R, Cruz DN, et al. Epidemiology of acute kidney injury in critically ill patients: the multinational AKI-EPI study. Intensive Care Med. 2015;41(8):1411–23.
Bedford M, Stevens PE, Wheeler TWK, Farmer CKT. What is the real impact of acute kidney injury? BMC Nephrol. 2014;15(1):95.
Susantitaphong P, Cruz DN, Cerda J, Abulfaraj M, Alqahtani F, Koulouridis I, et al. World incidence of AKI: a meta-analysis. Clin J Am Soc Nephrol. 2013;8(9):1482–93.
Mehta RL, Cerdá J, Burdmann EA, Tonelli M, García-García G, Jha V, et al. International Society of Nephrology’s 0by25 initiative for acute kidney injury (zero preventable deaths by 2025): a human rights case for nephrology. Lancet 2015 J;385(9987):2616–2643.
Bouchard J, Mehta RL. Acute Kidney Injury in Western Countries. Kidney Dis (Basel, Switzerland). 2016;2(3):103–10.
Google Scholar
Chertow GM, Burdick E, Honour M, Bonventre JV, Bates DW. Acute kidney injury, mortality, length of stay, and costs in hospitalized patients. J Am Soc Nephrol. 2005;16(11):3365–70.
Silver SA, Chertow GM. The economic consequences of Acute kidney injury. Nephron. 2017;137(4):297–301.
Kerr M, Bedford M, Matthews B, O’Donoghue D. The economic impact of acute kidney injury in England. Nephrol Dial Transplant. 2014;29(7):1362–8.
Sterwart J, Findlay G, Smith N, Kelly K, Mason M. Acute kidney injury: adding insult to injury. Natl Confid Enq into Patient Outcomes Death. 2009.
Mehta RL, Pascual MT, Soroko S, Savage BR, Himmelfarb J, Ikizler TA, et al. Spectrum of acute renal failure in the intensive care unit: the PICARD experience. Kidney Int. 2004;66(4):1613–21.
Uchino S, Kellum JA, Bellomo R, Doig GS, Morimatsu H, Morgera S, et al. Acute renal failure in critically ill patients: a multinational, multicenter study. JAMA. 2005;294(7):813–8.
Hoste EA, Clermont G, Kersten A, Venkataraman R, Angus DC, De Bacquer D, et al. RIFLE criteria for acute kidney injury are associated with hospital mortality in critically ill patients: a cohort analysis. Crit Care. 2006;10(3):R73.
Thakar CV, Christianson A, Freyberg R, Almenoff P, Render ML. Incidence and outcomes of acute kidney injury in intensive care units: a veterans administration study*. Crit Care Med. 2009;37(9):2552–8.
Srisawat N, Sileanu FE, Murugan R, Bellomod R, Calzavacca P, Cartin-Ceba R, et al. Variation in risk and mortality of acute kidney injury in critically ill patients: a multicenter study. Am J Nephrol. 2015;41(1):81–8.
Trongtrakul K, Sawawiboon C, Wang AY, Chitsomkasem A, Limphunudom P, Kurathong S, et al. Acute kidney injury in critically ill surgical patients: epidemiology, risk factors and outcomes. Nephrology (Carlton) 2017. doi: https://doi.org/10.1111/nep.13192 . [Epub ahead of print].
Chua H-R, Wong W-K, Ong VH, Agrawal D, Vathsala A, Tay H-M, et al. Extended mortality and chronic kidney disease after septic Acute kidney injury. J Intensive Care Med 2018 Jan 1:885066618764617. doi: https://doi.org/10.1177/0885066618764617 . [Epub ahead of print].
Chew STH, Mar WMT, Ti LK. Association of ethnicity and acute kidney injury after cardiac surgery in a south east Asian population. Br J Anaesth. 2013;110(3):397–401.
Tang X, Chen D, Yu S, Yang L, Mei C, ISN AKF 0 by 25 China Consortium. Acute kidney injury burden in different clinical units: Data from nationwide survey in China. Sun J, editor. PLoS One 2017;12(2):e0171202.
Yamout H, Levin ML, Rosa RM, Myrie K, Westergaard S. Physician prevention of Acute kidney injury. Am J Med. 2015;128(9):1001–6.
Cooper CM, Fenves AZ. Before you call renal: Acute kidney injury for hospitalists. J Hosp Med. 2015;10(6):403–8.
Yang F, Zhang L, Wu H, Zou H, Du Y. Clinical analysis of cause, treatment and prognosis in acute kidney injury patients. Musabayane CT, editor. PLoS One 2014;9(2):e85214.
Manns B, Doig CJ, Lee H, Dean S, Tonelli M, Johnson D, et al. Cost of acute renal failure requiring dialysis in the intensive care unit: clinical and resource implications of renal recovery*. Crit Care Med. 2003;31(2):449–55.
Bagshaw SM, Uchino S, Bellomo R, Morimatsu H, Morgera S, Schetz M, et al. Septic Acute kidney injury in critically ill patients: clinical characteristics and outcomes. Clin J Am Soc Nephrol. 2007;2(3):431–9.
Liaño F, Pascual J. Epidemiology of acute renal failure: a prospective, multicenter, community-based study. Madrid Acute Renal Failure study group. Kidney Int. 1996;50(3):811–8.
Meran S, Wonnacott A, Amphlett B, Phillips A. How good are we at managing acute kidney injury in hospital? Clin Kidney J. 2014;7(2):144–50.
Odutayo A, Adhikari NKJ, Barton J, Burns KEA, Friedrich JO, Klein D, et al. Epidemiology of acute kidney injury in Canadian critical care units: a prospective cohort study. Can J Anesth Can d’anesthésie. 2012;59(10):934–42.
Anderson S, Eldadah B, Halter JB, Hazzard WR, Himmelfarb J, Horne FM, et al. Acute kidney injury in older adults. J Am Soc Nephrol. 2011;22(1):28–38.
Schmitt R, Coca S, Kanbay M, Tinetti ME, Cantley LG, Parikh CR. Recovery of kidney function after acute kidney injury in the elderly: a systematic review and meta-analysis. Am J Kidney Dis. 2008;52(2):262–71.
Cerda J, Lameire N, Eggers P, Pannu N, Uchino S, Wang H, et al. Epidemiology of Acute kidney injury. Clin J Am Soc Nephrol. 2008;3(3):881–6.
Musso CG, Reynaldi J, Martinez B, Pierángelo A, Vilas M, Algranati L. Renal reserve in the oldest old. Int Urol Nephrol. 2011;43(1):253–6.
Nakamura M, Seki G, Iwadoh K, Nakajima I, Fuchinoue S, Fujita T, et al. Acute kidney injury as defined by the RIFLE criteria is a risk factor for kidney transplant graft failure. Clin Transpl. 2012;26(4):520–8.
Mehrotra A, Rose C, Pannu N, Gill J, Tonelli M, Gill JS. Incidence and consequences of Acute kidney injury in kidney transplant recipients. Am J Kidney Dis. 2012;59(4):558–65.
Perez Valdivieso JR, Bes-Rastrollow M, Monedero P, De Irala J, La Villa FJ. Evaluation of the prognostic value of the risk, injury, failure, loss and end-stage renal failure (RIFLE) criteria for acute kidney injury. Nephrology. 2008;13(5):361–6.
Cruz DN, Bolgan I, Perazella MA, Bonello M, de Cal M, Corradi V, et al. North east Italian prospective hospital Renal outcome survey on Acute kidney injury (NEiPHROS-AKI): targeting the problem with the RIFLE criteria. Clin J Am Soc Nephrol. 2007;2(3):418–25.
Saito S, Uchino S, Takinami M, Uezono S, Bellomo R. Postoperative blood pressure deficit and acute kidney injury progression in vasopressor-dependent cardiovascular surgery patients. Crit Care. 2016;20(1):74.
da Silva Júnior GB, Daher EDF, Mota RMS, Menezes FA. Risk factors for death among critically ill patients with acute renal failure. Sao Paulo Med J. 2006;124(5):257–63.
A. Molitoris B, J. Meier D, Wang E, M. Sandoval R, Sheridan E, S. Strickland J. Quantifying glomerular filtration rates: kidney function analysis method and apparatus. Recent Patents Biomarkerse 2012 Aug;2(3):209–18.
VA/NIH Acute Renal Failure Trial Network, Palevsky PM, Zhang JH, O’Connor TZ, Chertow GM, Crowley ST, et al. Intensity of Renal support in critically ill patients with Acute kidney injury. N Engl J Med. 2008;359(1):7–20.
Clec’h C, Gonzalez F, Lautrette A, Nguile-Makao M, Garrouste-Orgeas M, Jamali S, et al. Multiple-center evaluation of mortality associated with acute kidney injury in critically ill patients: a competing risks analysis. Crit Care. 2011;15(3):R128.
Hsu RK, McCulloch CE, Dudley RA, Lo LJ, Hsu CY. Temporal changes in incidence of Dialysis-requiring AKI. J Am Soc Nephrol. 2013;24(1):37–42.
Hoste EAJ, Schurgers M. Epidemiology of acute kidney injury: how big is the problem? Crit Care Med. 2008;36(4 Suppl):S146–51.
Lo LJ, Go AS, Chertow GM, McCulloch CE, Fan D, Ordoñez JD, et al. Dialysis-requiring acute renal failure increases the risk of progressive chronic kidney disease. Kidney Int. 2009;76(8):893–9.
Schiffl H, Lang SM, Fischer R. Long-term outcomes of survivors of ICU acute kidney injury requiring renal replacement therapy: a 10-year prospective cohort study. Clin Kidney J. 2012;5(4):297–302.
Sakhuja A, Kumar G, Gupta S, Mittal T, Taneja A, Nanchal RS. Acute kidney injury requiring Dialysis in severe Sepsis. Am J Respir Crit Care Med. 2015;192(8):951–7.
Thongprayoon C, Cheungpasitporn W, Kittanamongkolchai W, Srivali N, Ungprasert P, Kashani K. Optimum methodology for estimating baseline serum creatinine for the acute kidney injury classification. Nephrology. 2015;20(12):881–6.
Kashani K, Cheungpasitporn W, Ronco C. Biomarkers of acute kidney injury: the pathway from discovery to clinical adoption. Clin Chem Lab Med. 2017;55(8):1074–89.
Download references
Acknowledgements
Not applicable.
The authors have no funding sources to declare.
Author information
Authors and affiliations.
Department of Renal Medicine, Academia, Singapore General Hospital, 20 College Road, Singapore, 169856, Singapore
Su Hooi Teo, Kian-Guan Lee, Riece Koniman, Alvin Ren Kwang Tng, Zhong Hong Liew, Ru Yu Tan, Han Khim Tan, Hui Lin Choong, W. Y. Marjorie Foo & Manish Kaushik
Health Services Research Unit, Bachelor of Nursing, University of Sydney, Singapore General Hospital, Singapore, Singapore
Thin Thiri Naing & Huihua Li
Centre for Quantitative Medicine, Duke-NUS Medical School, Singapore, Singapore
You can also search for this author in PubMed Google Scholar
Contributions
MK/SHT/ CHL/ HKT/ WYMF designed the research; MK/SHT/ KGL performed research; SHT/ KGL/ KR/ TA/ ZHL/ TTN collected the data; LH/ SHT/ KGL / RYT analyzed the data; All authors contributed in authorship of the manuscript.
Corresponding author
Correspondence to Su Hooi Teo .
Ethics declarations
Ethics approval and consent to participate.
The study protocol was approved by the SingHealth Centralized Institutional Review Board (IRB). The requirement for written consent was waived as no interventions or any additional investigations were required for the study purposes.
Consent for publication
Competing interests.
All authors have declared that they have no competing interests.
Additional information
Publisher’s note.
Springer Nature remains neutral with regard to jurisdictional claims in published maps and institutional affiliations.
Additional file
Additional file 1:.
Table S1. Univariable Analysis of Risk Factors associated with Mortality for Patients with AKI (based on one episode of AKI per patient). Table S2. Multivariable Analysis of Risk Factors associated with Mortality for Patients with AKI (based on one episode of AKI per patient). (DOCX 22 kb)
Rights and permissions
Open Access This article is distributed under the terms of the Creative Commons Attribution 4.0 International License ( http://creativecommons.org/licenses/by/4.0/ ), which permits unrestricted use, distribution, and reproduction in any medium, provided you give appropriate credit to the original author(s) and the source, provide a link to the Creative Commons license, and indicate if changes were made. The Creative Commons Public Domain Dedication waiver ( http://creativecommons.org/publicdomain/zero/1.0/ ) applies to the data made available in this article, unless otherwise stated.
Reprints and permissions
About this article
Cite this article.
Teo, S.H., Lee, KG., Koniman, R. et al. A prospective study of clinical characteristics and outcomes of acute kidney injury in a tertiary care Centre. BMC Nephrol 20 , 282 (2019). https://doi.org/10.1186/s12882-019-1466-z
Download citation
Received : 12 August 2018
Accepted : 17 July 2019
Published : 26 July 2019
DOI : https://doi.org/10.1186/s12882-019-1466-z
Share this article
Anyone you share the following link with will be able to read this content:
Sorry, a shareable link is not currently available for this article.
Provided by the Springer Nature SharedIt content-sharing initiative
- Acute kidney injury
BMC Nephrology
ISSN: 1471-2369
- Submission enquiries: [email protected]
- General enquiries: [email protected]
- Search Menu
- Supplements
- Author videos
- Advance Articles
- Author Guidelines
- Submission Site
- Open Access Options
- Why publish with CKJ?
- About the ERA
- Journals Career Network
- Editorial Board
- Advertising and Corporate Services
- Self-Archiving Policy
- The ERA Journals
- Journals on Oxford Academic
- Books on Oxford Academic

Article Contents
Introduction, materials and methods, time course of aki, acknowledgements.
- < Previous
Long-term consequences of acute kidney injury: a narrative review

- Article contents
- Figures & tables
- Supplementary Data
Joana Gameiro, Filipe Marques, José António Lopes, Long-term consequences of acute kidney injury: a narrative review, Clinical Kidney Journal , Volume 14, Issue 3, March 2021, Pages 789–804, https://doi.org/10.1093/ckj/sfaa177
- Permissions Icon Permissions
The incidence of acute kidney injury (AKI) has increased in the past decades. AKI complicates up to 15% of hospitalizations and can reach up to 50–60% in critically ill patients. Besides the short-term impact of AKI in patient outcomes, several studies report the association between AKI and adverse long-term outcomes, such as recurrent AKI episodes in 25–30% of cases, hospital re-admissions in up to 40% of patients, an increased risk of cardiovascular events, an increased risk of progression of chronic kidney disease (CKD) after AKI and a significantly increased long-term mortality. Despite the long-term impact of AKI, there are neither established guidelines on the follow-up care of AKI patients, nor treatment strategies to reduce the incidence of sequelae after AKI. Only a minority of patients have been referred to nephrology post-discharge care, despite the evidence of improved outcomes associated with nephrology referral by addressing cardiovascular risk and risk of progression to CKD. Indeed, AKI survivors should have specialized nephrology follow-up to assess kidney function after AKI, perform medication reconciliation, educate patients on nephrotoxic avoidance and implement strategies to prevent CKD progression. The authors provide a comprehensive review of the transition from AKI to CKD, analyse the current evidence on the long-term outcomes of AKI and describe predisposing risk factors, highlight the importance of follow-up care in these patients and describe the current therapeutic strategies which are being investigated on their impact in improving patient outcomes.
Acute kidney injury (AKI) is an acute decrease in kidney function defined by an increase in serum creatinine (SCr) or a decrease in urine output (UO) [ 1 , 2 ]. The incidence of AKI has increased in the past decades, reflecting the increased recognition of this diagnosis, patient ageing and increase in AKI risk factors and co-morbidities [diabetes, hypertension, chronic kidney disease (CKD), cardiovascular disease (CVD), liver disease, lung disease, sepsis and surgery] and exposure to nephrotoxic drugs [ 1 , 3–7 ]. AKI complicates 5.0 to 15.0% of hospitalizations and can reach up to 50–60% in critical care patients [ 1 , 3–7 ]. Despite remaining significantly high, mortality rates have declined in the past decade reflecting improvements in patient care, namely by improvements in dialytic care, availability of less nephrotoxic drugs and a decrease in use of dopamine and diuretics [ 2 , 7–9 ].
The increase in survival of AKI patients, has contributed to a significant increase in long-term outcomes associated with AKI, namely recurrence of AKI episodes, development or progression of CKD, characterized by the presence of kidney disease for >90 days, increasing risk of cardiovascular events, increasing hospital admissions, reduced quality of life and long-term mortality [ 10–15 ] ( Figure 1 ).

Long-term consequences after AKI. An AKI event can lead to renal recovery or development of Acute kidney disease (AKD). In the long-term, AKI and AKD are associated with development or progression of CKD, recurrent AKI episodes, risk of cardiovascular events and higher risk of long-term mortality. Even AKI recovery has been associated with increased long-term outcomes.
Despite the long-term impact of AKI, there are neither established guidelines on the follow-up care of AKI patients, nor treatment strategies to reduce the incidence of sequelae after AKI. Indeed, only a minority of patients received comprehensive nephrology post-discharge care, despite the evidence of improved outcomes associated with nephrology referral by addressing cardiovascular risk and risk of progression to CKD [ 16 , 17 ].
The authors provide a comprehensive review of current evidence of the long-term outcomes of AKI and predisposing risk factors, and highlight the importance of follow-up care in improving patient outcomes.
We conducted two literature searches in May 2020, using MEDLINE through the PubMed search engine with the MeSH terms: (i) AKI, prognosis and (ii) AKI, long term, outcomes.
We included articles published in English after the year 2010 up to 20 May 2020, of adult patients with AKI measuring mortality, dialysis dependence, CKD and cardiovascular events.
Diagnosis and pathophysiology of AKI
The Kidney Disease Improving Global Outcomes (KDIGO) guidelines define AKI as an increase in SCr of at least 0.3 mg/dL within 48 h, or an increase in SCr >1.5 times baseline within the prior 7 days, or a decrease in UO to <0.5 mL/kg/h for 6 h [ 18 ]. This classification further stratifies AKI according to three stages of disease severity which correlate with worse degrees of prognosis [ 18 ] ( Figure 2 ).
![dissertation in acute kidney injury AKI, AKI recovery and AKD definitions (adapted from KDIGO, Acute Disease Quality Initiative (ADQI) and from Duff and Murray [56]).](https://oup.silverchair-cdn.com/oup/backfile/Content_public/Journal/ckj/14/3/10.1093_ckj_sfaa177/1/m_sfaa177f2.jpeg?Expires=1716845375&Signature=PFfQiy9ojaoTEizwBIjv3N8i4Gy~OQGuwpYSYZfewWuewgb5jQXs5F~Ff7fcedXAeCaoW1NORIzqXoxWnXSvtG-M7eq6UxBEPMsz8Z57K5aWqq53lnXjEtha7R-N9LRSGqangWMnEKIHDq8HH5yIJz8~b6EPg5Yw0AJxiV-r-aHs0paMtLp-JwPROm6V8k5NQN32rQaYjCTklMkoM7J7Zg9HQ1Y0favZRywli6OY4M7kNtEvQou3Bukf~O3XtaGc~oWqc5RRWWdeOwPjcC~3oWn91SiPZ-tUR0Q7TvkYgu5kg~l2dlv3PUG7i27NAwb6k2Ja7XFG2BiEEaOIZoMkYg__&Key-Pair-Id=APKAIE5G5CRDK6RD3PGA)
AKI, AKI recovery and AKD definitions (adapted from KDIGO, Acute Disease Quality Initiative (ADQI) and from Duff and Murray [ 56 ]).
The kidney is highly susceptible to systemic imbalances and the causes of AKI range from pre-renal AKI, acute tubular necrosis, acute interstitial nephritis, acute glomerular diseases and acute obstructive nephropathy [ 19 ]. The most common causes of AKI in hospitalized patients are septic shock, post major surgery, cardiogenic shock and hypovolaemia [ 20 ].
The pathophysiology of AKI is a complex interplay of pathways triggered by an inciting event which leads to an imbalance of oxygen supply and demand [ 21–25 ]. These processes include haemodynamic instability, microcirculatory dysfunction, tubular cell injury, tubular obstruction, renal congestion, microvascular thrombi, endothelial dysfunction and inflammation [ 21–26 ].
Despite affecting all the segments of the nephron, proximal tubular cells are the most frequently injured, causing loss of polarity, apoptosis or necrosis, which depend on severity of injury [ 26 , 27 ]. Damage to proximal tubular cells consequently results in afferent arteriolar vasoconstriction mediated by tubuloglomerular feedback, luminal obstruction and back leak of filtrate across injured proximal tubular cells, which leads to an abrupt decrease in glomerular filtration [ 21 ]. Additionally, kidney injury prompts the production and release of inflammatory and vasoactive mediators causing leucocyte adherence and interstitial infiltration and lead to microcirculatory flow disruption.
Indeed, inflammation is considered to play a critical role in the pathophysiology of AKI, namely in local kidney injury, in the multi-organ failure associated with AKI, in kidney recovery and also in the progression to CKD which can result if these immune mechanisms persist [ 26–30 ].
It is postulated that transient AKI reflects a temporary reduction in renal function without structural damage, whereas persistent AKI reflects structural tubular damage [ 31–34 ]. The duration of AKI could also reflect the potential to recover the injured kidney [ 31 , 33 , 35 ]. Additionally, the degree of severity of the patient’s illness can also impact on AKI duration [ 31 , 33 ]. Thus, non-recovery from AKI seems to be predisposed by several mechanisms resulting in the exposure of a reduced nephron mass to higher injury in association with impaired repair mechanisms [ 36 ].
Several studies have reported an association of rapid recovery of kidney function and better short-term survival [ 37–42 ]. Coca et al. conducted the largest prospective study to date demonstrating the prognostic impact of AKI duration [ 31 ]. In their cohort of postoperative AKI diabetic patients, the mortality rate increased by AKI duration when stratified by AKIN stage [ 31 ]. They demonstrated that the mortality rate for patients with AKIN Stage 1 with a duration of >7 days was >2-fold higher than for patients with AKIN Stage 3 for <2 days [ 31 ] ( Figure 3 ).
![dissertation in acute kidney injury Impact of AKI severity and duration on mortality (adapted from Coca et al. [31]).](https://oup.silverchair-cdn.com/oup/backfile/Content_public/Journal/ckj/14/3/10.1093_ckj_sfaa177/1/m_sfaa177f3.jpeg?Expires=1716845375&Signature=vUhZy5t34JMDZOFxUBB1ahcvGK4xRBfz6O-p62Allq6xWmkTGalm7ndQnJbzxKhaetUQSF4jPOVEpWHTcf7lqqIhs198i2rmKVx3dz3GZbhLRO9akTKIl~AamxIiLzN4btEzUYuhtWwmUr6RGTKVTKfoFunoYWo8gg2qxnI178Sf4otq1brhtvmBmokblPFwIchPwP8saSqsT8Qrzsiz4OGSeUvq-4kTcmktTU8AQK4NRpnx23d6689xLvKEiHxHzuqsf2XAyONixpQ5t6kGRa5d3NYQATWUby4puBVGfsACejgkiwVt5InwCA6vatwEYM5hob-WKkBFWeJTAQwmUg__&Key-Pair-Id=APKAIE5G5CRDK6RD3PGA)
Impact of AKI severity and duration on mortality (adapted from Coca et al. [ 31 ]).
The previous classifications systems of AKI do not take into account the duration of AKI, which is a significant aspect of AKI severity [ 37 ]. The Acute Disease Quality Initiative (ADQI) has recently defined transient AKI when baseline kidney function is recovered within 48 h, while persistent AKI is defined as kidney dysfunction which persists for longer than 48 h ( Figure 2 ) [ 37 ].
AKI recovery
Renal recovery after AKI is a complex process which is not entirely understood though appears to be dependent on AKI severity, aetiology, duration and baseline renal function [ 43 ]. The timeline and trajectory of renal recovery will depend on reversal of the pathophysiological processes involved [ 44 ]. Renal repair may be the result of regeneration of cells and reestablishment of polarity [ 27 ].
Studies report that the incidence of renal recovery can range from 0% to 90% considering all stages of AKI severity, but from 0% to 40% in cases of dialysis requiring AKI [ 36 ]. The heterogeneity in populations studied and in AKI and reversibility definitions used has contributed to the difficulty in defining and quantifying renal recovery after AKI [ 36 ]. The most often used criteria to assess renal recovery is a decrease in SCr, which is associated with certain limitations, such as loss of muscle mass, changes in volume of distribution, changes in renal reserve and hyperfiltration [ 45 ]. This is supported by studies demonstrating the increased risk in CKD after AKI even when there is an apparent return of SCr to baseline [ 46 , 47 ].
The presence of proteinuria has also been recognized as a marker of underlying kidney injury and has been associated with worse outcomes after AKI episodes [ 48 ]. Novel biomarkers for AKI are being researched to more accurately assess renal recovery, namely plasma neutrophil gelatinase-associated lipocalin (NGAL), tissue inhibitor metalloproteinase-2 and insulin-like growth factor binding protein-7 ([TIMP-2] × [IGFBP7]), urine concentrations of interleukin (IL)-18 and liver-type fatty acid-binding protein (L-FABP) [ 49–52 ].
The ideal definition of kidney recovery after AKI should accurately assess baseline kidney function to differentiate non-recovery from pre-existing CKD, current residual kidney function and reserve and be able to provide prognosis.
The trajectory of renal recovery can take many forms and is associated with long-term prognosis [ 36 ]. Recovery can be assessed as a relative or absolute change, or as a fixed threshold, and according to how persistent an episode of AKI is or to how sustained the recovery is [ 53 ]. Kellum et al. identified five phenotypes of renal recovery after AKI, namely early sustained AKI reversibility, late sustained AKI reversibility, relapse AKI and recovery, relapse AKI without recovery and never recovered AKI, which had distinct characteristics and correlated differently with prognosis [ 54 ]. In this study, non-recovery of renal function at hospital discharge was frequent and associated with an increase risk in mortality ( Figure 4 ). Interestingly, late recovery of renal function was associated with better outcomes than non-recovery, and worse than early reversal of renal function [ 54 ]. Heung et al. also identified different patterns of renal recovery after AKI and reported an increasing risk of CKD according to AKI severity, duration of injury and time to recovery and that this risk was significant even in mildest forms of AKI with fast recovery [ 47 ]. Therefore, outcomes are not only associated with the degree of renal recovery but also with time to recovery.
![dissertation in acute kidney injury Time course of AKI (adapted from Kellum et al. [54]). Patients who develop AKI may experience (i) early sustained reversal of AKI (reversal before 7 days and sustained till hospital discharge), (ii) late reversal (reversal after 7 days and sustained till hospital discharge), (iii) relapsing AKI with complete recovery at hospital discharge, (iv) relapsing AKI without complete recovery at hospital discharge and (v) non-reversal of AKI.](https://oup.silverchair-cdn.com/oup/backfile/Content_public/Journal/ckj/14/3/10.1093_ckj_sfaa177/1/m_sfaa177f4.jpeg?Expires=1716845375&Signature=lMof50qn6uSoa3MXXjZFQRLLqPeHxOfkE8IWnb0Nw3fMkFHekyKM7BerwU0zVWodcRaq1iw0qddE~MIZy1kvZ9~oQobgp~QcVb9hHA84Kb7~144~PtOFcCFSOW-5RK2620vjzx7~HKP2KDdRLDk~AldjvaNXUhmP9UHSDFFc~FDNg98XEx~eATjC-Xw820rjhyzl87IDrFgTnWq9Pe5IDj5TyHxyznwj35dhdrlX4LeE1K-bv2H65-DNy~R~dtQ0FqhaKOsfqtKFVIq61yYq5KzQBeaTt0IsGBS-Gbl4wXwUvA-DL6~A80r3oy-J48XB9Vg5tmN6e~Bnqo9t650KbA__&Key-Pair-Id=APKAIE5G5CRDK6RD3PGA)
Time course of AKI (adapted from Kellum et al . [ 54 ]). Patients who develop AKI may experience (i) early sustained reversal of AKI (reversal before 7 days and sustained till hospital discharge), (ii) late reversal (reversal after 7 days and sustained till hospital discharge), (iii) relapsing AKI with complete recovery at hospital discharge, (iv) relapsing AKI without complete recovery at hospital discharge and (v) non-reversal of AKI.
In 2017, the ADQI defined AKI reversal as the absence of AKI by both SCr and UO criteria (according to the KDIGO classification) within 7 days after the AKI inciting event. The term acute kidney disease (AKD) was thus proposed to define a condition in which AKI Stage 1 or greater (KDIGO) is present for a duration between 7 and 90 days after AKI onset [ 55 ]. Thus, AKD follows on from AKI in patients who do not fully recover within 7 days and is stratified in four different stages of severity ( Table 1 ).
AKD Classification according to the ADQI
A recent perspective by Duff and Murray reflects on the lack of standardized definition of renal recovery in the literature and proposes that AKI recovery could be defined as a decrease of SCr of at least 33% from the reference SCr (value at admission or value which led to AKI diagnosis) within 7 days [ 56 ]. They also categorize AKI recovery into three stages inversely correlated with the KDIGO SCr criteria [ 56 ]. This approach would increase the recognition of AKI recovery and allow to assess its impact on long-term outcomes.
Important factors associated with non-recovery post-AKI include older age, presence of co-morbidities such as CKD, hypertension, diabetes mellitus and CVD, higher severity of the acute illness reflected by higher Acute Physiologic Assessment and Chronic Health Evaluation (APACHE) or Simplified Acute Physiology Score (SAPS) scores, haemodynamic instability, medical admission, higher severity of AKI, requirement of renal replacement therapy (RRT) and potentially the intermittent modality of RRT, though this remains controversial [ 36 , 54 , 57–60 ] ( Figure 5 ). Hence, non-recovery after AKI results from the exposure of a relatively decreased nephron mass or with impaired repair mechanisms to greater injury.

Risk factors associated with AKI and outcomes post-AKI.
Pathophysiology of AKI to CKD transition
Under certain circumstances, the pro-fibrotic and pro-inflammatory pathways can result in maladaptive repair and transition to CKD after AKI [ 44 ]. Older age, lower baseline kidney function, longer duration of AKI and higher severity of AKI contribute to maladaptive repair [ 44 ]. Thus, severe and repeated injury superimposed on reduced renal reserve in an inflammatory environment can result in a maladaptive repair, characterized by a permanent reduction in kidney function associated with significant structural changes resulting from a complex interaction of injured renal tubular cells, endothelial cells, renin–angiotensin–aldosterone system (RAAS), immune system and interstitial fibroblasts [ 61 , 62 ].
Persistent inflammation and RAAS activation are crucial mechanisms in AKI to CKD transition [ 62 , 63 ]. Indeed, studies have demonstrated an association between RAAS inhibition and lower risk of CKD progression, though no randomized controlled trials have been performed with this goal [ 64 ]. The mechanisms of CKD progression after AKI include nephron loss and consequent hypertrophy of the remaining nephrons which leads to further tubulointerstitial fibrosis and further nephron loss; interstitial immune cell infiltration which leads to interstitial fibrosis; peritubular capillary loss resulting in renal hypoxia accelerating inflammation and fibrosis; injured renal tubular cells can adopt a profibrotic phenotype after cell cycle arrest, affecting other epithelial cells, pericytes and the immune system; and maladaptive repair which promotes the activation and growth of fibroblasts which contribute to the deposition of extracellular matrix and resulting fibrosis [ 44 , 61 , 65 , 66 ].
The term AKD reflects the continuing pathological processes and adverse events developing after AKI, highlights the importance of renal recovery and reinforces the hypothesis that AKI and CKD are a continuum rather than separate entities [ 55 ].
Biomarkers of AKI to CKD transition
Biomarkers of these pathological processes, such as epithelial tubular injury, cell cycle arrest, systemic inflammatory pathways and glomerular filtration, would help identify patients at risk for CKD development [ 44 ]. Elevated levels of urinary and serum kidney injury molecule 1 (KIM-1), NGAL, L-FABP, IL-18 and TIMP-2 × IGFBP7 indicate persistent tubular injury, which could be used to identify risk of later CKD development [ 44 , 48 , 67 , 68 ]. Indeed, in 692 patients, TIMP-2 × IGFBP7 > 2.0 at admission was associated with mortality or dialysis at 9 months (hazard ratio (HR) = 2.16, 95% CI 1.32–3.53) [ 69 ]. Also, urinary angiotensinogen has been associated with higher AKI severity and mortality and considering the role of RAAS activation in progression to CKD is considered a promising prognostic marker [ 44 , 70 ]. Further investigations on anti-fibrotic interventions are required to develop strategies to preserve renal function and prevent the transition from AKI to CKD.
Long-term renal outcomes
Recent research described the association between AKI and long-term renal outcomes such as recurrent AKI episodes, development and progression to CKD. In recent systematic reviews, the pooled rate of CKD after AKI ranged from 10.17 to 25.8 cases per 100 person-years [ 71 , 72 ].
Recurrent AKI
AKI has been associated with increased rates of hospital readmissions. The rate of readmission increases with AKI severity and can reach up to 40% in AKI requiring dialysis within the first month [ 73–76 ].
Several studies have focused on the incidence of recurrent episodes of AKI ( Table 2 ). Liu et al . conducted one of the largest studies on AKI, including 38 659 patients with hospital-acquired AKI and identified a second AKI episode in 28.6% of patients [ 77 ]. Furthermore, recurrent AKI was associated with an increased mortality risk (HR = 1.66; 95% CI 1.57–1.77). Likewise, Siew et al. reported recurrent AKI in 25% cases in a population of 11 683 patients [ 78 ]. Furthermore, the 1-year mortality was higher in patients with recurrent AKI (35% versus 18%, P < 0.001) [ 78 ].
Summary of studies assessing AKI and AKI recurrence
In a large population of hospitalized and community patients, Holmes et al. identified a recurrence rate of AKI of 30% and an association of recurrent AKI and higher 30-day mortality rate (OR = 1.33, 95% CI 1.28–1.38) [ 79 ]. Interestingly, with each episode of AKI, the probability of another episode also increased [ 79 ].
This has also been proven in intensive care unit (ICU) patients. Harris et al. demonstrated that AKI was associated with recurrent AKI episodes (OR = 1.9, 95% CI 1.0–3.6) in a cohort of 624 critically ill surgical patients. Moreover, recurrent AKI was associated with increased 1-year mortality (OR = 2.6, 95% CI 1.4–5.1) [ 80 ]. An increasing mortality risk associated with recurrent AKI was also demonstrated in a study of 400 critically ill septic patients in which the incidence of AKI was 20% (HR = 1.97, 95% CI 1.36–2.84) [ 81 ].
The impact of recurrent episodes of AKI on prognosis is also reflected in a cohort of 3679 diabetic patients with preserved renal function analysed by Thakar et al. In this study, 30% of patients experienced >2 AKI episodes which had a cumulative risk effect for developing CKD [ 82 ].
Furthermore, in a cohort of 359 patients in which recurrent AKI was observed in 34% of cases, recurrent AKI was associated with increased development of CKD (HR = 2.2, 95% CI 1.09–4.3), increased risk of cardiovascular events and increased mortality risk (HR = 4.5, 95% CI 2.7–7.5) [ 83 ].
AKI recurrence has a strong impact on renal, cardiovascular and patient outcomes. So, to prevent recurrent AKI, it is important to identify at-risk patients. Older age [ 77 , 79 , 81 ], black race and Hispanic ethnicity, [ 77 ] diabetes, [ 77 , 83 ] CVD, [ 77 , 78 , 83 ] decreased baseline kidney function, [ 77 , 79 , 81 , 83 ] liver disease, [ 77 , 78 ] cancer, [ 78 ] higher illness severity, [ 77 , 81 ] proteinuria, [ 77 ] anaemia [ 77 ] and longer AKI duration [ 78 ] have been associated with incomplete renal recovery after AKI [ 79 ]. Impaired renal reserve and the lingering effects of acute illness or its therapies might contribute to AKI recurrence and outcomes in these patients. Strategies to reduce the incidence of recurrent AKI might improve the long-term outcomes.
CKD development and/or progression
Several studies have demonstrated the association of CKD development following AKI, which have been summarized in previous systematic reviews [ 71 , 72 ]. It is, therefore, crucial to identify risk factors associated with CKD development ( Table 3 ).
Summary of studies assessing AKI and long-term outcomes
Coca et al. reported that AKI increased the risk of CKD by 8-fold (HR = 8.8, 95% CI 3.1–25.5) and the risk of end-stage kidney disease by 3-fold (HR = 3.1, 95% CI 1.9–5.0). Moreover, the risk was higher according to AKI severity (mild AKI HR = 2.3, 95% CI 1.7–3.3; severe AKI HR = 8.0, 95% CI 1.3–48.6) [ 72 ]. Similarly, See et al. demonstrated that the risk of CKD was 3-fold higher in AKI patients (HR = 2.67, 95% CI 1.99–3.58) [ 71 ].
The increased risk of development and/or progression of CKD as a long-term outcome associated with AKI have been demonstrated in multiple settings [ 47 , 90 ]. Chawla et al. demonstrated an increased risk of adverse kidney events [long-term dialysis, 25% decrease in estimated glomerular filtration rate (eGFR) and death] in AKI patients in a cohort of 36 980 Veterans (HR = 2.07, 95% CI 1.99–2.16) [ 14 ]. A Swedish cohort of 97 782 patients critical care patients reported an increased rate of CKD [6.0% versus 0.44%, adjusted incidence rate ratio (IRR) 7.6] and end stage renal disease (ESRD) (3.9% versus 0.3%, adjusted IRR = 22.5) [ 93 ]. In 3245 cardiac surgery patients, the prevalence of CKD was significantly higher in AKI patients (6.8% versus 0.2%, P < 0.001; RR = 1.92, 95% CI 1.37–2.69) in the 2 years after surgery, even with complete recovery of renal function at discharge [ 94 ]. This was also reported in major abdominal surgery in a previous study of 390 patient in whom AKI was a risk factor for need for long-term dialysis and/or a 25% decrease in eGFR after hospital discharge (adjusted HR = 1.6, P = 0.046) [ 96 ].
The risk of CKD is also higher in relation to the severity of AKI as demonstrated in previous studies, and even higher in patients who require dialysis [ 84 ]. Ryden et al. studied 29 330 patients who underwent primary isolated coronary artery bypass grafting and demonstrated that the risk for ESRD increased with AKI severity, and was 2.92 (95% CI 1.87–4.55) for AKIN Stage 1 and 3.81 (95% CI 2.14–6.79) for AKIN Stages 2 and 3 [ 91 ]. In AKI requiring dialysis, the incidence rate of ESRD was 2.63/100 person-years in a study by Wald et al. (HR = 3.23, 95% CI 2.70–3.86) [ 84 ].
The risk of ESRD is higher in patients with previous CKD. Wu et al. reported an incidence rate of long-term dialysis of 17.8/100 person-years in patients with AKI-on-CKD when compared with patients without previous CKD [ 86 ].
Other than AKI severity, considering that even less severe stages of AKI are associated with long-term outcomes, the recovery of renal function after an AKI episode and its risk of developing CKD have also been studied.
Patients who recover renal function less often progress to CKD. Pannu et al. analysed long-term outcomes among 3231 survivors of hospitalization over a 6-year period, and reported an 2.1% incidence of ESRD and 9.8% of CKD in the AKI group, and demonstrated that patients who did not recover renal function after AKI had a higher risk of CKD and ESRD (HR = 4.13, 95% CI 3.38–5.04) [ 88 ]. On the contrary, in a retrospective cohort of 221 087 hospitalized patients by Heung et al . 31.8% of AKI patients progressed to CKD as compared to only 15.5% of non-AKI patients, on a 1-year follow-up (P < 0.001). In this study, even after recovery of AKI within 48 h, there was a relative risk of CKD progression which also increased associated with AKI severity [ 47 ]. Therefore, the duration of AKI might also impact on outcomes.
Indeed, a different threshold of AKI duration has been reported in the prospective study by Bhatraju et al. of 1538 participants in which non-recovery of renal function within the first 72 h after AKI was associated with a 51% greater risk of CKD development or dialysis requirement or death (95% CI 22–88%, P < 0.001) [ 105 ]. However, the risk of CKD development was still higher than non-AKI patients.
Accordingly, the presence of AKD has also been associated with CKD development. Among 225 patients undergoing PCI after myocardial infarction, 58.5% of AKD patients developed new or progressed CKD [ 100 ]. In 256 septic-AKI patients, AKD was also independently associated with adverse renal outcomes (HR = 2.87, 95% CI 2.0–4.1, P < 0.001) [ 107 ].
The majority of studies assessed the link between AKI and CKD based on eGFR decline, still more recent studies have identified new-onset albuminuria following AKI as a marker of CKD progression.
Proteinuria is a well-established and risk factor for CKD progression and cardiovascular events [ 108–110 ]. Interestingly, in a retrospective cohort of 657 840 patients, the risk of developing or worsening proteinuria was higher in AKI patients (OR = 1.39, 95% CI 1.33–1.46) and increased according to AKI severity [ 90 ]. Also, proteinuria and albuminuria have been more prevalent in AKI patients and associated with CKD progression [ 90 ]. Parr et al. also demonstrated that AKI patients had increased risk of developing proteinuria (OR range 1.20–1.39), which also increased according to AKI severity [ 111 ].
Factors associated with higher risk of CKD following AKI were previous increased baseline SCr, male gender, African American race, older age, diabetes, previous CVD, hypoalbuminaemia, lower haematocrit, AKI severity, duration and recovery pattern of AKI, recurrent AKI episodes ( Figure 5 ) [ 14 , 59 , 80 , 84 , 87 , 93 , 96 , 103 , 112 , 113 ].
Development of CKD after AKI is also a risk factor for the development of cardiovascular events and for long-term mortality. Cabrales et al. demonstrated that on a 4-year follow-up after an AKI episode, patients with hypertension (OR = 1.62, 95% CI 1.2–2.6) had a higher risk of developing CKD, patients with previous CKD had a higher risk of CKD progression. Furthermore, CKD patients had an increased risk of cardiovascular events (62.7 versus 21.7%, P < 0.05), and that mortality risk was increased by 4-fold (OR = 4.3, 95% CI 1.13–4.90) [ 101 ].
Furthermore, CKD after AKI has also been associated with increased mortality risk. For instance, in 634 AKI patients, Lai et al. found that the long-term mortality risk increased as kidney function declined during follow-up [ 114 ]. In 425 critically ill AKI patients, development of CKD was an independent predictor of mortality (OR = 4.3, 95% CI 2.9–6.2) [ 87 ].
Long-term cardiovascular outcomes
AKI is associated with increased long-term cardiovascular morbidity [ 115 ]. Odutayo et al . performed a systematic review and reported that AKI was associated with an 86% increased risk of cardiovascular mortality and a 38% increased risk of major cardiovascular events, namely development of chronic heart failure, acute myocardial infarction and stroke [ 116 ].
The relationship between CKD and CVD is well documented [ 115 ]. The term cardiorenal syndrome has been proposed to describe the complex bidirectional interactions between heart and kidneys [ 115 , 117 ]. AKI may lead to direct and indirect effect on cardiac function and structure and thus increase the risk of cardiovascular events. The pathophysiological processes include activation of the sympathetic nervous system, activation of the RAAS, endothelial dysfunction, inflammation, cardiac fibrosis, volume expansion, hypertension, electrolyte disturbances, acidaemia and anaemia [ 115 , 118 ].
AKI has been associated with development with blood pressure elevation. In a retrospective study of 43 611 hospitalized patients, AKI was associated with a 22% increased risk of hypertension within a 2-year follow-up, which greater according to AKI severity [ 119 ]. Indeed, in experimental studies, it has been reported that sodium-sensitive hypertension develops after an ischaemic renal event, due to impaired renal sodium excretion [ 120 ].
The association between AKI and CVD has been demonstrated in several studies ( Table 3 ). In a retrospective study of US Veterans, Chawla et al . reported an increased risk of cardiovascular outcomes, namely myocardial infarction, stroke or heart failure (HR = 1.24, 95% CI 1.18–1.30), in AKI patients [ 14 ]. In a cohort of 4742 cardiac surgery patients the risk of myocardial infarction, heart failure and stroke was significantly increased in AKI patients on a 5-year follow-up (HR = 1.37, 95% CI 1.05–1.80). Additionally, AKI was associated with an increase in both short- and long-term mortality [ 95 ]. Wu et al. demonstrated an incidence rate of coronary events of 19.8/1000 person-years (HR = 1.67, 95% CI 1.36–2.04) independently of progression to CKD [ 92 ]. Similar results have also been reported in non-cardiovascular surgery patients [ 102 ]. Also, in a post hoc analysis of 9361 patients enrolled in the Systolic Blood Pressure Intervention Trial, AKI patients had a higher risk of cardiovascular events (HR = 1.52, 95% CI 1.05–2.20).
The risk of cardiovascular events increases according to AKI severity [ 98 , 103 ]. Lee et al . reported that AKI patients who required dialysis more frequently developed cardiovascular events (HR = 1.97, 95% CI 1.75–2.23) and that this risk was high even patients who recovered renal function (HR = 1.73, 95% CI 1.54–1.95) [ 103 ]. Furthermore, even small transient and changes in renal function have been associated with increased risk of cardiovascular events [ 85 ].
Parikh conducted a study on 968 adults who underwent cardiac surgery which also demonstrated the association of AKI and AKI severity and an increased risk of cardiovascular events [ 98 ]. Interestingly, in this study, there was a significant association between peak postoperative cardiac injury biomarkers and CV outcomes while there was no association between peak postoperative urinary injury biomarkers and CV outcomes [ 98 ]. This further reinforces the systemic and haemodynamic effects of AKI on cardiac dysfunction.
Reported factors associated with higher risk of CVD following AKI were older age, CKD, diabetes and previous cardiovascular events ( Figure 5 ) [ 14 , 15 , 98 , 121 ].
Long-term mortality
The association between AKI and mortality in the long-term is well recognized ( Table 3 ). Interestingly, this association is independent of pre-existing CKD. In a review by Coca et al. , the pooled mortality was 8.9 deaths/100 person-years following AKI [ 122 ]. Similarly, a more recent review by See et al . reported a pooled mortality of 13.2 deaths/100 person-year after AKI [ 71 ].
Chawla et al. demonstrated that the risk of mortality after AKI (HR = 1.85, 95% CI 1.76–1.94, P < 0.001) was almost 2-fold higher than the risk of mortality after myocardial infarction [ 14 ]. This was an important finding which raised awareness to the detrimental impact of AKI on long-term outcomes.
In a longitudinal cohort of 7075 adult patients who were submitted to cardiac surgery, AKI was a predictor of mortality in the 5 years following surgery (30 days to 1 year: HR = 1.834, 95% CI 1.459–2.306; 1–3 years: HR = 1.285, 95% CI 1.023–1.610; and 3–5 years: HR = 1.330, 95% CI 1.123–1.750) [ 99 ].
The increased severity of AKI is also associated with increased mortality risk [ 71 , 122 ]. Fuchs et al. analysed the long-term outcomes of 12 399 survivors from an ICU admission and reported that patients with AKI Stage 3 AKIN had a 61% higher mortality risk on a 2-year follow-up compared with patients without AKI (P = 0.001) [ 89 ]. Less severe changes in renal function were also associated with mortality risk, though this risk was progressively higher with the increasing severity of AKI (AKIN 1: HR = 1.12, AKIN 2: 1.19, AKIN 3: 1.24, compared with no AKI, P < 0.05) [ 89 ]. Soliman et al. also demonstrated that moderate or severe AKI was associated with higher mortality in 2420 patients, 1 year after ICU admission [Risk, Injury, Failure, Loss of kidney function, and End-stage kidney disease (RIFLE) injury adjusted relative risk 1.14, 95% CI 1.01–1.29; P = 0.03; RIFLE failure adjusted relative risk 1.25, 95% CI 1.01, 1.55; P = 0.04] [ 97 ]. Another cohort demonstrated that patients who required RRT had the highest risk of long-term mortality (adjusted HR = 2.65, 95% CI 2.0–3.52) [ 31 ].
The duration of AKI has also been studied as a predictor of long-term mortality. Coca et al. prospectively studied 35 302 diabetic patients who underwent non-cardiac surgery and described that not only the severity but also the duration of AKI decreased long-term survival. Remarkably, patients with longer duration of AKI had higher mortality in every stage of AKIN (log-rank test P < 0.001) [ 31 ]. Similarly, Cheng et al. demonstrated that compared with transient renal dysfunction, patients with persistent AKI following contrast administration had higher mortality after 1 year (RR = 3.768, 95% CI 1.612–8.810; RR = 4.106, 95% CI 1.765–9.551) [ 106 ]. Still, transient changes in renal function are also associated with higher mortality when compared with patients without AKI. Mizota et al . reported that transient AKI was frequent in patients undergoing major abdominal surgery and that compared to patients without AKI, patients with transient (adjusted HR = 2.01, 95% CI 1.34–2.93, P = 0.001) and persistent AKI (adjusted HR = 6.20, 95% CI 3.00–11.43, P < 0.001) had increased mortality rates on a 1-year follow-up [ 104 ].
Importantly, recovery of renal function after AKI appears to be predictor of better long-term survival. In a prospective cohort of 425 critically ill patients the mortality rate patients who recovered renal function after AKI was lower than patients with CKD (46% versus 83%, P < 0.001) [ 87 ]. In a study of 1742 septic patients, it was reported that patients who recovered renal function after AKI had similar survival compared with patients without AKI (HR = 1.01, 95% CI 0.69–1.47, P = 0.96) over a median follow-up of 1.7 years [ 123 ]. Kofman et al. evaluated the incidence and prognosis of AKD after AKI and described that development of AKD was associated with increased long-term mortality in 225 patients with myocardial infarction (35% versus 17%, P < 0.001) [ 100 ].
As previously described, development of CKD and cardiovascular events after AKI is also associated with higher mortality rates.
Whether AKI directly contributes to mortality or serves as a surrogate marker of illness severity in higher-risk patients is still uncertain. The most frequently reported causes of mortality have been CVD, infections and cancer [ 87 , 124 ].
Other than AKI severity, duration and renal recovery; lower baseline kidney function, male gender, older age, presence of comorbidities (diabetes, hypertension, CVD and cancer) and hypoalbuminaemia have also been associated with increased mortality risk following AKI ( Figure 5 ) [ 14 , 99 , 123 , 125–127 ].
Follow-up care after AKI
Despite consistent evidence that AKI has a negative impact on long-term outcomes, the reported rates of nephrology follow-up after AKI are low [ 128 , 129 ]. Furthermore, to date, there is no standardized AKI or AKD follow-up care.
The US Renal Data System annual report of 2015 states that only 19% of patients had a nephrology follow-up at 12 months after an AKI hospitalization [ 130 ]. Siew et al . reported nephrology referral in only 4% of patients at 3 months and only 9% at 1 year, despite the fact that the mortality rate during this period was 22% [ 16 ]. Patients who were not referred to nephrology consult were slightly older, but had better kidney function and were less likely to have diabetes or heart failure [ 16 ]. An important limitation of this study is that the reasons for referral or non-referral are not reported, namely decisions to limit care.
The benefit of nephrology referral is uncertain, though, Harel et al . reported that on the 41% of 3877 AKI patients who were referred to nephrology follow-up within 90 days there was a 24% mortality reduction in 2 years of follow-up, in a retrospective analysis [ 131 ]. Interestingly, to reduce survival bias, patients were matched using a propensity score for co-morbidities and required a minimum survival period following hospital discharge of 90 days, to exclude patients with high disease burden in whom nephrology follow-up would be of less benefit.
The exact factors that contribute to this survival benefit are not completely clarified; however, recognition and treatment of cardiovascular risk factors and CKD complications are most likely implicated. Additionally, the nephrologist can be useful in patients with decision to limit care, as part of the palliative care team.
During the AKI episode the main goal should be the recovery to baseline kidney function in the shortest period of time in order to reduce the duration and disease severity [ 132–134 ]. After discharge it is crucial to preserve renal function and prevent further deterioration, by controlling hypertension, proteinuria, diabetes mellitus and CVD [ 135 ].
The current recommendations by the KDIGO and the ADQI state that patients should be followed by a nephrologist at least 3 months after an AKI episode in order to estimate kidney recovery and/or progression to CKD, or progressive CKD [ 18 , 133 ]. The follow-up assessment should include kidney function and proteinuria, medication reconciliation, patient education to nephrotoxic avoidance and strategies to prevent CKD progression [ 133 , 136 ].
Prescribing RAAS inhibitors after an AKI episode is promising as it might decrease the loss of kidney function, decrease CVD events and decrease mortality [ 64 , 137 , 138 ]. Chou et al. prospectively analysed 587 patients who recovered kidney function after cardiac-surgery associated AKI, and demonstrated that prescription of RAAS inhibitors after kidney function recovery was independently associated with lower risk of CKD development (26.6% versus 42.2%, HR = 0.46, P < 0.001) [ 64 ]. A retrospective cohort of 46 253 AKI survivours who were prescribed RAAS inhibitors within 6 months of the AKI episode, demonstrated a decreased mortality risk after 2 years (HR = 0.85, 95% CI 0.81–0.89). However, there was an increased risk of hospitalization for a renal cause [ 137 ]. Still, the risk of AKI recurrence associated with RAAS inhibitors has not been demonstrated in more recent studies [ 139 ].
Another promising therapeutic intervention is the use of statins. On a retrospective cohort of 19 707 patients with CKD after AKI, patients who were prescribed statins after AKI had lower rate of hospitalizations and mortality risk over a 2-year follow-up [ 75 ]. Still, timing of statin prescription was not assessed neither were some important covariates, namely blood pressure.
The use of RAAS inhibitors and statins is promising to reduce the cardiovascular risk after AKI, which has a significant prevalence in these patients. However, interventional and further observational studies are needed to establish the concrete benefit of RAAS inhibitors and/or statins after AKI and to define the adequate timing and dose to start these medications after AKI.
Several medications which can decrease renal function or are associated with adverse events due to drug accumulation in CKD patients must also be adjusted in high-risk patients. Non-steroidal anti-inflammatory drugs (NSAIDs) affect renal function due to prostaglandin inhibition and are associated with an increased risk of AKI and CKD [ 140 ]. AKI is predominantly haemodynamically mediated but can also be immune-mediated, and is higher in elderly patients and with concomitant use of RAAS inhibitors or diuretics [ 140 ]. The development of CKD appears to be associated with higher doses of NSAIDs [ 141 ]. Although the risk of adverse effects of NSAIDs following AKI has not been specifically evaluated it is important to recognize the increased risk of this population and to carefully prescribe these medications [ 141 ].
Given that the risk of hypoglycaemia after discharge following AKI has been reportedly higher in patients medicated with insulin and sulfonylurea, it is necessary to intervene and lower the risk of these episodes in patients medicated with glucose-lowering agents with renal excretion [ 142 ]. The use of sodium–glucose cotransporter 2 (SGLT2) inhibitors to improve glycaemic control after AKI is promising as these have been associated with decreasing the risk of cardiovascular events and CKD progression [ 143 ]. Still, further randomized trials are necessary to assess the safety of these drugs in patients with recent AKI or CKD patients.
Assessing which patients are at higher risk for CKD development, cardiovascular events and long-term mortality after AKI are therefore crucial ( Figure 5 ). Prediction models applied at discharge could estimate the risk of adverse outcomes and target patients at risk with specialized interventions [ 144 ]. The use of biomarkers to estimate risk is also promising as higher levels of NGAL, IL-18, KIM-1, albuminuria, TIMP-2 × IGFBP7 have been associated with increased long-term adverse outcomes after AKI [ 69 , 145 ]. To date, only NGAL and TIMP-2 × IGFBP7 have been approved, though their widespread use in clinical practice is still distant. These biomarkers might provide prognostic information during the AKI period, although no studies have analysed patient follow-up assessed with these biomarkers.
Currently, two randomized clinical trials are comparing standardized care to nephrology follow-up [ 146 , 147 ]. The FUSION trial will compare patients with a referral to family physician after hospitalization and later local nephrology follow-up if considered appropriate by the physician versus patients who will be referred to an AKI follow-up clinic within 30 days after discharged and routine laboratory every 3 months, and assess patient outcomes on a 5-year follow-up [ 146 ]. This trial The AFTER-AKI trial will compare patients discharged with usual discharge protocols and patients who will receive specific follow-up protocols based on their risk of CKD, and assess outcomes after 1-year follow-up [ 147 ]. These are highly anticipated to clarify the importance of specialized care after AKI on improving patient outcomes.
Further research is essential to stratify high-risk patients, define timing for nephrology follow-up and to develop strategies to improve patient outcomes.
It is crucial to stop regarding AKI as a short-term reversible condition and to raise awareness on the long-term complications, such as progression to CKD, increased cardiovascular events and mortality. Whether AKI is a marker of patients with more co-morbidities and increased risk for poor prognosis or directly contributes to adverse outcomes is still unclear.
It is evident that AKI survivors should have specialized long-term follow-up. Nephrology follow-up is crucial to assess kidney function after AKI, perform medication reconciliation, educate patients on nephrotoxic avoidance and implement strategies to prevent CKD progression.
Still, further investigation is required on assessment of biomarkers of renal recovery and worse renal and vital prognosis, and on assessment of safety and dosing of specific therapeutic strategies (RAAS inhibitors, statins and SGLT2 inhibitors) to prevent CKD and CVD after AKI, and in defining optimal follow-up timing ( Table 4 ).
List of research needs on the period after AKI
The authors would like to acknowledge Joana Paraíba for the significant contributions in designing the figures.
No funding was received for this study.
AUTHORS’ CONTRIBUTIONS
J.G. made substantial contributions to the study concept and was involved in drafting the manuscript, F.M. made substantial contributions to the study design and J.A.L. was involved in revising it critically for intellectual content.
CONFLICT OF INTEREST STATEMENT
The authors declare there is no conflict of interest.
Susantitaphong P , Cruz DN , Cerda J et al. World incidence of AKI: a meta-analysis . Clin J Am Soc Nephrol 2013 ; 8 : 1482 – 1493
Google Scholar
Hsu RK , McCulloch CE , Dudley RA et al. Temporal changes in incidence of dialysis-requiring AKI . J Am Soc Nephrol 2013 ; 24 : 37 – 42
Lameire N , Van Biesen W , Vanholder R. The changing epidemiology of acute renal failure . Nat Rev Nephrol 2006 ; 2 : 364 – 377
Rodrigues FB , Bruetto RG , Torres US et al. Incidence and mortality of acute kidney injury after myocardial infarction: a comparison between KDIGO and RIFLE criteria . PLoS One 2013 ; 8 : e69998
Luo X , Jiang L , Du B et al. ; The Beijing Acute Kidney Injury Trial (BAKIT) Workgroup. A comparison of different diagnostic criteria of acute kidney injury in critically ill patients . Crit Care 2014 ; 18 : R144
Fujii T , Uchino S , Takinami M et al. Validation of the kidney disease improving global outcomes criteria for AKI and comparison of three criteria in hospitalized patients . Clin J Am Soc Nephrol 2014 ; 9 : 848 – 854
Bagshaw SM , George C , Bellomo R. Changes in the incidence and outcome for early acute kidney injury in a cohort of Australian intensive care units . Crit Care 2007 ; 11 : R68
Bellomo R. The epidemiology of acute renal failure: 1975 versus 2005 . Curr Opin Crit Care 2006 ; 12 : 557 – 560
Cruz DN , Ronco C. Acute kidney injury in the intensive care unit: current trends in incidence and outcome . Crit Care 2007 ; 11 : 149
Chertow GM , Burdick E , Honour M et al. Acute kidney injury, mortality, length of stay, and costs in hospitalized patients . J Am Soc Nephrol 2005 ; 16 : 3365 – 3370
Kim J , Kim YJ , Ryoo S et al. One-year progression and risk factors for the development of chronic kidney disease in septic shock patients with acute kidney injury: a single-centre retrospective cohort study . J Clin Med 2018 ; 7 : 554
Rubin S , Orieux A , Clouzeau B et al. The incidence of chronic kidney disease three years after non-severe acute kidney injury in critically ill patients: a single-center cohort study . J Clin Med 2019 ; 8 : 2215
Sawhney S , Mitchell M , Marks A et al. Long-term prognosis after acute kidney injury (AKI): what is the role of baseline kidney function and recovery? A systematic review . BMJ Open 2015 ; 5 : e006497 – e006497
Chawla LS , Amdur RL , Shaw AD et al. Association between AKI and long-term renal and cardiovascular outcomes in United States veterans . Clin J Am Soc Nephrol 2014 ; 9 : 448 – 456
Omotoso BA , Abdel-Rahman EM , Xin W et al. Acute kidney injury (AKI) outcome, a predictor of long-term major adverse cardiovascular events (MACE) . Clin Nephrol 2016 ; 85 : 1 – 11
Siew ED , Peterson JF , Eden SK et al. outpatient nephrology referral rates after acute kidney injury . J Am Soc Nephrol 2012 ; 23 : 305 – 312
Kirwan CJ , Blunden MJ , Dobbie H et al. critically ill patients requiring acute renal replacement therapy are at an increased risk of long-term renal dysfunction, but rarely receive specialist nephrology follow-up . Nephron 2015 ; 129 : 164 – 170
Khwaja A. KDIGO clinical practice guidelines for acute kidney injury . Nephron 2012 ; 120 : c179 – c184
Kellum JA , Lameire N , for the KDIGO AKI Guideline Work Group Diagnosis, evaluation, and management of acute kidney injury: a KDIGO summary (Part 1) . Crit Care 2013 ; 17 : 204
Uchino S. Acute renal failure in critically ill patients a multinational, multicenter study . JAMA 2005 ; 294 : 813
Ostermann M , Liu K. Pathophysiology of AKI . Best Pract Res Clin Anaesthesiol 2017 ; 31 : 305 – 314
Case J , Khan S , Khalid R et al. Epidemiology of acute kidney injury in the intensive care unit . Crit Care Res Pract 2013 ; 2013 : 1 – 9
Akcay A , Nguyen Q , Edelstein CL. Mediators of inflammation in acute kidney injury . Mediators Inflamm 2009 ; 2009 : 1 – 12
Basile DP , Anderson MD. Pathophysiology of acute kidney injury. Comprehensive physiology . Hoboken, NJ : John Wiley & Sons, Inc , 2012
Google Preview
Devarajan P. Update on mechanisms of ischemic acute kidney injury . J Am Soc Nephrol 2006 ; 17 : 1503 – 1520
Sharfuddin AA , Molitoris BA. Pathophysiology of ischemic acute kidney injury . Nat Rev Nephrol 2011 ; 7 : 189 – 200
Liu KD , Brakeman PR. Renal repair and recovery . Crit Care Med 2008 ; 36 : S187 – S192
Bonventre JV. Pathophysiology of acute kidney injury: roles of potential inhibitors of inflammation . Contrib Nephrol 2007 ; 156 : 39 – 46
Lee DW , Faubel S , Edelstein CL. Cytokines in acute kidney injury (AKI) . Clin Nephrol 2011 ; 76 : 165 – 173
Jang HR , Rabb H. Immune cells in experimental acute kidney injury . Nat Rev Nephrol 2015 ; 11 : 88 – 101
Coca SG , King JT , Rosenthal RA et al. The duration of postoperative acute kidney injury is an additional parameter predicting long-term survival in diabetic veterans . Kidney Int 2010 ; 78 : 926 – 933
Vanmassenhove J , Glorieux G , Hoste E et al. AKI in early sepsis is a continuum from transient AKI without tubular damage over transient AKI with minor tubular damage to intrinsic AKI with severe tubular damage . Int Urol Nephrol 2014 ; 46 : 2003 – 2008
Darmon M , Truche AS , Abdel-Nabey M et al. Early recognition of persistent acute kidney injury . Semin Nephrol 2019 ; 39 : 431 – 441
Ronco C , Kellum JA , Haase M. Subclinical AKI is still AKI . Crit Care 2012 ; 16 : 313
Schmitt R , Coca S , Kanbay M et al. Recovery of kidney function after acute kidney injury in the elderly: a systematic review and meta-analysis . Am J Kidney Dis 2008 ; 52 : 262 – 271
Forni LG , Darmon M , Ostermann M et al. Renal recovery after acute kidney injury . Intensive Care Med 2017 ; 43 : 855 – 866
Chawla LS , Bellomo R , Bihorac A et al. ; on behalf of the Acute Disease Quality Initiative Workgroup 16. Acute kidney disease and renal recovery: consensus report of the Acute Disease Quality Initiative (ADQI) 16 workgroup . Nat Rev Nephrol 2017 ; 13 : 241 – 257
Sood MM , Shafer LA , Ho J et al. Early reversible acute kidney injury is associated with improved survival in septic shock . J Crit Care 2014 ; 29 : 711 – 717
Perinel S , Vincent F , Lautrette A et al. Transient and persistent acute kidney injury and the risk of hospital mortality in critically ill patients . Crit Care Med 2015 ; 43 : e269 – e275
Fidalgo P , Ahmed M , Meyer SR et al. Association between transient acute kidney injury and morbidity and mortality after lung transplantation: a retrospective cohort study . J Crit Care 2014 ; 29 : 1028 – 1034
Gameiro J , Duarte I , Marques F et al. Transient and persistent AKI and outcomes in patients undergoing major abdominal surgery . Nephron 2020 ; 144 : 236 – 244
Coelho S , Fonseca JN , Gameiro J et al. Transient and persistent acute kidney injury in acute liver failure . J Nephrol 2019 ; 32 : 289 – 296
Bagshaw SM. Epidemiology of renal recovery after acute renal failure . Curr Opin Intern Med 2007 ; 6 : 31 – 37
Yang L. How acute kidney injury contributes to renal fibrosis . Adv Exp Med Biol 2019 ; 1165 : 117 – 142
Prowle JR , Kolic I , Purdell-Lewis J et al. Serum creatinine changes associated with critical illness and detection of persistent renal dysfunction after AKI . Clin J Am Soc Nephrol 2014 ; 9 : 1015 – 1023
Bucaloiu ID , Kirchner HL , Norfolk ER et al. Increased risk of death and de novo chronic kidney disease following reversible acute kidney injury . Kidney Int 2012 ; 81 : 477 – 485
Heung M , Steffick DE , Zivin K et al. Acute kidney injury recovery pattern and subsequent risk of CKD: an analysis of Veterans health administration data . Am J Kidney Dis 2016 ; 67 : 742 – 752
Malhotra R , Siew ED. Biomarkers for the early detection and prognosis of acute kidney injury . Clin J Am Soc Nephrol 2017 ; 12 : 149 – 173
Pike F , Murugan R , Keener C et al. Biomarker enhanced risk prediction for adverse outcomes in critically ill patients receiving RRT . Clin J Am Soc Nephrol 2015 ; 10 : 1332 – 1339
Kashani K , Cheungpasitporn W , Ronco C. Biomarkers of acute kidney injury: the pathway from discovery to clinical adoption . Clin Chem Lab Med 2017 ; 55 : 1074 – 1089
Srisawat N , Wen X , Lee M et al. Urinary biomarkers and renal recovery in critically ill patients with renal support . Clin J Am Soc Nephrol 2011 ; 6 : 1815 – 1823
Dewitte A , Joannès-Boyau O , Sidobre C et al. Kinetic eGFR and novel AKI biomarkers to predict renal recovery . Clin J Am Soc Nephrol 2015 ; 10 : 1900 – 1910
Kellum JA. How can we define recovery after acute kidney injury? considerations from epidemiology and clinical trial design . Nephron Clin Pract 2014 ; 127 : 81 – 88
Kellum JA , Sileanu FE , Bihorac A et al. Recovery after acute kidney injury . Am J Respir Crit Care Med 2017 ; 195 : 784 – 791
Bagshaw SM , George C , Bellomo R et al. Early acute kidney injury and sepsis: a multicentre evaluation . Crit Care 2008 ; 12 : R47
Duff S , Murray PT. Defining early recovery of acute kidney injury . Clin J Am Soc Nephrol. 2020 ; Apr 1;CJN.13381019.
Ali T , Khan I , Simpson W et al. Incidence and outcomes in acute kidney injury: a comprehensive population-based study . J Am Soc Nephrol 2007 ; 18 : 1292 – 1298
Liang KV , Sileanu FE , Clermont G et al. Modality of RRT and recovery of kidney function after AKI in patients surviving to hospital discharge . Clin J Am Soc Nephrol 2016 ; 11 : 30 – 38
Macedo E , Zanetta DMT , Abdulkader RCRM. Long-term follow-up of patients after acute kidney injury: patterns of renal functional recovery . PLoS One 2012 ; 7 : e36388
Wald R , Shariff SZ , Adhikari NKJ et al. The association between renal replacement therapy modality and long-term outcomes among critically ill adults with acute kidney injury . Crit Care Med 2014 ; 42 : 868 – 877
Chawla LS , Eggers PW , Star RA et al. Acute kidney injury and chronic kidney disease as interconnected syndromes . N Engl J Med 2014 ; 371 : 58 – 66
Fiorentino M , Grandaliano G , Gesualdo L et al. Acute kidney injury to chronic kidney disease transition . Contrib Nephrol 2018 ; 193 : 45 – 54
Venkatachalam MA , Weinberg JM , Kriz W et al. Failed tubule recovery, AKI-CKD transition, and kidney disease progression . J Am Soc Nephrol 2015 ; 26 : 1765 – 1776
Chou YH , Huang TM , Pan SY et al. Renin-angiotensin system inhibitor is associated with lower risk of ensuing chronic kidney disease after functional recovery from . Sci Rep 2017 ; 7 : 46518
Ferenbach DA , Bonventre JV. Mechanisms of maladaptive repair after AKI leading to accelerated kidney ageing and CKD . Nat Rev Nephrol 2015 ; 11 : 264 – 276
Chawla LS , Kimmel PL. Acute kidney injury and chronic kidney disease: an integrated clinical syndrome . Kidney Int 2012 ; 82 : 516 – 524
Han WK , Bailly V , Abichandani R et al. Kidney injury molecule-1 (KIM-1): a novel biomarker for human renal proximal tubule injury . Kidney Int 2002 ; 62 : 237 – 244
Hall IE , Yarlagadda SG , Coca SG et al. IL-18 and urinary NGAL predict dialysis and graft recovery after kidney transplantation . J Am Soc Nephrol 2010 ; 21 : 189 – 197
Koyner JL , Shaw AD , Chawla LS et al. Tissue inhibitor metalloproteinase-2 (TIMP-2)⋅IGF-binding protein-7 (IGFBP7) levels are associated with adverse long-term outcomes in patients with AKI . J Am Soc Nephrol 2015 ; 26 : 1747 – 1754
Alge JL , Arthur JM. Biomarkers of AKI: a review of mechanistic relevance and potential therapeutic implications . Clin J Am Soc Nephrol 2015 ; 10 : 147 – 155
See EJ , Jayasinghe K , Glassford N et al. Long-term risk of adverse outcomes after acute kidney injury: a systematic review and meta-analysis of cohort studies using consensus definitions of exposure . Kidney Int 2019 ; 95 : 160 – 172
Coca SG , Singanamala S , Parikh CR. Chronic kidney disease after acute kidney injury: a systematic review and meta-analysis . Kidney Int 2012 ; 81 : 442 – 448
Walther CP , Winkelmayer WC , Deswal A et al. Readmissions after acute kidney injury during left ventricular assist device implantation hospitalization . Am J Nephrol 2020 ; 51 : 172 – 181
Koulouridis I , Price LL , Madias NE , Jaber BL. Hospital-acquired acute kidney injury and hospital readmission: a cohort study . Am J Kidney Dis 2015 ; 65 : 275 – 282
Brar S , Ye F , James M et al. Statin use and survival after acute kidney injury . Kidney Int Reports 2016 ; 1 : 279 – 287
Hirayama A , Goto T , Hasegawa K. Association of acute kidney injury with readmissions after hospitalization for acute exacerbation of chronic obstructive pulmonary disease: a population-based study . BMC Nephrol 2020 ; 21 : 116
Liu KD , Yang J , Tan TC et al. Risk factors for recurrent acute kidney injury in a large population-based cohort . Am J Kidney Dis 2019 ; 73 : 163 – 173
Siew ED , Parr SK , Abdel-Kader K et al. Predictors of recurrent AKI . J Am Soc Nephrol 2016 ; 27 : 1190 – 1200
Holmes J , Geen J , Williams JD et al. Recurrent acute kidney injury: predictors and impact in a large population-based cohort . Nephrol Dial Transplant 2019 Aug 3;gfz155
Harris DG , Koo G , McCrone MP et al. Recurrent kidney injury in critically ill surgical patients is common and associated with worse outcomes . J Trauma Acute Care Surg 2014 ; 76 : 1397 – 1401
Rodrigo E , Suberviola B , Santibáñez M et al. Association between recurrence of acute kidney injury and mortality in intensive care unit patients with severe sepsis . J Intensive Care 2017 ; 5 : 28
Thakar CV , Christianson A , Himmelfarb J , Leonard AC. Acute kidney injury episodes and chronic kidney disease risk in diabetes mellitus . Clin J Am Soc Nephrol 2011 ; 6 : 2567 – 2572
Rodríguez E , Arias-Cabrales C , Bermejo S et al. Impact of recurrent acute kidney injury on patient outcomes . Kidney Blood Press Res 2018 ; 43 : 34 – 44
Wald R. Chronic dialysis and death among survivors of acute kidney injury requiring dialysis . JAMA 2009 ; 302 : 1179
Goldberg A , Kogan E , Hammerman H et al. The impact of transient and persistent acute kidney injury on long-term outcomes after acute myocardial infarction . Kidney Int 2009 ; 76 : 900 – 906
Wu VC , Huang TM , Lai CF et al. Acute-on-chronic kidney injury at hospital discharge is associated with long-term dialysis and mortality . Kidney Int 2011 ; 80 : 1222 – 1230
Schiffl H , Lang SM , Fischer R. Long-term outcomes of survivors of ICU acute kidney injury requiring renal replacement therapy: a 10-year prospective cohort study . Clin Kidney J 2012 ; 5 : 297 – 302
Pannu N , James M , Hemmelgarn B et al. Association between AKI, recovery of renal function, and long-term outcomes after hospital discharge . Clin J Am Soc Nephrol 2013 ; 8 : 194 – 202
Fuchs L , Lee J , Novack V et al. Severity of acute kidney injury and two-year outcomes in critically ill patients . Chest 2013 ; 144 : 866 – 875
Horne KL , Packington R , Monaghan J et al. The effects of acute kidney injury on long-term renal function and proteinuria in a general hospitalised population . Nephron Clin Pract 2014 ; 128 : 192 – 200
Rydén L , Sartipy U , Evans M et al. Acute kidney injury after coronary artery bypass grafting and long-term risk of end-stage renal disease . Circulation 2014 ; 130 : 2005 – 2011
Wu VC , Wu CH , Huang TM et al. Long-term risk of coronary events after AKI . J Am Soc Nephrol 2014 ; 25 : 595 – 605
Rimes-Stigare C , Frumento P , Bottai M et al. Evolution of chronic renal impairment and long-term mortality after de novo acute kidney injury in the critically ill; a Swedish multi-centre cohort study . Crit Care 2015 ; 19 : 221
Xu JR , Zhu JM , Jiang J et al. Risk factors for long-term mortality and progressive chronic kidney disease associated with acute kidney injury after cardiac surgery . Medicine 2015 ; 94 : e2025
Hansen MK , Gammelager H , Jacobsen CJ et al. Acute kidney injury and long-term risk of cardiovascular events after cardiac surgery: a population-based cohort study . J Cardiothorac Vasc Anesth 2015 ; 29 : 617 – 625
Gameiro J , Neves JB , Rodrigues N et al. Acute kidney injury, long-term renal function and mortality in patients undergoing major abdominal surgery: a cohort analysis . Clin Kidney J 2016 ; 9 : 192 – 200
Soliman IW , Frencken JF , Peelen LM et al. The predictive value of early acute kidney injury for long-term survival and quality of life of critically ill patients . Crit Care 2016 ; 20 : 242
Parikh CR , Puthumana J , Shlipak MG et al. Relationship of kidney injury biomarkers with long-term cardiovascular outcomes after cardiac surgery . J Am Soc Nephrol 2017 ; 28 : 3699 – 3707
Ferreiro A , Lombardi R. Acute kidney injury after cardiac surgery is associated with mid-term but not long-term mortality: a cohort-based study . PLoS One 2017 ; 12 : e0181158
Kofman N , Margolis G , Gal-Oz A et al. Long-term renal outcomes and mortality following renal injury among myocardial infarction patients treated by primary percutaneous intervention . Coron Artery Dis 2019 ; 30 : 87 – 92
Arias-Cabrales C , Rodríguez E , Bermejo S et al. Short- and long-term outcomes after non-severe acute kidney injury . Clin Exp Nephrol 2018 ; 22 : 61 – 67
Pourafkari L , Arora P , Porhomayon J et al. Acute kidney injury after non-cardiovascular surgery: risk factors and impact on development of chronic kidney disease and long-term mortality . Curr Med Res Opin 2018 ; 34 : 1829 – 1837
Lee S , Park S , Kang MW et al. Postdischarge long-term cardiovascular outcomes of intensive care unit survivors who developed dialysis-requiring acute kidney injury after cardiac surgery . J Crit Care 2019 ; 50 : 92 – 98
Mizota T , Dong L , Takeda C et al. Transient acute kidney injury after major abdominal surgery increases chronic kidney disease risk and 1-year mortality . J Crit Care 2019 ; 50 : 17 – 22
Bhatraju PK , Zelnick LR , Chinchilli VM et al. Association between early recovery of kidney function after acute kidney injury and long-term clinical outcomes . JAMA Netw Open 2020 ; 3 : e202682
Cheng W , Wu X , Liu Q et al. Post-contrast acute kidney injury in a hospitalized population: short-, mid-, and long-term outcome and risk factors for adverse events . Eur Radiol 2020 ; 30 : 3516 – 3527
Gameiro J , Carreiro C , Fonseca JA et al. Acute kidney disease and long-term outcomes in critically ill acute kidney injury patients with sepsis: a cohort analysis . Clin Kidney J 2020
Lea J. The relationship between magnitude of proteinuria reduction and risk of end-stage renal disease . Arch Intern Med 2005 ; 165 : 947 – 53
Chronic Kidney Disease Prognosis Consortium, Matsushita K, van der Velde M, Astor BC et al . Association of estimated glomerular filtration rate and albuminuria with all-cause and cardiovascular mortality in general population cohorts: a collaborative meta-analysis . Lancet 2010 ; 375 : 2073 – 2081
Wu VC , Huang TM , Wu PC et al. Preoperative proteinuria is associated with long-term progression to chronic dialysis and mortality after coronary artery bypass grafting surgery . PLoS One 2012 ; 7 : e27687
Parr SK , Matheny ME , Abdel-Kader K et al. Acute kidney injury is a risk factor for subsequent proteinuria . Kidney Int 2018 ; 93 : 460 – 469
Bihorac A , Yavas S , Subbiah S et al. Long-term risk of mortality and acute kidney injury during hospitalization after major surgery . Ann Surg 2009 ; 249 : 851 – 858
Harel Z , Bell CM , Dixon SN et al. Predictors of progression to chronic dialysis in survivors of severe acute kidney injury: a competing risk study . BMC Nephrol 2014 ; 15 : 114
Lai CF , Wu VC , Huang TM et al. the National Taiwan University Hospital Study Group on Acute Renal Failure (NSARF). Kidney function decline after a non-dialysis-requiring acute kidney injury is associated with higher long-term mortality in critically ill survivors . Crit Care 2012 ; 16 : R123
Legrand M , Rossignol P. Consequences of acute kidney injury . N Engl J Med 2020 ; 382 : 2238 – 2247
Odutayo A , Wong CX , Farkouh M et al. AKI and long-term risk for cardiovascular events and mortality . J Am Soc Nephrol 2017 ; 28 : 377 – 387
Bagshaw SM , Hoste EA , Braam B et al. Syndrome type 3: pathophysiologic and epidemiologic considerations . Cardiorenal In, 2013 , 137 – 157 .
Di Lullo L , Reeves PB , Bellasi A et al. Cardiorenal syndrome in acute kidney injury . Semin Nephrol 2019 ; 39 : 31 – 40
Hsu C , Hsu RK , Yang J et al. Elevated BP after AKI . J Am Soc Nephrol 2016 ; 27 : 914 – 923
Spurgeon-Pechman KR , Donohoe DL , Mattson DL et al. Recovery from acute renal failure predisposes hypertension and secondary renal disease in response to elevated sodium . Am J Physiol Physiol 2007 ; 293 : F269 – F278
Ou SM , Chu H , Chao PW et al. Long-term mortality and major adverse cardiovascular events in sepsis survivors a nationwide population-based study . Am J Respir Crit Care Med 2016 ; 194 : 209 – 217
Coca SG , Yusuf B , Shlipak MG et al. Long-term risk of mortality and other adverse outcomes after acute kidney injury: a systematic review and meta-analysis . Am J Kidney Dis 2009 ; 53 : 961 – 973
Fiorentino M , Tohme FA , Wang S et al. Long-term survival in patients with septic acute kidney injury is strongly influenced by renal recovery . PLoS One 2018 ; 13 : e0198269
Silver SA , Harel Z , McArthur E et al. Causes of death after a hospitalization with AKI . J Am Soc Nephrol 29 : 1001 – 1010
Stads S , Fortrie G , van Bommel J et al. Impaired kidney function at hospital discharge and long-term renal and overall survival in patients who received CRRT . Clin J Am Soc Nephrol 2013 ; 8 : 1284 – 1291
Rimes-Stigare C , Frumento P , Bottai M et al. Long-term mortality and risk factors for development of end-stage renal disease in critically ill patients with and without chronic kidney disease . Crit Care 2015 ; 19 : 383
Fortrie G , Stads S , Aarnoudse AJH et al. Long-term sequelae of severe acute kidney injury in the critically ill patient without comorbidity: a retrospective cohort study . PLoS One 2015 ; 10 : e0121482
Karsanji DJ , Pannu N , Manns BJ et al. Disparity between nephrologists’ opinions and contemporary practices for community follow-up after AKI hospitalization . Clin J Am Soc Nephrol 2017 ; 12 : 1753 – 1761
Silver SA , Siew ED. Follow-up care in acute kidney injury: lost in transition . Adv Chronic Kidney Dis 2017 ; 24 : 246 – 252
Saran R , Li Y , Robinson B et al. US renal data system 2015 annual data report: epidemiology of kidney disease in the United States . Am J Kidney Dis 2016 ; 67 : A7 – A8
Harel Z , Wald R , Bargman JM et al. Nephrologist follow-up improves all-cause mortality of severe acute kidney injury survivors . Kidney Int 2013 ; 83 : 901 – 908
Moore PK , Hsu RK , Liu KD. Management of acute kidney injury: core curriculum 2018 . Am J Kidney Dis 2018 ; 72 : 136 – 1418
Kashani K , Rosner MH , Haase M et al. Quality improvement goals for acute kidney injury . Clin J Am Soc Nephrol 2019 ; 14 : 941 – 953
Hodgson LE , Selby N , Huang TM et al. The role of risk prediction models in prevention and management of AKI . Semin Nephrol 2019 ; 39 : 421 – 430
Chen TK , Knicely DH , Grams ME. Chronic kidney disease diagnosis and management . JAMA 2019 ; 322 : 1294
Vanmassenhove J , Vanholder R , Lameire N. Points of concern in post acute kidney injury management . Nephron 2018 ; 138 : 92 – 103
Brar S , Ye F , James MT et al. for the Interdisciplinary Chronic Disease Collaboration. Association of angiotensin-converting enzyme inhibitor or angiotensin receptor blocker use with outcomes after acute kidney injury . JAMA Intern Med 2018 ; 178 : 1681
Gayat E , Hollinger A , Cariou A et al. Impact of angiotensin-converting enzyme inhibitors or receptor blockers on post-ICU discharge outcome in patients with acute kidney injury . Intensive Care Med 2018 ; 44 : 598 – 605
Hsu C , Liu KD , Yang J et al. Renin-angiotensin system blockade after acute kidney injury (AKI) and risk of recurrent AKI . Clin J Am Soc Nephrol 2020 ; 15 : 26 – 34
Zhang X , Donnan PT , Bell S et al. Non-steroidal anti-inflammatory drug induced acute kidney injury in the community dwelling general population and people with chronic kidney disease: systematic review and meta-analysis . BMC Nephrol 2017 ; 18 : 256
Baker M , Perazella MA. NSAIDs in CKD: are they safe? Am J Kidney Dis 2020
Hung AM , Siew ED , Wilson OD et al. Risk of hypoglycemia following hospital discharge in patients with diabetes and acute kidney injury . Dia Care 2018 ; 41 : 503 – 512
Perkovic V , Jardine MJ , Neal B et al. Canagliflozin and renal outcomes in type 2 diabetes and nephropathy . N Engl J Med 2019 ; 380 : 2295 – 2306
Sawhney S , Beaulieu M , Black C et al. Predicting kidney failure risk after acute kidney injury among people receiving nephrology clinic care . Nephrol Dial Transplant 2020 ; 35 : 836 – 845
Coca SG , Garg AX , Thiessen-Philbrook H et al. Urinary biomarkers of AKI and mortality 3 years after cardiac surgery . J Am Soc Nephrol 2014 ; 25 : 1063 – 1071
ClinicalTrials.gov . Nephrologist follow-up versus usual care after an acute kidney injury hospitalization (FUSION). NCT02483039.
ClinicalTrials.gov . Improving post discharge care after acute kidney injury (AFTER AKI) . NCT02915575.
- renal function
- kidney failure, chronic
- renal failure, acute
Email alerts
Citing articles via.
- ckj Twitter
- ERA Twitter
- ERA Facebook
- ERA Instagram
- ERA LinkedIn
- ERA Youtube
Affiliations
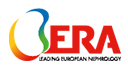
- Online ISSN 2048-8513
- Print ISSN 2048-8505
- Copyright © 2024 European Renal Association
- About Oxford Academic
- Publish journals with us
- University press partners
- What we publish
- New features
- Open access
- Institutional account management
- Rights and permissions
- Get help with access
- Accessibility
- Advertising
- Media enquiries
- Oxford University Press
- Oxford Languages
- University of Oxford
Oxford University Press is a department of the University of Oxford. It furthers the University's objective of excellence in research, scholarship, and education by publishing worldwide
- Copyright © 2024 Oxford University Press
- Cookie settings
- Cookie policy
- Privacy policy
- Legal notice
This Feature Is Available To Subscribers Only
Sign In or Create an Account
This PDF is available to Subscribers Only
For full access to this pdf, sign in to an existing account, or purchase an annual subscription.
Click through the PLOS taxonomy to find articles in your field.
For more information about PLOS Subject Areas, click here .
Loading metrics
Open Access
Peer-reviewed
Research Article
Acute kidney injury and its progression in hospitalized patients—Results from a retrospective multicentre cohort study with a digital decision support system
Contributed equally to this work with: Thea Sophie Kister, Johannes Remmler
Roles Conceptualization, Data curation, Formal analysis, Investigation, Methodology, Validation, Visualization, Writing – original draft
Affiliation Institute of Laboratory Medicine, Clinical Chemistry and Molecular Diagnostics (ILM), University of Leipzig Medical Center, Leipzig, Germany

Roles Data curation, Formal analysis, Validation, Visualization, Writing – review & editing
Roles Writing – review & editing
Roles Funding acquisition, Project administration, Resources, Writing – review & editing
Affiliation Muldentalkliniken GmbH Non-Profit Company, Hospital Grimma and Wurzen, Grimma, Germany
Affiliation Medical Department III, Division of Nephrology, University of Leipzig Medical Center, Leipzig, Germany
Roles Software, Writing – review & editing
Affiliation Bereich 1 –Informationsmanagement, University of Leipzig Medical Center, Leipzig, Germany
Affiliation Xantas AG, Leipzig, Germany
Roles Funding acquisition, Project administration, Software, Writing – review & editing
Roles Conceptualization, Funding acquisition, Project administration, Resources, Software, Supervision, Writing – review & editing
* E-mail: [email protected]
- Thea Sophie Kister,
- Johannes Remmler,
- Maria Schmidt,
- Martin Federbusch,
- Felix Eckelt,
- Berend Isermann,
- Heike Richter,
- Markus Wehner,
- Uwe Krause,
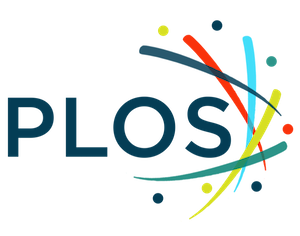
- Published: July 12, 2021
- https://doi.org/10.1371/journal.pone.0254608
- Peer Review
- Reader Comments
In this retrospective multicentric cohort study, we evaluate the potential benefits of a clinical decision support system (CDSS) for the automated detection of Acute kidney injury (AKI). A total of 80,389 cases, hospitalized from 2017 to 2019 at a tertiary care hospital (University of Leipzig Medical Center (ULMC)) and two primary care hospitals (Muldentalkliniken (MTL)) in Germany, were enrolled. AKI was defined and staged according to the Kidney disease: improving global outcomes (KDIGO) guidelines. Clinical and laboratory data was automatically collected from electronic patient records using the frameworks of the CDSS. In our cohort, we found an overall AKI incidence proportion of 12.1%. We identified 6,393/1,703/1,604 cases as AKI stage 1/2/3 (8.0%/2.1%/2.0%, respectively). Administrative coding with N17 (ICD-10-GM) was missing in 55.8% of all AKI cases with the potential for additional diagnosis related groups (DRG) reimbursement of 1,204,200 € in our study. AKI was associated with higher hospital mortality, increased length of hospitalisation and more frequent need of renal replacement therapy. A total of 19.1% of AKI cases (n = 1,848) showed progression to higher AKI stages (progressive AKI) during hospitalization. These cases presented with considerably longer hospitalization, higher rates of renal replacement therapy and increased mortality (p<0.001, respectively). Furthermore, progressive AKI was significantly associated with sepsis, shock, liver cirrhosis, myocardial infarction, and cardiac insufficiency. AKI, and especially its progression during hospitalization, is strongly associated with adverse outcomes. Our automated CDSS enables timely detection and bears potential to improve AKI outcomes, notably in cases of progressive AKI.
Citation: Kister TS, Remmler J, Schmidt M, Federbusch M, Eckelt F, Isermann B, et al. (2021) Acute kidney injury and its progression in hospitalized patients—Results from a retrospective multicentre cohort study with a digital decision support system. PLoS ONE 16(7): e0254608. https://doi.org/10.1371/journal.pone.0254608
Editor: Bhagwan Dass, University of Florida, UNITED STATES
Received: March 10, 2021; Accepted: June 29, 2021; Published: July 12, 2021
Copyright: © 2021 Kister et al. This is an open access article distributed under the terms of the Creative Commons Attribution License , which permits unrestricted use, distribution, and reproduction in any medium, provided the original author and source are credited.
Data Availability: Our data are available upon request. Although data are pseudonymised, details such as the combination of sex, age, diagnoses, and period of assessment at ULMC and MTL could potentially be used to identify individual cases and patients. Therefore, in accordance with the General Data Protection Regulation, we are restrained regarding the public release of our data set. When administrative and legal requirements are met, trusted research institutions may request data access through the current director of the Institute of Laboratory Medicine, Clinical Chemistry and Molecular Diagnostics Leipzig ( [email protected] ) or the AMPEL project directly ( [email protected] ).
Funding: We acknowledge support from Leipzig University for Open Access Publishing. This study is a part of the AMPEL project, which is co-financed through public funds according to the budget decided by the Saxon State Parliament (RL eHealthSax 2017/18, grant No.100331796, URL: https://www.sachsen.de ). The funder provided support in the form of salaries for authors MS and FE, but did not have any additional role in the study design, data collection and analysis, decision to publish, or preparation of the manuscript. The specific roles of these authors are articulated in the ‘author contributions’ section. Coauthors JMT and AK are employees of Xantas Ag. The company receives funding by RL eHealthSax 2017/18 for the sole purpose of technically implementing a CDSS, which they may use commercially. Yet, they did not participate in the preparation of the frameworks, study design, data collection and analysis or the decision to publish. During the preparation of the manuscript, they contributed to the development of the software, review and editing. The specific roles of these authors are articulated in the ‘author contributions’ section.
Competing interests: The authors have declared that no competing interests exist. The affiliation with Xantas AG is not commercial. AK and JMT are employees of Xantas AG. The company receives funding by RL eHealthSax 2017/18 for the sole purpose of technically implementing a CDSS, which they may use commercially. Yet, they did not participate in the preparation of the frameworks, study design, data collection and analysis or the decision to publish. There are no patents, products in development or marketed products associated with this research to declare. This does not alter our adherence to PLOS ONE policies on sharing data and materials.
Introduction
Acute kidney injury (AKI) is a common and serious clinical event that affects up to 15.0% of all hospitalized and up to 50.0% intensive care unit patients [ 1 ]. In studies using definitions conforming to the Kidney disease: improving global outcomes criteria (KDIGO), the pooled rate of AKI was 23.2% worldwide, and the AKI-associated mortality was 23.0% [ 2 ]. AKI is associated with an increased short- and long-term mortality, as well as the development of chronic kidney disease (CKD) [ 3 ]. Pre-existing CKD has been identified as a risk factor for AKI associated with increased mortality, in part due to the increased risk of AKI-associated end-stage renal disease [ 4 ]. According to the KDIGO Clinical Practice Guidelines, AKI is defined by an increased serum creatinine (SCr) and/or reduced urine output, separated into three different stages of severity ( Table 1 ) [ 5 ]. Even an increase of only 26.5 μmol/l in SCr (AKI stage 1) is correlated with a significant risk of mortality and morbidity [ 6 ], requiring determination of the underlying cause in a timely manner. Patients should be managed according to their susceptibilities and an individualized therapy based on patient risk has to be conducted [ 5 , 7 ]. However, due to the complexity of the AKI definition, diagnosis could be delayed or even overlooked.
- PPT PowerPoint slide
- PNG larger image
- TIFF original image
https://doi.org/10.1371/journal.pone.0254608.t001
Study aims and objectives
For this reason, we developed an automated diagnostic system that identifies patients with AKI immediately after laboratory diagnostics as a part of a digital clinical decision support system (CDSS). To evaluate the system, we applied it retrospectively to all hospitalized patients during the years 2017 to 2019 and analysed the incidence proportion of AKI and the distribution of the three stages at a university hospital and two regional hospitals. Thus, we analysed differences in AKI reporting between a tertiary care hospital and primary care institutions. Furthermore, administrative coding [ 8 ] and the involvement of nephrologists [ 9 ] in AKI diagnosis was investigated. In particular, cases with further decrease in kidney function, and therefore, a progression to a higher AKI stage of severity during hospitalization (progressive AKI) were analysed in more detail.
Materials and methods
Study population.
In this retrospective observational cohort study, all hospitalized cases (n = 227 194) admitted between 1 st January 2017 and 31 st December 2019 at the University of Leipzig Medical Center (ULMC, 1 451 beds) and the Muldentalkliniken (MTL, hospital locations Grimma (177 beds) and Wurzen (178 beds) were analysed, including repeated hospital admissions. The ULMC is a tertiary care hospital with its own department of nephrology, several internists with specialization in nephrology and an inpatient dialysis department.
MTL hospitals are primary health care institutions without a nephrology department or internists specialized in nephrology. Instead, they cooperate with an outpatient dialysis centre (KfH, board of trustees for dialysis and kidney transplantation) in Grimma. The study population consisted of all adult patients (≥18 years) (n = 190 752 cases) who received at least two creatinine measurements (n = 82 225 cases). Patients with diagnosis coded according the International Statistical Classification of Diseases and Related Health Problems–German Modification (ICD-GM) N18.5 (dialysis-dependent chronic kidney disease) [ 10 ] were excluded from our study ( Fig 1 , n = 80 389 cases remaining as study cohort). Cases with kidney transplantation in patients’ history were not excluded unless the diagnosis “N18.5 (dialysis-dependent chronic kidney disease)” was also coded. This study has been approved by the Ethics Committee of the Medical Faculty of the University of Leipzig, Germany (No. 214/18-ek). AKI was defined and staged according to the KDIGO Clinical Practice Guideline for Acute Kidney Injury ( Table 1 ) [ 5 ].
https://doi.org/10.1371/journal.pone.0254608.g001
Acquisition of data
Clinical and laboratory data was automatically collected from electronic patient records within the clinic information system using the frameworks of the AMPEL system [ 11 ] ( www.ampel.care ), a CDSS, to monitor laboratory results. AMPEL was recently implemented at ULMC and MTL. Within the system, follow-up treatments and examinations are monitored, and patients’ caregivers are notified when life threatening conditions have been detected (Walter Costa MB et al. The Clinical Decision Support System AMPEL for Laboratory Diagnostics: Implementation and Technical Evaluation. Journal of Medical Internet Research, minor revision). Extracted data included age, sex and creatinine measurements (together with estimated glomerular filtration rates (GFR)). Additionally, relevant diagnoses/comorbidities [ 1 ], which are coded based on ICD-10-GM [ 10 ], and case outcomes (lengths of hospitalization, hospital mortality, eGFR on discharge, presence of nephrological consultations, at least one renal replacement therapy or renal replacement therapy within 72 hours of discharge) were retrieved. We used a second data source (the laboratory information system) to independently validate the automated AKI detection by the AMPEL system. All data were pseudonymized for analysis. The retrospective use of medical records was approved by the ethics committee and works according to an opt-out system. Hence, no additional informed consent was required.
AKI was defined and staged based on absolute and relative changes of creatinine levels within the case, according to KDIGO-criteria, with the lowest sCr level of the last 168 hours serving as a baseline in this definition ( Table 1 ). Urine excretion as an AKI criterion was not available. All creatinine measurements were performed in serum on a Cobas 8000 Analyzer (Roche, Mannheim, Germany; Creatinine Plus Ver. 2 kit, enzymatic method). The highest measured AKI stage determined the classification into the three severities. The progression of AKI was classified according to the first and the maximum determined AKI stage. eGFR was estimated using the Chronic Kidney Disease Epidemiology Collaboration equation (CKD-EPI) [ 12 ] without consideration of ethnicity.
A case-based analysis was performed using Microsoft Excel for Office 365 ProPlus (Microsoft Corporation, Redmond, USA). Further statistical analysis was performed using SPSS 25 (SPSS Inc., Chicago, USA), MedCalc 12 (MedCalc Software bvba, Ostend, Belgium) and R 4.0.2.
The characteristics of the cohort are shown as median and interquartile ranges (IQR). Mann-Whitney U test was used to compare continuous characteristics between two groups, and Pearson’s chi-squared test was used to compare categorical data.
Study cohort characteristics and differences between primary and tertiary care centres
Between 1 st January 2017 and 31 st December 2019, 80 389 (62 932 at ULMC and 17 457 at MTL) hospitalized cases met the inclusion criteria ( Fig 1 ) . In our cohort we found a creatinine-based AKI incidence proportion of 12.1% (9 700 of 80 389 cases), which was higher in the tertiary care hospital (ULMC) (12.4%) than in MTL (10.9%) (p<0.001) ( Table 2 ). Considering the basic case characteristics, we observed significant differences in the median age: ULMC: 65, MTL: 78 (p<0.001). Women were underrepresented at ULMC, but overrepresented at MTL. Regarding the case outcomes, patients were hospitalized for a median of 8 days at ULMC and 7 days at MTL (p<0.001). The overall hospital mortality was 4.8% without significant differences between the two types of hospitals (p = 0.359). We observed a lower initial eGFR in cases at MTL (49 ml/min), compared to cases at ULMC (76 ml/min) (p<0.001). The frequency of need for at least one renal replacement therapy differed significantly between ULMC (2.8%) and MTL (0.8%) (p<0.001).
https://doi.org/10.1371/journal.pone.0254608.t002
Comparison of AKI cases with non-AKI cases and in-between AKI stages
We identified 6 393 cases as AKI stage 1 (AKIN1) (8.0%), 1 703 as AKI stage 2 (AKIN2) (2.1%) and 1 604 as AKI stage 3 (AKIN3) (2.0%) ( Table 3 ). Median patient age of AKI cases was 5 years higher than that of non-AKI cases, whereas among AKI cases, higher stages were associated with younger age. eGFR at discharge did not differ significantly between AKIN1 and AKIN2 cases (p = 0.147), although it was lower in AKIN3 cases (p<0.001). The frequency of renal replacement therapy (at least one dialysis as well as dialysis within 72 hours of discharge) also increased with the AKI stages ( Table 3 ). Additionally, the median length of hospitalization, as well as the hospital mortality, rose with AKI stage (Figs 2 and 3 ). Notably, in comparison to non-AKI cases, the length of hospitalization, the hospital mortality and need of renal replacement therapy were higher in AKI cases, whereas the eGFRs (first and before discharge) were lower ( Table 3 ). We observed associations between selected comorbidities and AKI. The incidence proportions of hypertension, diabetes mellitus, exsiccosis, shock, coronary heart disease, myocardial infarction, cardiac insufficiency, sepsis, and liver cirrhosis were higher in cases with AKI than in non-AKI cases.
Hospital mortality in % for progressive and non-progressive AKI. Patients were divided into six groups according to the first and maximum detected AKI stage, which is equal for non-progressive AKI (AKIN1, AKIN2, AKIN3) and given as AKIN X to Y (X = first and Y = max AKI stage) for progressive AKI.
https://doi.org/10.1371/journal.pone.0254608.g002
Kaplan-Meier curves for the first 42 days of hospitalisation after first AKI detection. Patients were divided into six groups according to first and maximum detected AKI stage, which is equal for non-progressive AKI (AKIN1, AKIN2, AKIN3) and given as AKIN X to Y (X = first and Y = max AKI stage) for progressive AKI. Censored survival data due to hospital discharge are indicated by +. Median lengths of hospitalisation were 14, 23, 15, 26, 22, 15 days for AKIN1, AKIN1 to 2, AKIN2, AKIN1 to 3, AKIN2 to 3 and AKIN3, respectively.
https://doi.org/10.1371/journal.pone.0254608.g003
https://doi.org/10.1371/journal.pone.0254608.t003
Administrative coding of AKI
Overall, we noted a high absence of administrative coding as N17 (ICD-10-GM code for Acute kidney failure) in AKIN1 (68.1%), AKIN2 (41.0%) and AKIN3 (22.3%) cases ( Table 4 ). Yet, almost every AKI was followed up with at least one creatinine measurement after its detection (ULMC/MTL in 88.4%/81.1% of the cases, respectively). The frequency of follow-up and coding increased with higher AKI stages. In 5.3% of all non-AKI cases, N17 was coded. Considering the German diagnosis and procedure related reimbursement system, additional revenues of 1 204 200 € could have been generated within the three years by correct administrative coding of the cases within our cohort.
https://doi.org/10.1371/journal.pone.0254608.t004
Progressive AKI and its clinical implications
In a second step, we compared cases with progressive AKI (AKIN1→AKIN2, AKIN1→AKIN3, AKIN2→AKIN3) to those without disease progression during hospitalization (AKIN1→AKIN1, AKIN2→AKIN2, AKIN3→AKIN3, first→maximum AKI) ( Table 5 and S1 Table ). Although progressive AKI was evident in 1,848 cases (19.1% of all AKI cases), these were likely to be younger in median age (p<0.001) and predominantly male (p = 0.006). As progressive AKI was associated with longer hospitalisation and higher rates of renal replacement therapy and mortality, the overall outcome of patients with progressive AKI was worse, compared to cases without progression. Especially with regard to mortality, progressive AKI seems to be a significant prognosis factor (Figs 2 and 3 ). This is also reflected in higher rate of intermediate or intensive care among cases with progressive AKI and reflects also in higher administrative N17 coding. In a minority of cases, completely documented nephrological consultations occurred and were more often conducted in cases with progression (analysis for ULMC only). Cases with progressive AKI showed significantly higher incidence proportions for certain comorbidities; we observed a strong association with sepsis, shock, liver cirrhosis, myocardial infarction, and cardiac insufficiency ( Table 5 and S1 Table ).
https://doi.org/10.1371/journal.pone.0254608.t005
In this study, we confirmed a high frequency of AKI among our cohort and demonstrated its association with severe comorbidities and increased mortality during hospitalization. In line with previous studies, we reported a creatinine-based AKI incidence proportion of 12.1%. Studies published after the implementation of the KDIGO guidelines in 2012 have found incidences ranging from 10.7% [ 2 ], 15.3% [ 13 ], 18.3% [ 14 ], 22.7% [ 15 ], 23.2% [ 2 ] to 31.3% [ 8 ].
Despite its high incidence, AKI is still an underrated disease. In our study, we observed low rates of administrative coding, a phenomenon which was also observed in other studies [ 8 , 16 – 19 ]. This may indicate insufficient consideration or detection of the disease, which could contribute to worse outcomes, given that documentation/coding is associated with improved outcomes after adjustment for AKI severity [ 16 ]. Additionally, the financial aspect of a correct and complete coding is evident, considering the German diagnosis and procedure related reimbursement system.
To the best of our knowledge, our study is the first comparative analysis of AKI between a tertiary care hospital and two primary care institutions in Germany, and we found comparable AKI incidences proportions. However, patients in these sites differed significantly in age and sex. Age differences are easily explained by rural and urban demographics within the catchment area [ 20 ]. This may also partly explain the lower eGFRs at admission and discharge at MTL [ 21 ]. Additionally, stricter indication for follow-up measurements at MTL could have caused a selection bias towards patients in worse conditions, as a second creatinine measurement (part of our inclusion criteria) was performed more frequent at ULMC (45.2% of all hospitalized cases, compared to 35.8% at MTL). At ULMC, the rates of renal replacement therapy were more than twice as high compared to those at MTL, which is most likely due to a missing department of nephrology and in-house dialysis centre at MTL, where critically-ill patients with a renal disease are transferred to another hospital in most cases. Interestingly, the hospital mortality within our cohort did not differ significantly between the two sites.
The presence, as well as the stage of AKI, was strongly associated with the case outcomes in our cohort. Thereby, our results confirmed those of previous studies showing that cases with AKI had longer lengths of hospitalization, higher rates of renal replacement therapy and a higher risk of admission to intensive care units as well as higher hospital mortality than cases without AKI [ 13 ]. These outcome measures worsened with increasing AKI stage, in accordance with the literature [ 18 , 22 ]. Of note, in our study, are the substantial differences between non-AKI and AKIN1 cases, matching findings that even modest changes in SCr are associated with higher mortality [ 6 ] ( Table 3 ). AKI is associated with several comorbidities across several medical conditions, and may represent an additional risk factor in affected patients. Hypertension [ 23 ], diabetes mellitus [ 24 ], volume depletion such as exsiccosis and shock [ 25 ], cardiological complications (e.g. coronary heart disease, myocardial infarction and cardiac insufficiency [ 26 ]), sepsis [ 27 ] and liver cirrhosis [ 28 ] were often discovered alongside AKI. In our cohort, we confirmed, in particular, a strong association of AKI with sepsis and shock ( Table 3 ). Regarding eGFRs (first eGFR as well as eGFR at discharge), we observed higher values in AKIN2 compared to AKIN1 ( Table 3 ). This unexpected finding could be explained by the limitation of the KDIGO criteria: AKIN1 is defined combinatorically by a relative (SCr > 50% over 7 days) and an absolute (SCr > 0.3mg/dL over 48h) criterion for SCr dynamics [ 29 ], whereas for AKIN2, only the relative criterion (SCr > 100% over 7 days) is relevant ( Table 1 ). The absolute criterion is reached faster in patients with high baseline SCr. Similarly, the overrepresentation of male sex among our AKI cases might have presented another limitation to the absolute criterion (SCr > 0.3mg/dL over 48h): lower muscle mass and associated lower SCr levels could underestimate the degree of renal failure in women and elderly patients [ 30 ].
To the best of our knowledge, our study is the first to distinguish between AKI cases with and without progression to a higher stage during hospitalization, with respect to characteristics and outcomes of these cases. In our cohort, 19.1% of all AKI cases showed a progression to a higher stage. For these patients, we discovered considerable worse outcomes, especially concerning hospital mortality. Cases with a progressive AKI were also associated with higher rates of renal replacement therapy, longer lengths of hospitalization and more frequent intensive care treatments. Progressive AKI is strongly associated with the highly relevant comorbidities sepsis and shock, as well as liver cirrhosis, myocardial infarction, and cardiac insufficiency. A distinctive restriction of the circulation and, therefore, a disturbed perfusion as a common effect of these diseases could be assumed [ 31 , 32 ]. Progressive AKI indicates a significantly worse prognosis. Thus, an immediate detection of any AKI and further medical work-up of the patient according to the KDIGO guideline seem urgently necessary and might prevent progressive AKI and further complications. However, due to the complicated criteria, timely identification of these patients is difficult in routine hospital care workflow. Our AMPEL-CDSS can identify these patients in a timely manner and notify clinicians immediately. Consequently, we are planning a prospective trial to verify that this CDSS can improve renal and other clinical outcomes. In the process of diagnosis and therapy, documented nephrological consultations rarely occurred in our cohort. If consultations were ordered, they were often delayed. Balasubramanian et al . showed a significant risk reduction of further kidney function deterioration if renal consultations took place within 18 hours of AKI-onset [ 9 , 33 ]. Delayed consultations in our cohort might have been due to missed diagnosis and a lack of consciousness of the significance of timely intervention [ 34 ] and could be improved by the AMPEL-CDSS as well.
Limitations of our study are its retrospective nature and the AKI definition solely based on serum creatinine dynamics. Urine excretion as an AKI criterion was not included, as urine excretion is often not systematically documented. This might cause an underestimation of the AKI incidence proportion, which is reflected by the fact that 5.3% of all non-AKI cases in our cohort were coded with N17. Furthermore, we exclusively included inpatient cases. Thus, pre-stationary diagnostics and long-term outcome data, such as outpatient deaths, were not available. An analysis of patient transfers from MTL to a tertiary care hospital was not performed.
Nevertheless, our automated approach was necessary to evaluate the potentials of the AMPEL-CDSS (which depends on these automated analysis of limited data) and enabled the investigation of a large cohort in a multicentric manner. Thus, we were able to comparatively analyse AKI cases on two different levels of medical care in Germany. Moreover, our study uniquely investigated and proved the substantial prognostic value of a progressive AKI during hospitalization. Our results show the potentials and importance of an automated, real-time detection of AKI and its progression, which might improve patient outcome [ 35 ]. This is the designated aim of our CDSS called AMPEL, which is currently being implemented and will be further evaluated in prospective studies.
Supporting information
S1 table. comparison of the frequency, the patient characteristics, the administrative coding, the case outcomes and the comorbidities between cases with progressive aki and those without..
https://doi.org/10.1371/journal.pone.0254608.s001
- View Article
- PubMed/NCBI
- Google Scholar
- 7. Willam C, John S, Eckardt K-U KDIGO-Leitlinien zum akuten Nierenversagen. Bayer. Aztebl.;2015:344–348.
- 10. World Health Organization [2004]: International statistical classification of diseases and related health problems. 10. rev., 2. ed. Geneva: World Health Organization.
- 20. Demografiemonitor. available: https://www.demografie.sachsen.de/monitor/html/atlas.html .
Advertisement

- Previous Issue
- Previous Article
- Next Article
Defining Cardiac Surgery–associated AKI
Epidemiology of cardiac surgery–associated aki, pathogenesis of cardiac surgery–associated aki, predicting cardiac surgery–associated aki, key clinical research for prevention and mitigation of cardiac surgery–associated aki, recent novel exploratory studies of cardiac surgery aki prevention, guidelines and practice updates, acute kidney injury after cardiac surgery: prediction, prevention, and management.

This article has been selected for the Anesthesiology CME Program ( www.asahq.org/JCME2023DEC ). Learning objectives and disclosure and ordering information can be found in the CME section at the front of this issue.
This article is featured in “This Month in Anesthesiology,” page A1.
Submitted for publication June 2, 2023. Accepted for publication August 9, 2023. Published online first on October 9, 2023.
- Split-Screen
- Article contents
- Figures & tables
- Supplementary Data
- Peer Review
- Open the PDF for in another window
- Cite Icon Cite
- Get Permissions
- Search Site
Sreekanth R. Cheruku , Jacob Raphael , Javier A. Neyra , Amanda A. Fox; Acute Kidney Injury after Cardiac Surgery: Prediction, Prevention, and Management. Anesthesiology 2023; 139:880–898 doi: https://doi.org/10.1097/ALN.0000000000004734
Download citation file:
- Ris (Zotero)
- Reference Manager
Acute kidney injury (AKI) is a common complication in cardiac surgery patients, with a reported incidence of 20 to 30%. The development of AKI is associated with worse short- and long-term mortality, and longer hospital length of stay. The pathogenesis of cardiac surgery–associated AKI is poorly understood but likely involves an interplay between preoperative comorbidities and perioperative stressors. AKI is commonly diagnosed by using increases in serum creatinine or decreased urine output and staged using a standardized definition such as the Kidney Disease Improving Global Outcomes classification. Novel biomarkers under investigation may provide earlier detection and better prediction of AKI, enabling mitigating therapies early in the perioperative period. Recent clinical trials of cardiac surgery patients have demonstrated the benefit of goal-directed oxygen delivery, avoidance of hyperthermic perfusion and specific fluid and medication strategies. This review article highlights both advances and limitations regarding the prevention, prediction, and treatment of cardiac surgery–associated AKI.
Approximately 2 million patients undergo cardiac surgery each year, 1 with cardiac surgery–associated acute kidney injury (AKI) occurring in an estimated 20 to 30% of them. 1–3 Acute kidney injury is an abrupt decline in kidney function that occurs over hours and sometimes days and is characterized by a rapid increase in serum creatinine, decrease in urine output, or both. Only a small subset ( i.e. , 2 to 3%) of patients who develop AKI after cardiac surgery require renal replacement therapy. Although cardiac surgeries are done to increase quality of life and survival, the development of cardiac surgery–associated AKI is significantly associated with higher hospitalization costs 4 and with increased short- and long-term postoperative mortality. 3 , 5–7 Several registry and retrospective cohort studies report significant associations between cardiac surgery–associated AKI and later development of chronic kidney disease, 8 end-stage renal disease, 9 heart failure, 10 and major adverse cardiovascular events. 11–14 Given the high incidence of cardiac surgery–associated AKI and its potentially devastating sequelae, there is an urgent clinical need to learn how best to prevent and mitigate cardiac surgery–associated AKI.
This review article highlights both advances and limitations in present medical knowledge regarding effective preventions, risk predictions and subphenotypes, and treatments of cardiac surgery–associated AKI. This review also touches on some new and exploratory preventive or therapeutic approaches to cardiac surgery–associated AKI that could be assessed in future randomized clinical trials. Finally, we will summarize recommendations outlined in recent guidelines and clinical practice updates 15 , 16 regarding areas of perioperative practice that may prevent or mitigate cardiac-surgery AKI and its sequelae.
Accurate prediction of cardiac surgery–associated AKI requires a consistent definition of the AKI outcome that can be compared between studies. Historical definitions used for cardiac surgery–associated AKI have ranged from subtle increases in serum creatinine to the need for new postoperative renal replacement therapy. The Society of Thoracic Surgeons defines AKI as a 3-fold increase in serum creatinine, creatinine more than 4 mg/dL, or initiation of dialysis after cardiac surgery. Eventually, clinicians and researchers began to adopt more uniform definitions of AKI, with the creation of the Risk, Injury, Failure, Loss, and End-stage renal failure (RIFLE) and Acute Kidney Injury Network (AKIN) classifications. Both classifications use elevations in serum creatinine during different periods of time (7 days in RIFLE vs . 48 h in AKIN) and decreases in urine output to categorize AKI into stages. In 2012, the Kidney Disease: Improving Global Outcomes (KDIGO) 17 became the newest classification for AKI, integrating components of both RIFLE and AKIN criteria, and including rises in serum creatinine for both 48 h and 7 days after cardiac surgery. Table 1 reviews the elements of the RIFLE, AKIN, and KDIGO criteria. During the past 10 plus years, the KDIGO criteria have become an established standard for defining cardiac surgery–associated AKI both in clinical practice and in clinical research. However, there are some discrepancies in how the KDIGO criteria are used in cardiac surgery studies. Some of these studies diagnosed AKI using serum creatinine increases alone, while others used both the serum creatinine criteria and the KDIGO criteria for oliguria. 18 These discrepancies exist because some investigators find urine output to be unreliable in the setting of diuretic use.
Diagnostic Criteria for RIFLE, AKIN, and KDIGO

A meta-analysis that included 32 observational studies of cardiac surgery patients published between 2006 and 2014 reported a median pooled incidence for AKI of 22.1%. 19 The incidence of stage 1, 2, and 3 AKI defined by either RIFLE, AKIN, or KDIGO criteria among 19 studies that reported these data was 17.9%, 4.4%, and 3.5%, respectively. Another meta-analysis of 91 observational studies of cardiac surgery patients published between 2004 and 2014 reported a similar pooled incidence rate of AKI of 22.3%. 3 The pooled AKI rate in this study was lower in patients with coronary artery bypass graft (CABG) surgery (19.0%) compared to those who underwent valve surgery (27.5%) or aortic surgery (29.0%). A large epidemiologic study used to create the Mehta score reported that type of surgery is an independent predictor of dialysis-dependent AKI after cardiac surgery with combined CABG-mitral valve surgery (odds ratio 2.57) and mitral valve surgery (odds ratio 2.01) having the highest risk. 20 AKI was also associated with significantly higher length of stay in the intensive care unit (ICU; 5.4 days vs . 2.2 days) and hospital (15.0 days vs . 10.5 days) when compared to patients without AKI. 21
Acute kidney injury–associated in-hospital and long-term (1 to 5 yr) mortality in the latter meta-analysis was 10.7% and 30.0%, respectively. 3 Duration of cardiac surgery–associated AKI has been found to predict mortality in a number of published studies. One meta-analysis of nine observational studies of cardiac surgery patients reported that patients who recovered renal function before hospital discharge had a significantly lower long-term mortality risk compared to patients discharged with persistent abnormal renal function. 21 However, even patients who recovered their renal function after developing AKI in this study had a significantly higher mortality than those who did not develop AKI. A 90-day follow-up of participants of the Randomized Evaluation of Normal versus Augmented Level Replacement Therapy (RENAL) Study, which randomly assigned critically ill patients (including cardiac surgery patients) between receiving higher and lower levels of renal replacement therapy dose intensity, reported an overall mortality of 62.3% among all patients with AKI requiring renal replacement. 22 This was confirmed in a retrospective study of cardiac surgery patients that reported a mortality of 58.6% in patients requiring new postoperative renal replacement therapy. 23
The pathogenesis of cardiac surgery–associated AKI is complex, resulting from a multitude of potential perioperative insults to the kidney. The commonality of these insults is lower oxygen delivery to the kidneys relative to the kidneys’ oxygen demand, resulting in renal tubular injury. The hypothesized mechanisms that contribute to cardiac surgery–associated AKI include hypoperfusion, atheroembolic events, exposure to nephrotoxins, inflammation, and oxidative stress ( fig. 1 ). 24

Pathophysiology of cardiac surgery–associated acute kidney injury.
The kidneys receive 20% of the total cardiac output; however, intrarenal oxygen availability requires an orchestrated balance between supply ( i.e. , blood flow) and demand, which is determined by basal metabolism and tubule-glomerular functions. For example, the renal glomeruli extract only 10 to 20% of the oxygen delivered to them and maintain a low oxygen tension at baseline, 25 which makes them particularly vulnerable to hypoxia during periods of hypoperfusion. Renal hypoperfusion can occur throughout the perioperative period due to hypotension, decreased cardiac output, sympathetic stimulation, the administration of vasoconstrictive medications, and activation of the renin-angiotensin-aldosterone system. Each of these events can interfere with renal autoregulation and reduce glomerular filtration rate. 26
Cardiopulmonary bypass is associated with nonpulsatile flow, altered hemodynamics, decreased oxygen delivery, inflammation, and oxidative stress, each of which can contribute to AKI. 26 Renal perfusion while on cardiopulmonary bypass is directly proportional to mean arterial pressure (MAP) and pump flow rate, suggesting that normal autoregulatory mechanisms may be impaired during this period. 27 Lannemyer et al. 28 found that cardiopulmonary bypass induces renal vasoconstriction and redistribution of blood from the kidneys in the setting of unchanged glomerular filtration rate and renal oxygen consumption. This oxygen supply–demand mismatch may explain why the duration of time, 29 degree of hemodilution, 30 , 31 and hypotension 32 on cardiopulmonary bypass have all been linked to postoperative AKI. Atherosclerotic disease is also prevalent in patients undergoing cardiac surgery and elements of the surgical procedure are associated with atheroembolic events, including placement of intra-aortic balloon pumps, manipulation of the left atrium, and both clamping and declamping of the aorta. One autopsy-based study found that, among patients who died in the hospital after cardiac surgery, 10.4% had atheroembolic findings in the kidneys. 33 Rewarming from cardiopulmonary bypass provides a period of time when the kidney is susceptible to hypoxic injury. During this time, oxygen consumption increases with temperature in the renal medulla and can exceed available supply. 34
Perioperative medications associated with nephrotoxicity include antibiotics, angiotensin-converting enzyme inhibitors, angiotensin receptor blockers, nonsteroidal anti-inflammatory drugs (NSAIDs), diuretics, and intravascular contrast agents ( table 2 ). Commonly used antibiotics, including aminoglycosides, β-lactams, and vancomycin, mediate AKI through immune and nonimmune mechanisms. Aminoglycosides accumulate in proximal tubular cells and cause apoptosis and acute tubular necrosis. 35 β-Lactam antibiotics can cause a hypersensitivity reaction resulting in acute interstitial nephritis and can also mediate crystal development and obstructive injury to the tubules. 35 Vancomycin is thought to cause AKI by promoting free radical formation and oxidative stress resulting in acute tubular necrosis, causing a hypersensitivity reaction resulting in acute interstitial nephritis, and through intratubular crystal obstruction. Among these antibiotics, KDIGO guidelines recommend avoiding aminoglycosides unless a suitable alternative is unavailable. 36 Intravascular contrast agents are associated with acute tubular necrosis due to their cytotoxic effect on tubular cells and by mediating renal vasoconstriction. Because this association is based on observational studies, there is controversy regarding the true incidence of contrast-associated AKI and effective interventions in high-risk patients. Even studies utilizing propensity matching to control for non–contrast-associated etiologies of AKI reported disparate results with one study finding a higher incidence of contrast-associated AKI in patients with impaired baseline kidney function 37 and one study finding no association between contrast exposure and AKI regardless of baseline kidney function. 38 Observational studies of cardiac surgical patients suggest that exposure to intravascular iodinated contrast agents less than 7 days before surgery may result in a higher incidence of postoperative AKI. 39–41 Until data from randomized clinical trials are available, the decision to postpone cardiac surgery after contrast exposure should be based on an individual evaluation of the patient and other risk factors for postoperative AKI. 42
Medication Classes Associated with Nephrotoxicity

Although angiotensin-converting enzyme inhibitors and angiotensin receptor blockers are associated with improved renal blood flow, their preoperative administration is associated with an increased risk of AKI in patients undergoing cardiac surgery. The mechanism for this injury may be functional renal insufficiency, or hypotension resulting in decreased renal perfusion pressure. 43 For this reason, the KDIGO “bundle of care” recommends discontinuing these medications for 48 h after cardiac surgery. 36 Diuretics, including the loop diuretic furosemide and mannitol, have not been found to reduce cardiac surgery–associated AKI, and furosemide may actually increase the risk of AKI by inducing hypovolemia in some patients. 15
Cardiac surgery patients are also susceptible to pre-renal and postrenal causes of AKI throughout the perioperative period. Aggressive preoperative diuresis, hemofiltration during cardiopulmonary bypass, hemorrhage, and vasoplegia can contribute to an absolute or relative hypovolemia and impaired renal perfusion. Postrenal AKI can result from urinary catheter obstruction ( e.g. , from blood clots or kinking of the catheter tubing) or from urinary retention after the catheter is removed in the postoperative period. A thorough review of historical details ( e.g. , diuretic administration, prostatic hyperplasia), physical examination ( e.g. , flushing the urinary catheter, bladder ultrasonography), and laboratory tests ( e.g. , fractional excretion of sodium, fractional excretion of urea) are necessary to distinguish between pre-renal, postrenal, and intrinsically renal etiologies of AKI after cardiac surgery.
Clinical and Perioperative Risk Factors
Identifying patients with modifiable risk factors for cardiac surgery–associated AKI may facilitate interventions to mitigate their risk throughout the perioperative period. Numerous articles have assessed clinical and surgical risk factors for cardiac surgery–associated AKI, but, to date, the variables identified for risk prediction are most accurate for predicting stage 3 AKI, which is renal failure associated with a 3-fold increase in serum creatinine or requiring renal replacement therapy. These models include the Cleveland Clinic score, 44 the Mehta score, 20 and the Simplified Renal Index. 45 Each of these uses preoperative risk factors to predict the risk of severe cardiac surgery–associated AKI, which are listed in table 3 . The Cleveland Clinic score has been validated in a cohort of 12,096 to have the best discriminatory value (area under the receiver operating characteristics curve [AUC]: 0.86) to predict the need for renal replacement therapy after cardiac surgery compared to the Mehta score and the Simplified Renal Index (AUC: 0.81 and 0.79, respectively). 46 Despite this, it has not been widely implemented in clinical practice. Research is being done to improve prediction of cardiac surgery–associated AKI using biomarkers of renal function and renal injury, because predictive models have integrated clinical variables with these biomarkers 47 and incorporated machine learning algorithms 48 to improve clinical risk prediction for cardiac surgery–associated AKI. The greatest limitation of these newer machine learning models is their use of data obtained at static intervals to predict an outcome that is impacted by the multimodal dynamic data during the perioperative period. Future models that incorporate these multimodal dynamic data ( e.g. , perioperative hemodynamics and oxygenation variables, among others) and key biomarkers of kidney health obtained at various time points may be able to better predict all stages of AKI with greater accuracy and within actionable time points to intervene.
Summary of Features Used in Clinical Prediction Models

In terms of identifying demographic and preoperative clinical characteristics that predict overall AKI after cardiac surgery, multiple parameters have been identified from observational studies, although not always consistently. Preoperative risk factors frequently identified include advanced age, 49 , 50 female sex, 49 higher body mass index, 49 , 51 proteinuria 52 and the presence of systemic comorbidities including hypertension, 49 diabetes, 49 , 50 , 53 chronic kidney disease, 42 , 49 , 50 , 53 chronic obstructive pulmonary disease, 49 left ventricular dysfunction, 49 , 53 and perioperative anemia. 49 Patients with chronic kidney disease have lower kidney reserve; hence, during cardiac surgery and cardiopulmonary bypass, the kidney can be overwhelmed by surgical stressors, inflammation, and oxidative stress, resulting in further decline in glomerular filtration rate. Husain-Syed et al . 54 studied patients who underwent CABG surgery at a single center and calculated their preoperative renal functional reserve using a high oral protein load. The authors compared patients who had an in-hospital postoperative increase in serum creatinine and returned to baseline to a group who did not experience a rise in postoperative creatinine. The patients who experienced a transient increase in postoperative serum creatinine that returned to preoperative baseline concentration were subsequently found to have significantly lower renal functional reserve when compared to patients who did not have any rise in serum creatinine after surgery. This illustrates that AKI leaves patients with reduced long-term function and less renal functional margin in the setting of additional subsequent AKI events. Hypertension and diabetes are highly prevalent in patients undergoing cardiac surgery. Chronic hypertension can lead to autoregulatory vasoconstriction of the preglomerular vasculature, which can impair autoregulatory mechanisms and make the kidneys vulnerable to injury when the perfusion pressure is inadequate. 55 Diabetes is associated with vasculopathy and nephropathy resulting from tissue hypoxia, inflammation, endothelial damage, and other mechanisms. Proteinuria is a marker of tubulointerstitial damage, and the resorption of filtered protein in the proximal tubules is associated with upregulation of inflammatory mediators. One study found that preoperative proteinuria was an independent predictor of cardiac surgery–associated AKI requiring renal replacement therapy by AKIN criteria. 52
Urgent surgery, reoperative surgery, and the preoperative placement of an intra-aortic balloon pump are also associated with cardiac surgery–associated AKI diagnosed using RIFLE criteria. Compared to patients undergoing CABG surgery, those undergoing isolated valve or aortic surgery have a higher incidence of cardiac surgery–associated AKI. 49 , 53
Intra-operative and early postoperative risk factors include cardiopulmonary bypass duration, 42 , 49 anemia while on cardiopulmonary bypass, blood transfusion, 49 and development of early postoperative proteinuria. 56 While prolonged cardiopulmonary bypass time has been associated with cardiac surgery–associated AKI, large trials comparing outcomes after on-pump and off-pump CABG surgery have failed to show a reduction in cardiac surgery–associated AKI among patients who underwent off-pump procedures. 57 , 58 Postoperative tissue hypoperfusion defined by elevated serum lactate levels 59 and the use of inotropes 59 , 60 have been associated with cardiac surgery–associated AKI. Additionally, re-exploration after surgery is a strong, independent predictor of cardiac surgery–associated AKI. 49 , 61
Blood and Urine Biomarkers
Current diagnostic criteria for cardiac surgery–associated AKI utilize increases in serum creatinine and decreases in urine output. While creatinine provides a good approximation of glomerular filtration rate when kidney function is normal, its accuracy is diminished in non–steady state conditions such as the perioperative period. The increased volume of distribution in postoperative cardiac surgery patients or in other critically ill populations who are exposed to aggressive fluid resuscitation 62 can lead to dilution of serum creatinine, delaying the diagnosis and underestimating the degree of kidney injury. 63 The inclusion of other AKI-related biomarkers may enable earlier detection and better prediction of cardiac surgery–associated AKI (greater sensitivity and/or specificity) than with clinical and surgical risk factors alone ( fig. 2 ). 47 This may allow discovery of mitigating therapies to prevent the incidence and further progression of AKI. While a number of blood and urine biomarkers have been assessed for prediction of cardiac surgery–associated AKI, many of these perform inconsistently between subpopulations of cardiac surgery patients and only one is approved for clinical use by the U.S. Food and Drug Administration. Additionally, evaluating biomarkers of kidney injury using functional biomarkers such as serum creatinine as clinical endpoints poses a challenge. Biomarker discovery and development is a vast area of research that is beyond the scope of this review, but we include a brief summary of some of the most relevant AKI biomarkers to date. These biomarkers are briefly reviewed in table 4 .
Novel Blood and Urine Biomarkers Being Investigated for Detection of Cardiac Surgery–associated AKI.

Timeline highlighting the potential for novel biomarkers to identify AKI as compared to serum creatinine-based criteria. AKI, acute kidney injury.
Neutrophil gelatinase–associated lipocalin is part of the innate immune system and functions by binding to bacterial iron-binding proteins. It is a sensitive and specific marker of injury to the kidneys and can be detected in the blood and urine after both nephrotoxic and ischemic injuries. While increases in blood and urine neutrophil gelatinase–associated lipocalin concentration have been associated with cardiac surgery–associated AKI in children, 64 a meta-analysis of observational studies found that it had a much lower predictive value in adults undergoing cardiac surgery (AUC 0.83 in adults vs . 0.89 in children) and those with chronic kidney disease (AUC 0.81 in patients with chronic kidney disease vs . 0.87 in patients without chronic kidney disease). 65
Cystatin C is a low molecular weight protein that undergoes complete reabsorption by the renal tubules. Unlike creatinine which is produced by muscle cells and undergoes tubular filtration, cystatin C is produced by all nucleated cells and undergoes glomerular filtration. It has therefore been investigated as a functional biomarker for kidney clearance. A post hoc analysis of a randomized controlled trial of cardiac surgery patients found that an early postoperative rise in serum cystatin C was associated with AKI requiring renal replacement therapy. 66 A meta-analysis evaluating the diagnostic accuracy of cystatin C in predicting AKI in adults in a variety of settings also found that it demonstrated high sensitivity (0.82) and specificity (0.82), with an AUC of 0.89. 67
Urinary interleukin-18 is specific to the renal tubules and is thought to mediate ischemic and inflammatory injury to the kidneys. Urinary levels of interleukin-18 were found to be significantly elevated in patients with cardiac surgery–associated AKI, as early as 4 to 6 h after cardiopulmonary bypass. 66 In cardiac surgery patients, one study found that patients with the highest concentration of urinary interleukin-18 (5th quintile) had a nearly 7-fold incidence of postoperative AKI. The addition of interleukin-18 to a clinical prediction model that included perioperative variables improved its receiver-operating characteristic curve (AUC) from 0.69 to 0.76. 47
Kidney Injury Molecule-1 is a transmembrane protein with two extracellular domains that separate from the cell surface and enter the urine after kidney injury. 68 The urinary concentration of kidney injury molecule-1 peaks as early as 3 h after kidney injury, making it an earlier marker of cardiac surgery–associated AKI compared to serum creatinine. 69 One study evaluating biomarkers in post–cardiac surgery patients found that the combination of kidney injury molecule-1 and interleukin-18 had excellent predictive value (AUC 0.93) for stage 3 AKIN and mortality. 70
Tissue inhibitor of metalloproteinase and insulin-like growth factor-binding protein are inducers of cell cycle arrest in renal tubular cells, which occurs in the very early phases of cellular injury. Urinary concentrations of these biomarkers were found to have a superior sensitivity and specificity in predicting KDIGO stage 2 to 3 AKI when compared to other novel biomarkers. 71 The product of the urinary concentrations of these biomarkers has been approved by the U.S. Food and Drug Administration for early clinical AKI prediction.
C-C motif chemokine ligand 14 is a chemokine that is released from tubular epithelial cells in response to injury. It mediates the renal inflammatory response that results from AKI by binding to receptors on monocytes and T cells and promoting chemotaxis in these cells. 72 An observational study in cardiac surgery patients found that elevated serum C-C motif chemokine ligand 14 was predictive of KDIGO stage 3 AKI (AUC 0.93). Because C-C motif chemokine ligand 14 is a biomarker of kidney disease progression, it has also been investigated as a predictor of chronic kidney disease. One study of ICU patients found that urinary C-C motif chemokine ligand 14 levels were associated with renal nonrecovery. 73
This section highlights results from some key areas that clinical trials and studies have focused on to date for the purposes of preventing or mitigating cardiac surgery–associated AKI.
Rewarming Temperature on Cardiopulmonary Bypass
A study that included two separate randomized controlled trials assigned patients undergoing CABG surgery to rewarming from 32° to 34°C versus 32° to 37°C in one trial (n = 233) and a strategy of mild sustained hypothermia (34°C) versus sustained normothermia (37°C) without rewarming in the second trial (n = 267). 74 These two randomized trials found that rewarming from 32° to 37°C in a 10- to 15-min period resulted in an increased incidence of AKI compared to rewarming to 34°C; however, that sustained mild hypothermia did not have a nephroprotective effect. Newland et al . 75 analyzed a large multicenter registry of cardiac surgery patients using propensity scoring to adjust for confounders and found that the duration of hyperthermic perfusion (greater than 37°C) was an independent predictor of increased cardiac surgery–associated AKI.
Goal-directed Oxygen Delivery on Cardiopulmonary Bypass
Clinical trials have recently sought to determine the impact of a goal-directed oxygen delivery while on cardiopulmonary bypass on cardiac surgery–associated AKI. The Goal-Directed Perfusion Trial (GIFT) evaluated maintaining oxygen delivery greater than 280 ml·min –1 ·m –2 versus conventional perfusion on the rate of AKI in cardiac surgery patients. 76 In this multicenter randomized controlled trial that enrolled 350 patients from nine European institutions, patients in the intervention group had a lower incidence of AKIN stage 1 AKI while no difference in the incidence of AKIN stage 2 or 3 AKI. A similar study that randomly assigned 300 patients undergoing cardiac surgery between targeting an oxygen delivery greater than 300 ml·min –1 ·m –2 versus conventional perfusion while on cardiopulmonary bypass found a higher rate of AKI (defined by KDIGO) in the conventional perfusion group. 77 A subgroup analysis of the study found that the goal-directed oxygen delivery strategy was superior in patients with lower hematocrits and lower body surface area. The results of these two trials suggest the need to individualize oxygen delivery while on cardiopulmonary bypass rather than use conventional body surface area–derived perfusion targets.
Vasopressors
Vasoplegia is common in cardiac surgery patients. 78 Because endogenous vasopressin is thought to be reduced after cardiac surgery and because vasopressin preferentially binds receptors in the efferent arterioles to potentially increase glomerular filtration rate, vasopressin use should be considered before using norepinephrine to treat post–cardiopulmonary bypass vasoplegia. 15 The Vasopressin versus Norepinephrine in Patients with Vasoplegic Shock after Cardiac Surgery (VANCS) trial randomly assigned 300 patients who experienced post–cardiopulmonary bypass vasoplegia to receive vasopressin versus norepinephrine as a first-line agent. 79 Patients who received vasopressin as a first-line agent had a significantly lower incidence of moderate to severe AKI and a lower mortality.
Perioperative Hypotension
Cardiac surgery is associated with potentially turbulent hemodynamics related to anesthetics, surgical manipulation of the heart and great vessels, comorbidities including cardiovascular and coronary artery disease, nonpulsatile flow on cardiopulmonary bypass, anemia, vasoplegia, and pump failure. Systemic hypotension due to any of these etiologies for a prolonged period can lead to impaired renal perfusion, renal tubular ischemia, and a consequent decrease in glomerular filtration rate. While evidence of an association between intraoperative hypotension and AKI has been established in the noncardiac surgery population, 80 there is controversy regarding this association in cardiac surgery patients. Several studies have attempted to evaluate the impact of intraoperative hypotension on cardiac surgery–associated AKI. One observational study of 6,523 patients reported an association between a MAP less than 65 for 10 min or more after cardiopulmonary bypass and the need for new postoperative renal replacement therapy. 81 Another study of 4,984 patients found an association between each 10-min period of hypotension throughout the cardiac surgical period and a composite outcome of stroke, AKI, or death after cardiac surgery. 82 Two randomized controlled trials 83 , 84 found that targeting higher MAPs on cardiopulmonary bypass did not reduce the incidence of cardiac surgery–associated AKI, while another clinical trial 85 showed that, in the higher MAP group, there was a significantly larger number of patients who doubled their postoperative creatinine. These findings suggest the need for further investigation on the role of intraoperative blood pressure optimization as a modality to avoid cardiac surgery–associated AKI.
Anemia and Transfusion
Both anemia 86 , 87 and blood transfusion 88 have been linked to cardiac surgery–associated AKI in multiple cohort studies. However, a large retrospective study found that combined exposure to anemia and blood transfusion increased the risk of post–cardiac surgery AKI more than either exposure alone. 89 The Transfusion Requirements in Cardiac Surgery (TRICS) III study, a multicenter randomized controlled trial, found no difference in the incidence of new-onset AKI requiring renal replacement therapy in patients who were transfused to a restrictive (7.5 g/dL) versus a liberal transfusion (9.5 g/dL) threshold. 90 A prespecified substudy of this trial also found no difference in the incidence of milder forms of AKI between patients randomly assigned to restrictive versus liberal transfusion thresholds. 91 Avoiding anemia and transfusion during the cardiac surgery perioperative period may necessitate multimodal preoperative anemia management with oral iron therapy, erythropoietin administration in patients with iron deficiency anemia, and supplementation with vitamin B 12 and folate for B 12 and folate deficiency anemia, respectively. 92
Hemolysis occurs frequently in cardiac surgery because of the shear stress applied on the red blood cells, which is invoked by blood flowing through the cardiopulmonary bypass circuit and the use of venting and suction devices. Erythrocyte washing and the transfusion of cell-salvaged blood are additional sources of hemolysis products during cardiac surgery. 93 , 94 Injured erythrocytes release cell-free hemoglobin and other heme-derived products, which normally bind to the hemoglobin and heme scavengers, haptoglobin and hemopexin, respectively, and then cleared by splenic and liver macrophages. 95 However, if these scavenging systems are overwhelmed during prolonged or excessive hemolysis, plasma levels of cell-free hemoglobin and heme increase, and their clearance relies on renal filtration. Renal cell exposure to cell-free hemoglobin and other hemolysis products results in increased oxidative stress, inflammation, and reduced bioavailability of NO in the kidneys, all of which may exacerbate renal ischemia-reperfusion injury and endothelial dysfunction, thus promoting tubular renal cell death. 96–100
Observational studies in patients undergoing cardiac surgery with cardiopulmonary bypass report that elevated levels of cell-free hemoglobin, as well as low levels of its scavenger, haptoglobin, are associated with increased cardiac surgery–associated AKI and increased mortality. 101–106 Furthermore, it has been demonstrated that elevated levels of catalytic iron, another by-product of hemolysis, are associated with increased AKI and mortality, both after cardiac surgery and in other critically ill patient groups. 107 , 108
Removal of Hemolysis Products
Extracorporeal cytokine removal to decrease the cardiopulmonary bypass–related inflammatory response via hemoadsorption has been attempted for some time. The technology is based on highly porous, biocompatible nonpolar polymer beads that bind and remove inflammatory mediators through size exclusion and nonspecific surface adsorption. The technology of polymer bead-based cytokine hemoadsorption has shown to rapidly eliminate cytokines in vitro and in vivo . 109 , 110 The Cytosorb hemoadsorption filter device (Cytosorbents Corporation, Princeton, New Jersey) has been designed to directly capture and reduce mid molecular weight inflammatory mediators (approximately 10 to 60 kDa). The substances are adsorbed due to physiochemical binding depending on their concentration. Several studies have examined the ability of the Cytosorb filter to remove cell-free hemoglobin and other hemolysis products during cardiopulmonary bypass, but the results were not promising, showing a very limited ability to remove cell-free hemoglobin. 111 , 112 Furthermore, even if effective, it is important to remember that the use of hemoadsorption requires patients to be on extracorporeal circulation such as during cardiopulmonary bypass, extracorporeal membrane oxygenation, or renal replacement therapy. 113
KDIGO “Bundle of Care”
The KDIGO Clinical Practice Guidelines includes recommendations for the prevention and treatment of AKI. 114 This section discusses the implementation of these recommendations in cardiac surgery as a “Bundle of Care.”
Functional Hemodynamic Monitoring
Cardiac output and stroke volume monitoring throughout the intraoperative and postoperative period is necessary to evaluate and optimize kidney perfusion. The PrevAKI single-center trial evaluated the impact of adherence to a bundle of interventions recommended by KDIGO to reduce AKI in high-risk patients undergoing cardiac surgery and reported a significant reduction in all stages of AKI in the KDIGO bundled care group as compared with the control group of routine care delivered in the post–cardiac surgery ICU. 115 Patients in the intervention group underwent monitoring with a Pulse index Continuous Cardiac Output monitor that provided stroke volume variation and cardiac index to guide the administration of fluids and inotropes, respectively. The multicenter PrevAKI trial, conducted by the same group of investigators, also utilized functional hemodynamic monitoring and optimized MAP and cardiac index in their intervention group. 116
Hemodynamic Optimization
Perioperative strategies for preventing cardiac surgery–associated AKI aim to optimize kidney perfusion—primarily by maintaining blood pressure and cardiac output within physiologic limits. The multicenter PrevAKI study reported that cardiac surgery patients at high risk for AKI who were randomly assigned to receive the KDIGO bundle of interventions had a significantly lower incidence of post–cardiac surgery stage 2 or stage 3 AKI (14% vs . 24%). However, there were no significant between-group differences in the multicenter study when stage 1 AKI was also assessed. This bundle included an algorithmic approach to hemodynamic optimization to maintain MAP > 65 and cardiac index > 2.5 l·min –1 ·m –2 , resulting in intervention group patients receiving significantly more fluids and being more likely to receive dobutamine. 116
Perioperative Medications
Changes in autoregulation due to comorbidities and medications such as angiotensin-converting enzyme inhibitors and angiotensin receptor blockers may potentiate renal hypoperfusion even when the systemic blood pressure is within the normal range. Patients with chronic hypertension, for example, have their autoregulatory curves shifted rightward, resulting in higher MAP requirement to ensure adequate renal perfusion. Both the single-center and multicenter PrevAKI trials discontinued angiotensin-converting enzyme inhibitors and angiotensin receptor blockers during the first 48 h after surgery as part of the KDIGO “bundle of care.” 115 , 116 However, a systematic review of six studies (three randomized controlled trials and three prospective cohort studies) evaluating the discontinuation of these agents before coronary angiography and cardiac surgery found no evidence that drug cessation impacted the incidence of AKI, citing a low quality of Grading of Recommendations, Assessment, Development and Evaluation (GRADE) evidence. 117
Dobutamine has been shown to reduce cardiac surgery–associated AKI when implemented as part of the KDIGO “bundle of care” in both the single-center and the multistudy Prev-AKI studies, suggesting that it should be used as part of a goal-directed strategy to maintain kidney perfusion in the postoperative ICU. Significantly more patients in the goal-directed management groups received dobutamine in both the single-center (31% vs . 9%) and multicenter (32.6% vs. 18.8%) trials, resulting in higher MAPs at various time points. 115 , 116
Intravenous Fluids
Compared to 0.9% saline, balanced crystalloid solutions such as Ringer’s lactate have been associated with a lower risk of AKI in critically ill patients, although the effect size was low in one meta-analysis of six studies (relative risk of 0.96). 118 This may be due to the effect of chloride-mediated vasoconstrictions of the kidneys as well as the detrimental effect of chloride-associated metabolic acidosis. 119 The Acute Disease Quality Initiative international consensus guidelines recommend resuscitation with a balanced crystalloid solution rather than 0.9% saline. 116 A meta-analysis evaluating the role of urinary alkalinization with sodium bicarbonate to prevent cardiac surgery–associated AKI found no benefit in the general cardiac surgery population. 117 However, there was a significantly lower incidence of renal replacement therapy in patients undergoing elective CABG surgery. Further studies are needed before the routine use of sodium bicarbonate can be recommended. The Albumin in Cardiac Surgery trial randomly assigned cardiac surgery patients to receive Ringer’s acetate solution or 4% albumin both in the cardiopulmonary bypass prime and used for intraoperative fluid resuscitation and found no significant difference in the incidence of AKI between the two groups. 120 However, the higher incidence of bleeding and infection seen in patients who received albumin may limit its use in cardiac surgery. Hydroxyethyl starch should be avoided throughout the perioperative period because of their association with AKI in critically ill patients, 121 including cardiac surgery patients. 122
Renal Replacement Therapy
The indications, clinical timing of initiation, and different modalities of renal replacement therapy have not been specifically studied in the context of cardiac surgery–associated AKI. In the general critically ill patient population, indications of renal replacement therapy are focused on solute control ( e.g. , electrolyte or acid-base imbalances) and volume control to restore euvolemia when patients are no longer diuretic-responsive. 123 , 124 For timing, data from recently completed clinical trials suggest that “early” initiation of renal replacement therapy in the absence of emergent indications of solute/volume control does not significantly improve mortality outcomes and is associated with more episodes of hypotension and hypophosphatemia. 125 Further, in secondary outcome analysis of the Standard versus Accelerated Initiation of Renal Replacement Therapy in Acute Kidney Injury (STARRT-AKI) trial, survivors of AKI-renal replacement therapy had higher risk of being renal replacement therapy-dependent at 90 days when compared to patients randomly assigned to the “delayed” renal replacement therapy initiation strategy. 126 Therefore, the current consensus recommendation is that renal replacement therapy should be provided for specific solute or volume control indications that are refractory to medical management, which applies to patients with cardiac surgery–associated AKI. In this context, it is critical to carefully monitor the perioperative clinical trajectory of patients at risk for or with cardiac surgery–associated AKI to evaluate the need of renal replacement therapy. 127 When renal replacement therapy is indicated, both intermittent and continuous renal replacement therapy are viable options for patients with cardiac surgery–associated AKI. Typically, renal replacement therapy is reserved for hemodynamically unstable patients given its lower net ultrafiltration rate, lower rate of osmotic shift, and slower change in extracellular fluid electrolyte concentrations with less impact on resting membrane potentials when compared to intermittent hemodialysis. 123 , 124
Urine Oximetry
Noninvasive urine oximetry is a novel concept for intraoperative and postoperative ICU use in cardiac surgery patients to assess the adequacy of kidney perfusion and oxygen delivery. This can be potentially done by continuously measuring urine oxygen partial pressure. When measured from urine as it is excreted, urine oxygen partial pressure is thought to approximate the partial pressure of oxygen in the renal medulla. 128 For this reason, urine oxygen partial pressure measurement is being investigated in cardiac surgery patients as a real-time monitor of oxygen delivery to the kidneys. Zhu et al . 129 used fiberoptic probes attached to the tip of urinary catheters to measure the partial pressure of urine in the bladders of patients undergoing cardiac surgery. The authors reported that low urine oxygen partial pressure predicted postoperative AKI and both the duration of low partial pressure as well as the nadir value increased the odds of AKI. Another study that measured urine oxygen partial pressure using a prototype device placed between the urinary catheter and collection bag in cardiac surgery found that mean oxygen partial pressure in the post–cardiopulmonary bypass period was associated with postoperative AKI. 130 These studies suggest that future studies and technical modifications may ultimately result in clinically reliable real-time monitoring of kidney oxygen delivery, allowing early interventions to mitigate risk of cardiac surgery–associated AKI.
Haptoglobin Administration
Animal studies in models of hemolysis-associated AKI have shown that administration of haptoglobin prevented circulating free hemoglobin-induced kidney injury. 131–133 In humans, a propensity-matched retrospective study reported that intraoperative administration of human haptoglobin (to bind and remove excessive cell-free hemoglobin) to patients exhibiting macrohemoglobinuria during cardiopulmonary bypass, was associated with a reduced incidence of cardiac surgery–associated AKI. 134 Because haptoglobin is the natural scavenger of hemoglobin in the body, this approach seems clinically promising; however, human haptoglobin is only available for use in Japan and is not available for clinical use in other countries.
Nitric Oxide
One of the consequences of intravascular hemolysis is depletion in plasma NO, 135 , 136 which may result in vasoconstriction and impaired tissue perfusion. 106 Cell-free hemoglobin is released in the form of ferrous oxyhemoglobin and quickly reacts with plasma NO to form ferric methemoglobin, hence reducing the level of plasma NO. 137 A second process contributing to reduced NO bioavailability during hemolysis is the increase in the level of the enzyme arginase-1, which is released from hemolyzed erythrocytes. 138 This enzyme metabolizes l- arginine, the main precursor of NO, therefore decreasing the available substrate for NO production. 137 Finally, injured erythrocytes also release asymmetric dimethylarginine, which directly inhibits endothelial NO synthase. 139 Several studies have explored the effects of intraoperative administration of NO on postoperative outcomes in cardiac surgery patients. In a prospective study of 244 adult patients undergoing multiple valve surgeries, Lei et al . 100 demonstrated that administration of NO (intraoperatively during cardiopulmonary bypass [80 ppm via the cardiopulmonary bypass circuit] and postoperatively for 24 h) compared to controls who received nitrogen, was associated with a significant reduction in cardiac surgery–associated AKI and major adverse kidney events up to 1 yr after surgery. Similarly, Kamenshchikov et al ., 140 reported that intraoperative administration of NO (40 ppm via the cardiopulmonary bypass circuit) was associated with a significant decrease in the incidence of cardiac surgery–associated AKI. Interestingly, there was no difference in the concentration of cell-free hemoglobin between treated patients and the controls in the latter study. Please note that using NO to prevent AKI is still investigational and not an approved indication.
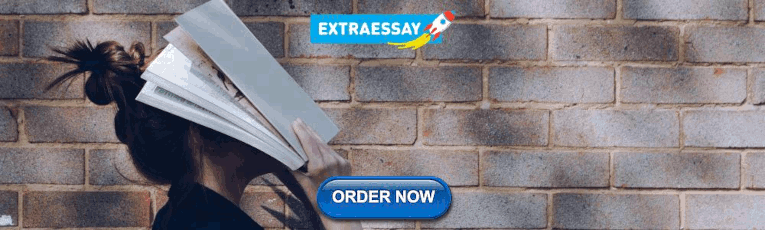
Acetaminophen
Acetaminophen has anti-inflammatory and antioxidative properties, specifically reducing the oxidation of cell-free hemoglobin by preventing the conversion of iron from the ferric ion to its more inflammatory and nephrotoxic ferryl ion form. 141 , 142 In an animal model of rhabdomyolysis-induced kidney injury, acetaminophen significantly decreased markers of lipid peroxidation and preserved kidney function. 142 Several observational retrospective studies have reported that exposure to acetaminophen was associated with decreased incidence of cardiac surgery–associated AKI in both adults and children undergoing cardiac surgery with cardiopulmonary bypass. 143 , 144 Furthermore, Janz et al . 145 reported a phase IIa randomized placebo-controlled trial of acetaminophen in patients with severe sepsis and elevated circulating cell-free hemoglobin. Patients who received acetaminophen had lower levels of markers of lipid peroxidation as well as lower serum creatinine. In addition, two small clinical trials in adults and children undergoing cardiac surgery demonstrated that acetaminophen decreased markers of lipid peroxidation in the plasma after cardiopulmonary bypass. Although no differences in the incidence of AKI between the acetaminophen and placebo groups were reported, those studies were limited by small sample sizes and low event rates. 146 , 147 Taken together, it seems that acetaminophen may have a kidney-protective effect in cardiac surgery; however, further studies, including appropriately powered prospective trials, are needed to fully understand the role of acetaminophen as a kidney-protective medication.
Published guidelines by the Society of Thoracic Surgeons, Society of Cardiovascular Anesthesiologists, American Society for Extracorporeal Technology, 15 and a practice update by the Society of Cardiovascular Anesthesiologists AKI Working group 16 summarize the available literature and provide recommendations for the management of cardiac surgery–associated AKI. The Society of Thoracic Surgeons, Society of Cardiovascular Anesthesiologists, American Society for Extracorporeal Technology guidelines graded the available evidence using the American College of Cardiology and American Heart Association Recommendation System. The Society of Cardiovascular Anesthesiologists practice update assessed published randomized controlled trials using the GRADE methodology.
The Society of Thoracic Surgeons, Society of Cardiovascular Anesthesiologists, American Society for Extracorporeal Technology guidelines recommended avoidance of hyperthermic perfusion on cardiopulmonary bypass and recommended goal-directed oxygen delivery on cardiopulmonary bypass as a Class I recommendations-evidence of benefit 15 to prevent cardiac surgery–associated AKI. Adopting the KDIGO “bundle of care” for high-risk postoperative patients is also recommended to reduce the risk of cardiac surgery–associated AKI (Class IIa recommendation: should be considered). The use of minimally invasive extracorporeal circulation techniques was recommended as a Class IIb recommendation: may be considered. The guidelines recommend the use of fenoldopam in patients who tolerate the drug without hypotension (Class IIb recommendation: may be considered), but use of this medication has not been widely accepted in clinical settings. The guidelines recommend against the perioperative use of dopamine and mannitol, citing evidence that neither drug has shown to protect against the development of cardiac surgery–associated AKI (Class III recommendation: not recommended).
The Society of Cardiovascular Anesthesiologists Practice Update endorses the use of goal-directed oxygen delivery and the KDIGO “bundle of care” in high-risk patients, citing moderate level of GRADE evidence. 16 This bundle, described in greater detail previously, includes volume and hemodynamic optimization, and avoidance of nephrotoxins and hypoglycemia. They also recommend the use of vasopressin over norepinephrine for treatment of vasoplegic shock after cardiac surgery, citing a low level of GRADE evidence. The practice update also reported that targeting higher MAP goals during cardiopulmonary bypass, and the perioperative use of dopamine and dexmedetomidine did not reduce cardiac surgery–associated AKI, citing a low level of GRADE evidence.
Acute kidney injury is common after cardiac surgery and is associated with worse outcomes than patients who do not develop post–cardiac surgery AKI. Although the pathogenesis of cardiac surgery–associated AKI is complex and multifactorial, the common consequence is renal tubular injury with a decline in glomerular filtration rate. The risk factors for cardiac surgery–associated AKI have been well characterized and used to develop predictive models. These models have performed well in predicting the need for renal replacement therapy but have less discriminatory ability to predict milder forms of AKI that are also associated with worse outcomes. Novel biomarkers of kidney function, inflammation, and injury may allow earlier detection of cardiac surgery–associated AKI, better prediction models for developing cardiac surgery–associated AKI and to enable clinical trialists to study potential preventive and treatment strategies in the patients who are truly at risk for developing cardiac surgery–associated AKI. Preventing AKI requires the identification of patients with modifiable risk factors and mitigating this risk using evidence-based interventions throughout the perioperative period. Discontinuing nephrotoxic medications, optimizing chronic medication conditions, and treating anemia are recommended before elective cardiac surgery. During the intraoperative and postoperative phases, utilizing balanced crystalloids for resuscitation and using a goal-directed hemodynamic strategy to optimize cardiac output and kidney perfusion pressure have been shown to improve kidney outcomes. Research-driven advances in monitoring, real-time data capture, and subphenotyping analyses, as well as discovery of novel detection and prediction biomarkers of kidney function and injury may ultimately allow real-time detection of kidney injury in the operating room or in the early postoperative period.
Research Support
This work was supported by National Institutes of Health, National Heart, Lung, and Blood Institute (Bethesda, Maryland) grant R01HL148448 (to Dr. Fox) and National Institute of Diabetes and Digestive and Kidney Diseases (Bethesda, Maryland) grants R01DK128208, R01DK133539, U01DK129989, and P30 DK079337 (to Dr. Neyra).
Competing Interests
Dr. Raphael has consulted for Octapharma USA, Inc. (Paramus, New Jersey). Dr. Neyra has received consulting honoraria from Baxter (Deerfield, Illinois), Outset (San Jose, California), Vifor (Zurich, Switzerland), and AcelRx (San Mateo, California). The other authors declare no competing interests.
Citing articles via
Most viewed, email alerts, related articles, social media, affiliations.
- ASA Practice Parameters
- Online First
- Author Resource Center
- About the Journal
- Editorial Board
- Rights & Permissions
- Online ISSN 1528-1175
- Print ISSN 0003-3022
- Anesthesiology
- ASA Monitor

- Terms & Conditions Privacy Policy
- Manage Cookie Preferences
- © Copyright 2024 American Society of Anesthesiologists
This Feature Is Available To Subscribers Only
Sign In or Create an Account
Thank you for visiting nature.com. You are using a browser version with limited support for CSS. To obtain the best experience, we recommend you use a more up to date browser (or turn off compatibility mode in Internet Explorer). In the meantime, to ensure continued support, we are displaying the site without styles and JavaScript.
- View all journals
Acute kidney injury articles from across Nature Portfolio
Acute kidney injury (AKI) is a rapid deterioration in kidney function that occurs within 48 hours of an initiating event and is associated with an absolute increase in serum creatinine of ≥26.4 μmol/l. Main causes of AKI include sepsis, ischaemia-reperfusion injury and nephrotoxins. Symptoms include fatigue, loss of appetite, headache, nausea and vomiting.
Latest Research and Reviews
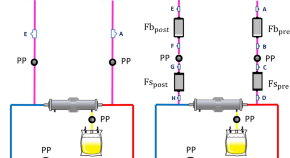
Spallation and particles infusion into the extracorporeal circuit during CRRT: a preventable phenomenon
- Maria Cristina Ruffa
- Giacomo Bignante
- Gianluca Villa
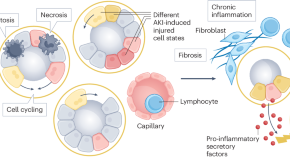
Epithelial cell states associated with kidney and allograft injury
This Review describes parallels in the injury mechanisms that underlie acute kidney injury, chronic kidney disease and allograft injury, and explains how our understanding of the molecular changes that occur in epithelia in the context of kidney disease may contribute to the therapeutic targeting of specific epithelial cell phenotypes for the treatment of transplantation complications.
- Christian Hinze
- Svjetlana Lovric
- Kai M. Schmidt-Ott
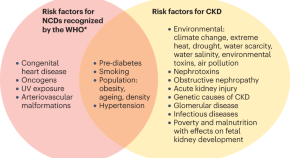
Chronic kidney disease and the global public health agenda: an international consensus
Addressing the burden of non-communicable diseases is a global public health priority. In this joint Consensus Statement, the American Society of Nephrology, the European Renal Association and the International Society of Nephrology highlight the need to recognize kidney disease as a key driver of premature mortality, in addition to other non-communicable diseases already prioritized by the World Health Organization.
- Anna Francis
- Meera N. Harhay
- Vivekanand Jha
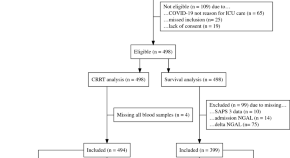
Plasma neutrophil gelatinase-associated lipocalin independently predicts dialysis need and mortality in critical COVID-19
- Jonas Engström
- Hazem Koozi
- Martin Spångfors
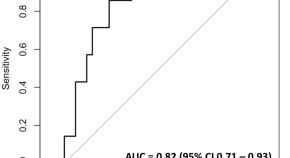
Development of a predictive model for risk stratification of acute kidney injury in patients undergoing cytoreductive surgery with hyperthermic intraperitoneal chemotherapy
- Martin Krause
- Soraya Mehdipour
- Rodney A. Gabriel
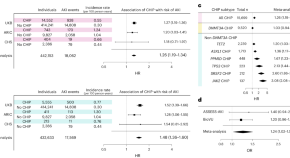
Clonal hematopoiesis of indeterminate potential is associated with acute kidney injury
Clonal hematopoiesis, which increases with age and is implicated in a variety of age-related diseases, is shown here to be associated with a greater risk of acute kidney injury and worse outcome following injury, as demonstrated using multiple patient cohorts, Mendelian randomization analysis and mechanistic studies in mouse disease models.
- Caitlyn Vlasschaert
- Cassianne Robinson-Cohen
- Alexander G. Bick
News and Comment
Clonal haematopoiesis and aki.
- Susan J. Allison
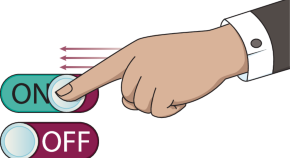
Switching off SOX9 for epithelial recovery after AKI
- Monica Wang
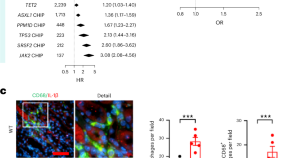
Acquired blood mutations cause acute kidney injury via dysregulated inflammation
Acute kidney injury affects one in five hospitalized patients and can lead to lasting kidney damage or death. We show that clonal hematopoiesis of indeterminate potential — a common age-related condition caused by blood cell mutations — increases the risk of acute kidney injury in multiple cohorts of human patients and in mouse models.
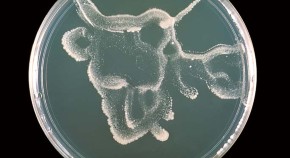
Targeting IL-1Ra to attenuate fungal infection
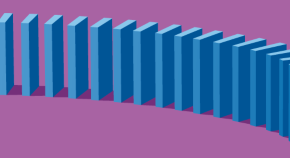
Propagation of acute kidney injury
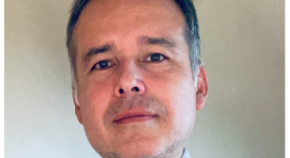
Reducing preventable acute kidney injury in a hospital setting
Acute kidney injury (AKI) is a global concern that affects patient outcomes and resource utilization. An effective educational programme aimed at improving early AKI identification, triaging and treatment, using risk scores and care bundles coupled with access to point-of-care diagnostics, can help to prevent AKI-related deaths in regions with limited resources.
- Rolando Claure-Del Granado
Quick links
- Explore articles by subject
- Guide to authors
- Editorial policies

Advertisement
Management of Acute Kidney Injury in Critically Ill Children
- Review Article
- Published: 02 March 2023
- Volume 90 , pages 481–491, ( 2023 )
Cite this article
- Sudarsan Krishnasamy 1 ,
- Aditi Sinha ORCID: orcid.org/0000-0002-9566-3370 2 &
- Arvind Bagga 2
4632 Accesses
2 Citations
3 Altmetric
Explore all metrics
Acute kidney injury (AKI) is common in critically ill patients, affecting almost one in four critically ill children and one in three neonates. Higher stages of AKI portend worse outcomes. Identifying AKI timely and instituting appropriate measures to prevent and manage severe AKI is important, since it is independently associated with mortality. Methods to predict severe AKI should be applied to all critically ill patients. Assessment of volume status to prevent the development of fluid overload is useful to prevent adverse outcomes. Patients with metabolic or clinical complications of AKI need prompt kidney replacement therapy (KRT). Various modes of KRT are available, and the choice of modality depends most on the technical competence of the center, patient size, and hemodynamic stability. Given the significant risk of chronic kidney disease, patients with AKI require long-term follow-up. It is important to focus on improving awareness about AKI, incorporate AKI prevention as a quality initiative, and improve detection, prevention, and management of AKI with the aim of reducing acute and long-term morbidity and mortality.
Similar content being viewed by others
Evaluation and Management of Acute Kidney Injury in Children
Avoid common mistakes on your manuscript.
Introduction
Acute kidney injury (AKI) is a clinical syndrome characterized by an abrupt decrease in kidney function, resulting in the accumulation of waste products and critical imbalances in fluid and electrolyte homeostasis. The term ‘AKI’ replaced ‘acute renal failure’ to encompass kidney dysfunction ranging from mildly elevated serum creatinine to severe forms requiring kidney replacement therapy (KRT) [ 1 ], emphasizing that even small increments in creatinine are associated with adverse short- and long-term outcomes.
Definitions and Staging
AKI has conventionally been defined and staged for severity based on serum creatinine and urine output (Table 1 ). The KDIGO criteria, which harmonize the AKIN with the RIFLE criteria, are most commonly used in adults and children [ 2 ]. The pediatric reference change value optimized criterion for AKI (pROCK), which is based on paired creatinine values in Chinese children admitted with nonrenal morbidities [ 3 ], might be more specific than KDIGO criteria in detecting ‘true’ AKI, but requires validation.
Neonatal AKI is more difficult to identify. Serum creatinine at birth reflects maternal serum levels. Levels decline in the first few weeks, making ‘baseline’ creatinine dynamic. While sensitive, urine output is difficult to measure and is often preserved despite AKI due to tubular immaturity. The RIFLE and KDIGO definitions were adapted for neonates but require validation (Supplementary Table S1 ) . In practice, an increase in creatinine by ≥ 0.3 mg/dL detects AKI better than a 50% rise in creatinine. A peak creatinine value of ≥ 2.5 mg/dL (~eGFR < 10 mL/min/1.73 m 2 ) indicates severe AKI, and values ≥ 0.5 mg/dL at discharge are of concern [ 4 ].
Epidemiology
In a prospective report, AKI affected ~5% of hospitalized patients and ~30% of patients in the pediatric intensive care unit (PICU) [ 5 ]. Three multicenter studies inform on the global epidemiology of pediatric AKI. Using KDIGO criteria, the Assessment of Worldwide Acute Kidney Injury, Renal Angina, and Epidemiology (AWARE) study reported AKI in 26.9% of critically ill patients [ 6 ]. The Assessment of Worldwide Acute Kidney Injury Epidemiology in Neonates (AWAKEN) study reported AKI in 29.9% newborns, most commonly in those born at < 29 wk of gestation [ 7 ]. In the 0by25 Global Snapshot study, similar proportions of hospitalized patients had community-acquired and incident AKI [ 8 ]. Patients with AKI in low- and middle-income countries were older than their counterparts in high-income countries, usually had community-acquired AKI, and commonly had severe AKI [ 8 ].
Etiology and Risk Factors
Based on pathophysiology, AKI is categorized as prerenal or functional, renal or intrinsic, and postrenal or obstructive. The Acute Dialysis Quality Initiative network summarized the risk factors into environmental, socioeconomic/cultural, process of care, acute exposures, and inherent factors [ 9 ]. Risk factors, etiology, and outcomes of AKI differ between resource-limited and resource-sufficient regions and for community-acquired and hospital-acquired AKI. AKI in developing countries is often community-acquired, caused by diarrheal dehydration, infections (sepsis, malaria, dengue, scrub typhus, leptospirosis), hemolytic uremic syndrome, glomerulonephritis, snake envenomation, and toxic medications [ 5 , 10 , 11 ]. In contrast, hospital-acquired AKI is more common in the developed world and is often associated with major surgery, septicemia, and/or multiorgan failure [ 11 ].
AKI in neonates is most often prerenal and is caused by systemic hypoperfusion due to placental hypoperfusion, birth asphyxia, insensible losses (e.g., preterm neonates on phototherapy), diarrheal dehydration, third-space losses (sepsis, necrotizing enterocolitis), congenital heart disease, post-cardiac surgery, and following nephrotoxic therapies [ 7 ].
Investigations in critically ill patients with AKI are directed at identifying the etiology of AKI and screening for complications (Table 2 ). Urinary indices to discriminate functional from intrinsic AKI, e.g., urine sodium (</≥ 20 mEq/L), fractional excretion of sodium (< 1%/≥ 1%), fractional excretion of urea (< 35%/> 50%), osmolality (> 400/< 350 mOsm/kg) and specific gravity (> 1020/< 1012), have limited utility in patients receiving diuretics and/or IV fluids. A kidney biopsy is indicated when suspecting glomerulonephritis or interstitial nephritis with unexplained AKI and allograft dysfunction. Various tools have been used to identify critically ill patients without AKI or with mild AKI who are at risk of severe AKI, the need for KRT, and mortality (Table 3 ).
Fluid Overload
Fluid overload may not just indicate but also lead to AKI by affecting multiple organs, e.g., increased intra-abdominal pressure compromises gas exchange, myocardial performance, intestinal function, and renal perfusion, and delayed wound healing promotes inflammation. Meta-analyses of observational studies indicate that the degree of fluid overload correlates with mortality, with a 19% increase in risk for every liter of fluid overload (34 studies, 31,076 adults) and 6% for every percentage point increment (44 studies, 7507 children) [ 12 , 13 ]. Fluid overload was associated with an increased risk of mechanical ventilation and AKI in critically ill children [ 13 ] and neonates (17 studies, 4772 neonates) [ 14 ]. While a threshold of > 10% defines ‘clinically significant’ fluid overload that merits KRT, lower values may also impact outcomes.
Renal Angina Index
The renal angina index (RAI), a product of the risk and injury scores assessed 8–12 h after ICU admission, has emerged as a satisfactory bedside tool to predict the occurrence of severe AKI in patients admitted to PICU (Table 3 ). A meta-analysis (22 studies, 14,001 children) found that RAI > 8 predicts day 3 severe AKI with 86% sensitivity and 77% specificity (AUC 0.88), and the need for KRT with 82% sensitivity and 74% specificity (AUC 0.85) [ 15 ].
Furosemide Stress Test (FST)
In early AKI, the response to furosemide might indicate tubular integrity. It was first described in critically ill adults, in whom a urine output of < 200 mL over 2 h following 1 mg/kg IV furosemide satisfactorily predicted stage-3 AKI (87.1% sensitivity, 84.1% specificity, and AUC 0.87) [ 16 ]. Subsequent studies confirm that FST outperforms conventional biomarkers in predicting progression to stage-3 AKI, the need for KRT, and mortality, allowing decisions on postponing KRT until conventionally indicated [ 17 ]. A meta-analysis (11 studies, 1366 critically ill adults) found that FST predicts AKI progression and the need for KRT with respective sensitivity and specificity of 81% vs. 84% and 88% vs. 77% [ 18 ]. However, FST is not validated in children. In three retrospective studies, urine output following IV furosemide predicted severe AKI in infants after cardiac surgery [ 19 , 20 , 21 ]. In a prospective study on 51 critically ill children with AKI stages 1–2, using a threshold of > 2 mL/kg urine over 2 h after furosemide administration, FST outperformed plasma NGAL and proenkephalin in predicting severe AKI and the need for KRT [ 22 ]. Larger prospective pediatric studies are necessary before FST is applied bedside.
Despite decades of research, a troponin-like diagnostic and predictive biomarker for pediatric AKI remains elusive. NGAL remains the most widely tested biomarker in children. In a meta-analysis of 56 studies investigating 49 biomarkers in 8617 children post-cardiac surgery, the urine NGAL to creatinine ratio had the highest diagnostic odds ratio (91%) with 91.3% sensitivity and 89.7% specificity [ 23 ], and combining it with RAI improved the diagnostic accuracy for severe AKI [ 15 ].
Dynamic Risk Prediction and Combination Tools
Dynamic models of AKI risk prediction that improved AKI recognition based on electronic inpatient records in individual studies include alerts for nephrotoxin exposure (e.g., NINJA), AKI care bundles [ 24 ], the four-pronged FOKIS (correlates with mortality; Table 3 ) [ 25 ], and a neonatal risk score (STARZ; predicts AKI with 82% sensitivity and 91.7% specificity) [ 26 ]. Experts recommend that clinical assessment and investigations be combined with biochemical and functional biomarkers to stratify the risk of severe AKI and inform decisions on its prevention and management [ 27 ].
This section discusses important concerns in the nondialytic management of AKI, excluding the management of complications and specific etiologies of AKI.
Fluid Management
Assessment of volume status is critical in all patients with AKI. While hypovolemia, e.g., following diarrheal dehydration, is detrimental, fluid overload is associated with adverse outcomes. The quantity, rate, and type of fluids to be used in critically ill patients is debated. Since hyperchloremia is associated with tubular injury, delayed recovery of kidney function, and increased mortality, balanced/buffered crystalloids with lower chloride concentrations have been preferred, although evidence is awaited [ 28 ]. The use of hydroxylethyl starch or IV albumin is not recommended [ 28 ]. Fluid stewardship, following ‘ROSE’ (resuscitation, optimization, stabilization, and evaluation/deresuscitation) for fluid therapy in septic shock, and attention to the four D’s (drug, duration, dosing, and de-escalation) is suggested [ 29 ].
Diuretics and Adjunctive Therapies
A meta-analysis (28 trials, 3228 adults with or at risk for AKI) showed that intermittent IV furosemide did not influence rates of AKI, need for KRT, or death [ 30 ]. Continuous infusion of low-dose furosemide in early AKI did not alter the progression of AKI or to KRT in adults or children [ 31 , 32 ]. In the absence of clear benefit, diuretics should not be used to prevent and/or treat AKI, and KRT should be initiated when indicated [ 33 ].
There is insufficient evidence to support the use of mannitol, dopamine, fenoldopam, atrial natriuretic peptide, nesiritide, or rasburicase in preventing AKI or the need for KRT [ 33 ]. The only evidence-based intervention to prevent AKI is theophylline (single IV dose of 5–8 mg/kg within an hour of birth) in neonates with severe perinatal asphyxia [ 33 ].
Drug Dosing and Nephrotoxic Medications
Drug dosing is difficult in critically ill patients with AKI due to altered pharmacokinetics, reduced clearances, extracorporeal removal during KRT, and dynamic organ functions. Evidence to guide dosing for most drugs is limited, especially for children. Antibiotic dosing while on KRT follows recommendations for adults [ 34 ]. For other medications, dosing while on continuous KRT (CKRT) assumes either a clearance of ~20–50 mL/min/1.73 m 2 or is estimated from the CKRT effluent rate [ 35 ]. Where feasible, therapeutic drug monitoring should support decisions regarding dosing. Electronic alerts (‘NINJA’, ‘Baby NINJA’) following use of nephrotoxic medications (Supplementary Table S2 ) reduce the incidence of drug-induced AKI [ 36 ].
Almost one-third of critically ill patients are severely malnourished; prevailing nutritional practices meet two-thirds of energy and one-third of protein requirements, fluid restriction accentuates underfeeding, and nutrient clearances are increased in CKRT [ 37 ]. Achieving nutritional goals enterally versus parenterally was linked to improved survival, while high protein intake does not retard renal recovery [ 38 ]. According to international guidelines, enteral nutrition is preferred and should commence preferably within 2 d, targeting calories at 120%–130% of basal metabolic needs, and protein intake of 2–3 g/kg/d [ 39 ]. Frequent review of the nutritional prescription, reassessing anthropometry, and monitoring blood levels of urea nitrogen, electrolytes, glucose, and lipids help achieve the nutritional goals.
Kidney Replacement Therapy
Timing of krt.
KRT should be promptly initiated in patients with AKI presenting with features of fluid overload > 10%, pulmonary edema, uremia (urea > 200 mg/dL) with encephalopathy, persistent hyperkalemia (potassium > 6 mEq/L), metabolic acidosis (pH < 7.15), and refractory or symptomatic hyponatremia or severe hyperuricemia or hyperphosphatemia associated with tumor lysis syndrome (TLS). Similarly, initiating KRT promptly in patients with hyperammonemia/metabolic decompensation in cases of inborn errors of metabolism (e.g., propionic acidemia, methylmalonic acidemia), or poisoning and intoxications (e.g., lithium, salicylate, valproic acid, metformin) improves outcomes.
The timing of initiation of KRT, in the absence of the above-mentioned factors, is debatable. Five recent RCTs in adult patients have reported the risks and benefits of early versus late initiation of KRT, and a meta-analysis (10 trials, 2143 adults) failed to find any benefit from initiating KRT early [ 40 ]. Rates of dialysis dependence and treatment-related adverse events were higher in patients initiated on KRT early. However, waiting until KRT was indicated urgently, was associated with higher mortality in AKIKI-2. These results cannot be extrapolated to pediatric patients, in whom retrospective studies have associated delayed initiation of KRT with adverse outcomes [ 41 , 42 ]. From a practical perspective, it seems prudent to initiate KRT if oliguria and azotemia persist beyond 48–72 h, even in the absence of clinical or metabolic complications of AKI.
Modalities of KRT
The advantages and disadvantages of different modalities of KRT are summarized in Table 4 and discussed below. The choice of modality in critically ill children is guided more by patient size, hemodynamic stability, the feasibility of securing vascular access and appropriately sized dialysis catheters, and available technical expertise for extracorporeal therapies, than dialysis efficiency [ 43 ]. Hence, intermittent hemodialysis is preferred in hemodynamically stable older children, and peritoneal dialysis is favored in young infants in whom vascular access, dialyzer size, and the nonavailability of suitable machines preclude hemodialysis. In hemodynamically unstable patients, prolonged intermittent KRT is preferred over PD, which is inefficient in the presence of mesenteric ischemia.
Peritoneal Dialysis
Peritoneal dialysis (PD) is a simple and inexpensive modality of KRT that is feasible in low- resource settings, infants, and hemodynamically unstable patients. PD is relatively contraindicated in patients with recent abdominal surgery, peritonitis, or an inguinal hernia. PD is less preferred when rapid clearance is required, e.g., in severe hyperammonemia. The International Society of Peritoneal Dialysis (ISPD) recently provided optimal and minimum standards for peritoneal access, dialysate fluids, and PD prescription for children with AKI [ 44 ]. A soft (Tenckhoff) catheter, inserted surgically or bedside using the Seldinger technique, is preferred over rigid (stiff) catheters and improvisations (nasogastric tube, intercostal drain) [ 44 ]. Measures to avoid peritonitis include administering an antibiotic dose 30 min before catheter insertion, removing rigid catheters within 72 h, preferring automated cyclers over manually operated open systems, and using closed dialysate circuits for manual cycles (Y-connections in older children and ready-made closed circuits with buretrols in infants). Commercially prepared dialysates are preferred to locally prepared fluids [ 44 ]. Higher than usual dextrose concentrations (1.5%–1.7%) are used if the ultrafiltration target is not met. Bicarbonate-based solutions are preferred to lactate-containing solutions in patients with hepatic dysfunction, hemodynamic instability, and worsening metabolic acidosis. The dwell volume should be 30–40 mL/kg (800–1100 mL/m 2 ), except if initiating PD soon after surgical catheter placement, when short (10–15 mL/kg) dwells are preferred to avoid leakage. The dwell duration depends on ultrafiltration and solute removal targets; e.g., it is 20–30 min in the presence of hyperkalemia and acidosis and lengthened to 2–3 h once azotemia improves. Patients are monitored closely for dehydration, hypervolemia or sepsis, and for electrolytes, pH and glucose every 8–12 h.
Continuous-flow peritoneal dialysis is considered when conventional PD is ineffective, particularly when dwell volume is limited by post-surgery status or high ventilatory settings. The prescription comprises low dwell volumes (10–20 mL/kg, high dialysate flow (50–100 mL/min per 1.73 m 2 ), and slow ultrafiltration (2.5 mL/min per 1.73 m 2 ) [ 44 ].
Intermittent Hemodialysis
Hemodialysis enables rapid and efficient solute clearance and ultrafiltration, making it the most suited modality for hemodynamically stable patients with vascular access, particularly in urgent settings, e.g., poisoning, TLS, hyperammonemia, pulmonary edema. Therapy is intermittent (3–4 h daily), allowing downtime for diagnostic procedures. However, hemodialysis requires technical expertise, especially with pediatric patients. Hemodialysis is difficult in young children in the absence of pediatric-specific equipment and large-bore vascular access, typically a double-lumen hemodialysis catheter in the internal jugular or femoral vein; subclavian access carries the risk of stenosis.
The hemodialysis prescription is similar in AKI and CKD and aimed at maximizing solute clearance and fluid removal without causing adverse events [ 45 ]. The prescription varies by the child’s size, including dialyzer size (0.7–1 × body surface area), tubings (neonatal, pediatric, or adult lines), and rates of blood flow (4–8 mL/kg/min), dialysate flow (1.5–2 × blood flow rate), and ultrafiltration (≤ 0.2 mL/kg/min or ≤ 5% weight). The dialysate composition may require alteration for severe hypernatremia or hyperkalemia. Session length and frequency are guided by the solute and ultrafiltration targets. If the extracorporeal blood volume, estimated by the dialyzer size and tubings, exceeds 10% of the blood volume, the circuit is primed with blood, 5% albumin, or saline to avoid intradialytic hypotension. Frequent assessment of dry weight, close monitoring of vital signs, and noninvasive blood volume monitoring enable safe ultrafiltration.
Continuous Kidney Replacement Therapy
CKRT is preferred in critically ill, hemodynamically unstable patients since it permits gradual and continuous solute clearance and ultrafiltration, thus avoiding rapid solute fluxes, which carry risks of intradialytic hypotension and raised intracranial pressure, while allowing liberal use of boluses, infusions and adequate nutritional support. However, CKRT is not uniformly available across PICUs due to the requirement of expensive equipment, consumables, and technical expertise.
The principles of solute clearance and ultrafiltration in CKRT are similar to those of intermittent hemodialysis but include lower flow rates. The most commonly used modes include slow continuous ultrafiltration (SCUF), continuous venovenous hemofiltration (CVVH), continuous venovenous hemodialysis (CVVHD) and continuous venovenous hemodiafiltration (CVVHDF) (Fig. 1 ). Two modalities (CVVH and CVVHD) rely on replacement fluid to mediate predominantly convective clearance. The choice of modality is guided by therapy goals (ultrafiltration versus solute clearance), available circuits, and physician preference. While a conventional dose of KRT is a dialysis fluid rate of 2000 mL/1.73 m 2 /h, in settings such as hyperammonemia, higher doses (4000–8000 mL/1.73 m 2 /h) are preferred. Circuit priming averts the risk of hypotension with high extracorporeal volumes. Outcomes do not differ significantly among various modalities [ 46 ].
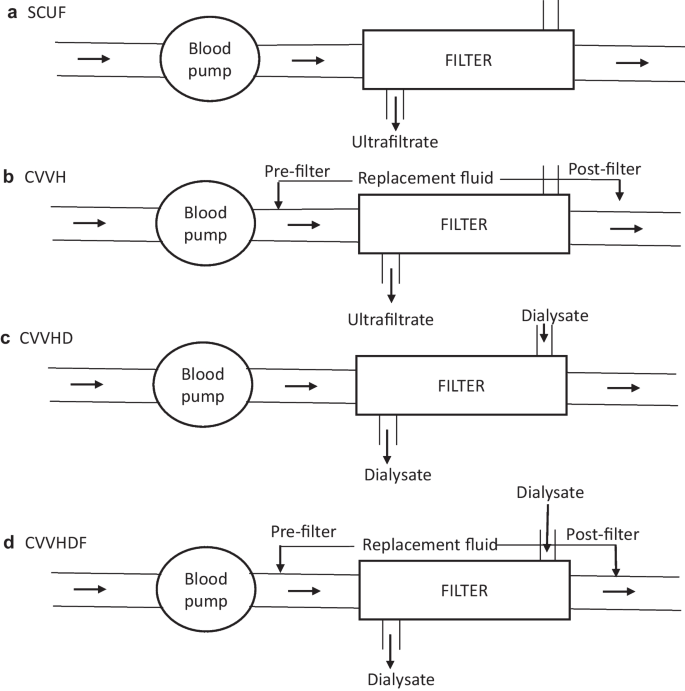
Circuit diagrams for the various modes of continuous kidney replacement therapy (CKRT). Blood flows from left to right from the patient to the blood pump and then to the filter, from which it is returned to the patient. a Slow continuous ultrafiltration (SCUF) : In this modality, there is no diffusive clearance and only ultrafiltrate (UF) is generated across the filter; this method is preferred for isolated UF removal when kidney function is normal. b Continuous venovenous hemofiltration (CVVH): Replacement fluid is run either pre- or post-filter in a volume to replace the effluent; excess effluent is removed to ensure the UF desired for negative fluid balance; clearance is convective rather than diffusive. c Continuous venovenous hemodialysis (CVVHD): Blood flows across the filter in a countercurrent fashion with the dialysate fluid, and the effluent predominantly consists of dialysate fluid with minimal, if any, UF, as in intermittent hemodialysis. d Continuous venovenous hemodiafiltration (CVVHDF) : This modality combines CVVH with CVVHD, such that the blood and dialysis fluid run in counter-current directions, the replacement fluid is either pre- or post-filter, and the effluent comprises the dialysate and replacement fluids
Prolonged Intermittent Therapies
Sustained low-efficiency dialysis (SLED) is a form of prolonged intermittent renal replacement therapy (PIRRT), combining the advantages of intermittent hemodialysis and CKRT [ 47 ]. SLED utilizes conventional hemodialysis machines, dialyzers, and tubings, but the blood and dialysate flow rates are lower (Q B : 3–5 mL/kg/min; ≤ 150 mL/min in older children; Q D : ≤ 2 times the Q B ; ≤ 300 mL/min in older children) and the sessions are longer (6–12 h). SLED achieves similar solute clearance and ultrafiltration as conventional hemodialysis without inducing hemodynamic instability in critically ill patients. The minimum dialysate rate is limited by the lack of pediatric-specific dialysis machines. Ultrafiltration goals (rate ≤ 0.2 mL/kg/min) and the need for anticoagulation and circuit priming are similar to hemodialysis. SLED may be performed daily or on alternate days at the physician’s discretion. The addition of convective clearance, as in hemodiafiltration (SLEDD-F) improves solute clearances.
Given its accessibility, lower cost, shorter session length and lesser technical complexity, SLED is increasingly preferred over CKRT in adult and pediatric critically ill patients. A meta-analysis (11 studies, 1160 critically ill adults) found that SLED and CKRT were associated with similar ultrafiltration and comparable lengths of hospital stay and rates of mortality, dialysis dependence, and renal recovery [ 48 ]. Based on experience with 203 children in three retrospective and two prospective series, SLED appears safe, effective, and feasible in critically ill pediatric patients [ 47 , 49 , 50 ].
Anticoagulation
Anticoagulation is necessary to prevent clotting in extracorporeal circuits, particularly with small catheters and low blood flow and/or high ultrafiltration rates. Anticoagulation is particularly challenging during CKRT, which requires prolonged circuit patency at slow blood flow rates. Unfractionated heparin is used most commonly and is injected into the circuit as an IV bolus, followed by its infusion or intermittent boluses [ 51 ]. In patients with coagulopathy, intermittent flushes with saline can be used to keep the circuit patent during intermittent hemodialysis and short SLED sessions; however, this limits ultrafiltration. Citrate-based protocols, allowing regional anticoagulation limited to the circuit rather than systemic, are particularly useful in patients with coagulopathy on CKRT. A meta-analysis on anticoagulation indicates that regional citrate anticoagulation provides better circuit and filter life than heparin [ 51 ]. However, careful titration is essential to prevent hypocalcemia, and dose adjustments are required in the presence of hepatic dysfunction. Less established options of anticoagulation include low molecular weight heparin, prostacyclin, direct thrombin inhibitors (argatroban), fondaparinux, and nafamostat [ 51 ].
Extracorporeal Therapy in Infants
Delivering KRT to infants is challenging since conventional hemodialysis equipment is designed for adults, with extracorporeal volumes and calibration errors that are unacceptably high for children < 17 kg body weight. Three machines, namely the Cardiorenal Pediatric Emergency Dialysis Machine (CARPEDIEM ® ), Newcastle Infant Dialysis and Ultrafiltration System (NIDUS ® ), and Aquadex ® , can perform KRT in neonates and young infants using low extracorporeal blood volume and a slow blood flow rate while permitting precise calibration of ultrafiltration. Multicenter retrospective data confirm their safety and feasibility in infants with AKI [ 52 , 53 ]. However, these machines are not marketed in India, and most centers use conventional machines, e.g., Prismaflex ® , to deliver CKRT to infants.
Specific Settings
AKI affects 36.2% (95% CI 18.8–55.8) patients with acute or acute-on chronic liver failure, chiefly as functional AKI or acute tubular necrosis following gastrointestinal bleeding, vomiting, and hepatorenal syndrome [ 54 ]. Volume expansion with fluids and albumin, loop diuretics, and vasoconstrictors (vasopressin, octreotide) are useful in hepatorenal syndrome. Beyond conventional indications, KRT is considered in the presence of hyperammonemia and hepatic encephalopathy. CKRT is preferred to intermittent hemodialysis since it permits the slow, continuous removal of ammonia, thus reducing the risk of cerebral edema [ 54 ]. Anticoagulation is avoided in the presence of coagulopathy. In the absence of conventional extracorporeal liver support (molecular adsorbent recirculating system, Prometheus, single-pass albumin dialysis) for fulminant acute liver failure, therapeutic plasma exchanges might serve as a bridge to liver transplantation [ 55 ].
During acute metabolic crises in inborn errors of metabolism, toxic metabolites like ammonia and leucine accumulate rapidly, leading to cerebral edema and neurological sequelae. CKRT has an effective modality to remove these metabolites and is initiated in patients with (i) rapidly deteriorating neurological status and blood ammonia > 150 μmol/L; (ii) moderate or severe encephalopathy; or (iii) persistently high ammonia levels > 400 μmol/L; (iv) rapid rise to > 300 μmol/L, despite adequate medical measures (protein restriction; nitrogen scavengers, e.g., sodium benzoate) [ 56 ]. CVVHD is recommended, with blood and dialysate flow rates of 30–50 mL/min and ≥ 2500–3000 mL/1.73 m 2 /h, respectively, and a dialysate to blood flow rate ratio of > 1.5 [ 56 ]. While intermittent hemodialysis also clears metabolites rapidly, it carries the risks of post-session rebound and intradialytic hypotension. Peritoneal dialysis is less effective and unreliable in clearing large metabolites (e.g., organic acids) [ 56 ].
AKI is an important component of the Cairo–Bishop criteria used to define and classify TLS. Risk stratification, adequate hydration, and routine and early use of allopurinol and/or rasburicase have reduced its incidence. The threshold for KRT initiation is low; chief indications include refractory hyperkalemia, hyperphosphatemia, hyperuricemia, metabolic acidosis, and fluid overload unresponsive to diuretics [ 57 ].
Mechanisms for AKI in COVID-19 include hypoxia, hypotension, inflammation associated with a cytokine storm, and exposure to nephrotoxic agents. About 10% of children hospitalized with COVID-19 developed AKI, of which the majority were mild and uncommonly merited KRT [ 58 ]. The PCRRT workgroup has outlined key considerations when managing critically ill children with COVID-19 and AKI [ 59 ].
Recent multicenter studies show that AKI in critically ill children is independently associated with prolonged ICU or hospital stay and high mortality, with stepwise increments by AKI stage [ 6 , 7 , 8 ]. Mortality is higher in developing countries, correlating inversely with per capita income and health expenditure [ 1 , 8 ]. In a systematic review of five studies on severe AKI requiring KRT in children, the cumulative incidence (95% CI) per 100 patient-years of reduced GFR (< 90 mL/min/1.73 m 2 ), proteinuria, hypertension and kidney failure were 6.3 (5.1–7.5), 3.1 (2.1–4.1), 1.4 (0.9–2.1), and 0.9 (0.6–1.4), respectively, underscoring the importance of prolonged follow up for development of CKD in patients with AKI [ 60 ].
Mehta RL, Kellum JA, Shah SV, et al. Acute kidney injury network: Report of an initiative to improve outcomes in acute kidney injury. Crit Care. 2007;11:R31.
Kidney Disease: Improving Global Outcomes (KDIGO) Acute Kidney Injury Work Group. KDIGO clinical practice guideline for acute kidney injury. Kidney Int Suppl. 2012;2:1–138.
Xu X, Nie S, Zhang A, et al. A new criterion for pediatric AKI based on the reference change value of serum creatinine. J Am Soc Nephrol. 2018;29:2432–42.
Article CAS PubMed PubMed Central Google Scholar
Askenazi D, Abitbol C, Boohaker L, et al. Neonatal kidney collaborative. Optimizing the AKI definition during first postnatal week using Assessment of Worldwide Acute Kidney Injury Epidemiology in Neonates (AWAKEN) cohort. Pediatr Res. 2019;85:329–338.
Mehta P, Sinha A, Sami A, et al. Incidence of acute kidney injury in hospitalized children. Indian Pediatr. 2012;49:537–42.
Article PubMed Google Scholar
Kaddourah A, Basu RK, Bagshaw SM, Goldstein SL. AWARE Investigators. Epidemiology of acute kidney injury in critically ill children and young adults. N Engl J Med. 2017;376:11–20.
Jetton JG, Boohaker LJ, Sethi SK, et al. Neonatal Kidney Collaborative (NKC). Incidence and outcomes of neonatal acute kidney injury (AWAKEN): A multicentre, multinational, observational cohort study. Lancet Child Adolesc Health. 2017;1:184–94.
Macedo E, Cerdá J, Hingorani S, et al. Recognition and management of acute kidney injury in children: The ISN 0by25 Global Snapshot Study. PLoS ONE. 2018;13:e0196586.
Article PubMed PubMed Central Google Scholar
Kashani K, Macedo E, Burdmann EA, et al. Acute kidney injury risk assessment: Differences and similarities between resource-limited and resource-rich countries. Kidney Int Rep. 2017;2:519–29.
Krishnamurthy S, Mondal N, Narayanan P, Biswal N, Srinivasan S, Soundravally R. Incidence and etiology of acute kidney injury in southern India. Indian J Pediatr. 2013;80:183–9.
Cerdá J, Bagga A, Kher V, Chakravarthi RM. The contrasting characteristics of acute kidney injury in developed and developing countries. Nat Clin Pract Nephrol. 2008;4:138–53.
Messmer AS, Zingg C, Müller M, Gerber JL, Schefold JC, Pfortmueller CA. Fluid overload and mortality in adult critical care patients-A systematic review and meta-analysis of observational studies. Crit Care Med. 2020;48:1862–70.
Alobaidi R, Morgan C, Basu RK, et al. Association between fluid balance and outcomes in critically ill children: A systematic review and meta-analysis. JAMA Pediatr. 2018;172:257–68.
Matsushita FY, Krebs VLJ, de Carvalho WB. Association between fluid overload and mortality in newborns: A systematic review and meta-analysis. Pediatr Nephrol. 2022;37:983–92.
Meena J, Kumar J, Thomas CC, et al. Diagnostic accuracy of renal angina index alone or in combination with biomarkers for predicting acute kidney injury in children. Pediatr Nephrol. 2022;37:1263–75.
Chawla LS, Davison DL, Brasha-Mitchell E, et al. Development and standardization of a furosemide stress test to predict the severity of acute kidney injury. Crit Care. 2013;17:R207.
McMahon BA, Chawla LS. The furosemide stress test: Current use and future potential. Ren Fail. 2021;43:830–9.
Chen JJ, Chang CH, Huang YT, Kuo G. Furosemide stress test as a predictive marker of acute kidney injury progression or renal replacement therapy: A systemic review and meta-analysis. Crit Care. 2020;24:202.
Kakajiwala A, Kim JY, Hughes JZ, et al. Lack of furosemide responsiveness predicts acute kidney injury in infants after cardiac surgery. Ann Thorac Surg. 2017;104:1388–94.
Penk J, Gist KM, Wald EL, et al. Furosemide response predicts acute kidney injury in children after cardiac surgery. J Thorac Cardiovasc Surg. 2019;157:2444–51.
Article CAS PubMed Google Scholar
Borasino S, Wall KM, Crawford JH, et al. Furosemide response predicts acute kidney injury after cardiac surgery in infants and neonates. Pediatr Crit Care Med. 2018;19:310–7.
Krishnasamy S, Sinha A, Bagga A, Hari P. The diagnostic performance of furosemide stress test in predicting progression to acute kidney injury stage 3: Need for kidney replacement therapy and mortality. The 34th Annual Conference of Indian Society of Pediatric Nephrology selected abstracts. Asian J Pediatr Nephrol. 2022;5:3–4.
Van den Eynde J, Schuermans A, Verbakel JY, et al. Biomarkers of acute kidney injury after pediatric cardiac surgery: A meta-analysis of diagnostic test accuracy. Eur J Pediatr. 2022;181:1909–21.
Kotwal S, Herath S, Erlich J, et al. Electronic alerts and a care bundle for acute kidney injury - An Australian cohort study. Nephrol Dial Transplant. 2022:gfac155. https://doi.org/10.1093/ndt/gfac155 .
Akcan-Arikan A, Gebhard D, Arnold MA, Loftis LL, Kennedy CE. Fluid overload and kidney injury score (FOKIS): A multidimensional real-time assessment of renal disease burden in the critically ill patient. Pediatr Crit Care Med. 2017;18:524–30.
Wazir S, Sethi SK, Agarwal G, et al. Neonatal acute kidney injury risk stratification score: STARZ study. Pediatr Res. 2022;91:1141–8.
Ostermann M, Zarbock A, Goldstein S, et al. Recommendations on acute kidney injury biomarkers from the acute disease quality initiative consensus conference: A consensus statement. JAMA Netw Open. 2020;3:e2019209.
Weiss SL, Peters MJ, Alhazzani W, et al. Surviving sepsis campaign international guidelines for the management of septic shock and sepsis-associated organ dysfunction in children. Pediatr Crit Care Med. 2020;21:e52-106.
Malbrain MLNG, Van Regenmortel N, Saugel B, et al. Principles of fluid management and stewardship in septic shock: it is time to consider the four D’s and the four phases of fluid therapy. Ann Intensive Care. 2018;8:66.
Bove T, Belletti A, Putzu A, et al. Intermittent furosemide administration in patients with or at risk for acute kidney injury: Meta-analysis of randomized trials. PLoS ONE. 2018;13:e0196088.
Bagshaw SM, Gibney RTN, Kruger P, Hassan I, McAlister FA, Bellomo R. The effect of low-dose furosemide in critically ill patients with early acute kidney injury: A pilot randomized blinded controlled trial (the SPARK study). J Crit Care. 2017;42:138–46.
Abraham S, Rameshkumar R, Chidambaram M, et al. Trial of furosemide to prevent acute kidney injury in critically ill children: A double-blind, randomized, controlled trial. Indian J Pediatr. 2021;88:1099–106.
Kellum JA, Lameire N. KDIGO AKI Guideline Work Group. Diagnosis, evaluation, and management of acute kidney injury: A KDIGO summary (Part 1). Crit Care. 2013;17:204.
Hoff BM, Maker JH, Dager WE, Heintz BH. Antibiotic dosing for critically ill adult patients receiving intermittent hemodialysis, prolonged intermittent renal replacement therapy, and continuous renal replacement therapy: An update. Ann Pharmacother. 2020;54:43–55.
Schetz M. Drug dosing in continuous renal replacement therapy: General rules. Curr Opin Crit Care. 2007;13:645–51.
Goldstein SL, Mottes T, Simpson K, et al. A sustained quality improvement program reduces nephrotoxic medication-associated acute kidney injury. Kidney Int. 2016;90:212–21.
Kyle UG, Akcan-Arikan A, Orellana RA, Coss-Bu JA. Nutrition support among critically ill children with AKI. Clin J Am Soc Nephrol. 2013;8:568–74.
Kyle UG, Akcan-Arikan A, Silva JC, Goldsworthy M, Shekerdemian LS, Coss-Bu JA. Protein feeding in pediatric acute kidney injury is not associated with a delay in renal recovery. J Ren Nutr. 2017;27:8–15.
Sethi SK, Maxvold N, Bunchman T, Jha P, Kher V, Raina R. Nutritional management in the critically ill child with acute kidney injury: A review. Pediatr Nephrol. 2017;32:589–601.
Gaudry S, Hajage D, Benichou N, et al. Delayed versus early initiation of renal replacement therapy for severe acute kidney injury: A systematic review and individual patient data meta-analysis of randomised clinical trials. Lancet. 2020;395:1506–15.
Cortina G, McRae R, Hoq M, et al. Mortality of critically ill children requiring continuous renal replacement therapy: effect of fluid overload, underlying disease, and timing of initiation. Pediatr Crit Care Med. 2019;20:314–22.
Modem V, Thompson M, Gollhofer D, Dhar AV, Quigley R. Timing of continuous renal replacement therapy and mortality in critically ill children*. Crit Care Med. 2014;42:943–53.
Ye Z, Wang Y, Ge L, et al. Comparing renal replacement therapy modalities in critically ill patients with acute kidney injury: A systematic review and network meta-analysis. Crit Care Explor. 2021;3:e0399.
Nourse P, Cullis B, Finkelstein F, et al. ISPD guidelines for peritoneal dialysis in acute kidney injury: 2020 Update (paediatrics). Perit Dial Int. 2021;41:139–57.
Fischbach M, Edefonti A, Schroder C, Watson A. European Pediatric Dialysis Working Group. Hemodialysis in children: General practical guidelines. Pediatr Nephrol. 2005;20:1054–66.
Zha J, Li C, Cheng G, Huang L, Bai Z, Fang C. The efficacy of renal replacement therapy strategies for septic-acute kidney injury: A PRISMA-compliant network meta-analysis. Medicine (Baltimore). 2019;98:e15257.
Sethi SK, Mittal A, Nair N, et al. Pediatric Continuous Renal Replacement Therapy (PCRRT) expert committee recommendation on prescribing prolonged intermittent renal replacement therapy (PIRRT) in critically ill children. Hemodial Int. 2020;24:237–51.
Dalbhi SA, Alorf R, Alotaibi M, et al. Sustained low efficiency dialysis is non-inferior to continuous renal replacement therapy in critically ill patients with acute kidney injury: A comparative meta-analysis. Medicine (Baltimore). 2021;100:e28118.
Yadav M, Tiwari AN, Lodha R, et al. Feasibility and efficacy of sustained low-efficiency dialysis in critically ill children with severe acute kidney injury. Indian J Pediatr. 2022. https://doi.org/10.1007/s12098-022-04214-z .
Shiri S, Naik NM, Av L, Vasudevan A. Sustained low efficiency dialysis in critically ill children with acute kidney injury: Single-center observational cohort in a resource-limited setting. Pediatr Crit Care Med. 2022. https://doi.org/10.1097/PCC.0000000000003127 .
Article Google Scholar
Raina R, Agrawal N, Kusumi K, Pandey A, Tibrewal A, Botsch A. A meta-analysis of extracorporeal anticoagulants in pediatric continuous kidney replacement therapy. J Intensive Care Med. 2022;37:577–94.
Goldstein SL, Vidal E, Ricci Z, et al. Survival of infants treated with CKRT: comparing adapted adult platforms with the Carpediem™. Pediatr Nephrol. 2022;37:667–75.
Menon S, Broderick J, Munshi R, et al. Kidney support in children using an ultrafiltration device: A multicenter, retrospective study. Clin J Am Soc Nephrol. 2019;14:1432–40.
Raina R, Sethi SK, Filler G, et al. PCRRT Expert Committee ICONIC position paper on prescribing kidney replacement therapy in critically sick children with acute liver failure. Front Pediatr. 2022;9:833205.
Alexander EC, Deep A. Therapeutic plasma exchange in children with acute liver failure (ALF): is it time for incorporation into the ALF armamentarium? Pediatr Nephrol. 2022;37:1775–88.
Raina R, Bedoyan JK, Lichter-Konecki U, et al. Consensus guidelines for management of hyperammonaemia in paediatric patients receiving continuous kidney replacement therapy. Nat Rev Nephrol. 2020;16:471–82.
Matuszkiewicz-Rowinska J, Malyszko J. Prevention and treatment of tumor lysis syndrome in the era of onco-nephrology progress. Kidney Blood Press Res. 2020;45:645–60.
Raina R, Mawby I, Chakraborty R, et al. Acute kidney injury in COVID-19 pediatric patients in North America: Analysis of the virtual pediatric systems data. PLoS ONE. 2022;17:e0266737.
Raina R, Chakraborty R, Sethi SK, Bunchman T. Kidney replacement therapy in COVID-19 induced kidney failure and septic shock: A Pediatric Continuous Renal Replacement Therapy [PCRRT] position on emergency preparedness with resource allocation. Front Pediatr. 2020;8:413.
Greenberg JH, Coca S, Parikh CR. Long-term risk of chronic kidney disease and mortality in children after acute kidney injury: a systematic review. BMC Nephrol. 2014;15:184.
Download references
Acknowledgements
The authors acknowledge the support of the Indian Council of Medical Research (5/7/1090/2013-RHN).
Author information
Authors and affiliations.
Department of Pediatrics, Jawaharlal Institute of Postgraduate Medical Education and Research, Puducherry, India
Sudarsan Krishnasamy
Division of Nephrology, Department of Pediatrics, All India Institute of Medical Sciences, New Delhi, India
Aditi Sinha & Arvind Bagga
You can also search for this author in PubMed Google Scholar
Contributions
SK made substantial contribution to the acquisition, analysis, and interpretation of data and drafted the manuscript; AS, SK, and AB made substantial contributions to the draft of the manuscript, revised it critically, and approved the final manuscript for submission; AB agrees to be accountable for all aspects of the work in ensuring that questions related to the accuracy and integrity of the work are appropriately investigated and resolved. AB will act as the guarantor for this paper.
Corresponding author
Correspondence to Aditi Sinha .
Ethics declarations
Conflict of interest, additional information, publisher's note.
Springer Nature remains neutral with regard to jurisdictional claims in published maps and institutional affiliations.
Supplementary Information
Below is the link to the electronic supplementary material.
Supplementary file1 (ODT 15.3 KB)
Rights and permissions.
Springer Nature or its licensor (e.g. a society or other partner) holds exclusive rights to this article under a publishing agreement with the author(s) or other rightsholder(s); author self-archiving of the accepted manuscript version of this article is solely governed by the terms of such publishing agreement and applicable law.
Reprints and permissions
About this article
Krishnasamy, S., Sinha, A. & Bagga, A. Management of Acute Kidney Injury in Critically Ill Children. Indian J Pediatr 90 , 481–491 (2023). https://doi.org/10.1007/s12098-023-04483-2
Download citation
Received : 17 July 2022
Accepted : 09 January 2023
Published : 02 March 2023
Issue Date : May 2023
DOI : https://doi.org/10.1007/s12098-023-04483-2
Share this article
Anyone you share the following link with will be able to read this content:
Sorry, a shareable link is not currently available for this article.
Provided by the Springer Nature SharedIt content-sharing initiative
- Kidney replacement therapy
- Find a journal
- Publish with us
- Track your research

An official website of the United States government
The .gov means it’s official. Federal government websites often end in .gov or .mil. Before sharing sensitive information, make sure you’re on a federal government site.
The site is secure. The https:// ensures that you are connecting to the official website and that any information you provide is encrypted and transmitted securely.
- Publications
- Account settings
Preview improvements coming to the PMC website in October 2024. Learn More or Try it out now .
- Advanced Search
- Journal List
- Kidney Res Clin Pract
- v.42(3); 2023 May
- PMC10265216
Acute kidney injury in patients with acute-on-chronic liver failure: clinical significance and management
Jeong-ju yoo.
1 Division of Gastroenterology and Hepatology, Department of Internal Medicine, Soonchunhyang University Bucheon Hospital, Soonchunhyang University School of Medicine, Bucheon, Republic of Korea
Moo Yong Park
2 Division of Nephrology, Department of Internal Medicine, Soonchunhyang University Bucheon Hospital, Soonchunhyang University School of Medicine, Bucheon, Republic of Korea
Sang Gyune Kim
Acute-on-chronic-liver failure (ACLF) refers to a phenomenon in which patients with chronic liver disease develop multiple organ failure due to acute exacerbation of underlying liver disease. More than 10 definitions of ACLF are extant around the world, and there is lack of consensus on whether extrahepatic organ failure is a main component or a consequence of ACLF. Asian and European consortiums have their own definitions of ACLF. The Asian Pacific Association for the Study of the Liver ACLF Research Consortium does not consider kidney failure as a diagnostic criterion for ACLF. Meanwhile, the European Association for the Study of the Liver Chronic Liver Failure and the North American Consortium for the Study of End-stage Liver Disease do consider kidney failure as an important factor in diagnosing and assessing the severity of ACLF. When kidney failure occurs in ACLF patients, treatment varies depending on the presence and stage of acute kidney injury (AKI). In general, the diagnosis of AKI in cirrhotic patients is based on the International Club of Ascites criteria: an increase of 0.3 mg/dL or more within 48 hours or a serum creatinine increase of 50% or more within one week. This study underscores the importance of kidney failure or AKI in patients with ACLF by reviewing its pathophysiology, prevention methods, and treatment approaches.
Introduction
Broadly, there are three clinical stages of liver cirrhosis; compensated cirrhosis, decompensated cirrhosis, and late decompensated cirrhosis (DC) [ 1 ]. However, in 1997, Jalan et al. [ 2 ] reported several cases of young cirrhotic patients who died shortly after a transjugular intrahepatic portosystemic shunt procedure for variceal bleeding due to increased intracranial pressure and rapid liver function deterioration. It is inappropriate to classify these cases into the extant cirrhosis stages because increased intracranial pressure is frequently found in acute liver failure rather than in DC. Accordingly, acute exacerbation of existing chronic liver disease has been officially referred to as acute-on-chronic-liver failure (ACLF) [ 3 ].
As suggested, ACLF refers to a specific condition of preexisting chronic liver disease (with or without liver cirrhosis) that is associated with rapid deterioration of liver function, multiorgan failure, and increased mortality [ 4 – 9 ]. The prognosis of ACLF is notably different from that of cirrhosis in that ACLF tends to get worse suddenly [ 4 , 10 ]. The socioeconomic burden of ACLF is estimated to be much larger than that of liver cirrhosis. In the United States, significantly greater per capita hospitalization costs ($51,000 vs. $14,000), hospitalization days (16 days vs. 7 days), and mortality rates (50% vs. 7%) were reported in ACLF patients than in liver cirrhosis patients [ 11 ]. ACLF is comparable to acute kidney injury (AKI) in chronic kidney disease (CKD).
Various definitions of acute-on-chronic-liver failure
Within the field of hepatology, ACLF has been extensively studied, and more than 1,000 papers have been published [ 9 ]. The classification and prognosis of ACLF vary across countries, race and ethnic groups, and etiologies, reflecting inconsistent views, and there are more than 10 definitions of ACLF around the world. The most widely accepted clinical definitions are those outlined by the Asian Pacific Association for the Study of the Liver ACLF Research Consortium (AARC) [ 12 ], the European Association for the Study of the Liver Chronic Liver Failure (EASL-CLIF) [ 13 ], and the North American Consortium for the Study of End-stage Liver disease (NACSELD) [ 14 ]. They are consistent in defining ACLF as a high-mortality disease that involves acute exacerbation of existing liver disease. However, their diagnostic considerations differ in terms of 1) liver condition, 2) organ failures, and 3) precipitating factors. Table 1 presents the most representative diagnostic criteria of the AARC, the EASL-CLIF, and the NACSELD [ 12 – 14 ].
Comparison of ACLF diagnostic criteria
AARC, Asian Pacific Association for the Study of the Liver ACLF Research Consortium; ACLF, acute-on-chronic-liver failure; EASL-CLIF, European Association for the Study of the Liver Chronic Liver Failure; HIV, human immunodeficiency virus; NACSELD, North American Consortium for the Study of End-stage Liver disease.
Multiple perspectives on organ failure and the importance of kidney failure
As shown in Table 1 , the AARC considers extrahepatic organ failure a consequence of ACLF and does not include it in the diagnostic criteria. The EASL-CLIF and the NACSELD, however, incorporate extrahepatic organ failure as an important component of the diagnostic criteria for ACLF. To evaluate organ failure, the AARC, the EASL-CLIF, and the NACSELD respectively consider one, five, and four organs ( Table 1 ). The definitions of organ failure of the EASL-CLIF and the AARC are presented in Table 2 [ 5 – 7 ]. The ACLF grades are classified depending on the number of organ failures, which is directly related to mortality ( Table 3 ). With a single organ failure, the 28-day mortality rate of ACLF was 6.3%, whereas that for failure of four or more organs reached 88.9% [ 6 , 15 ]. Kidney is known to be easily affected by extrahepatic organ failure in ACLF patients, and the incidence of kidney failure (55.8%) was higher than that of liver failure (43.6%) in the CANONIC study [ 15 , 16 ]. Also, kidney failure can more adversely affect the prognosis of ACLF than can failure of any other single organ [ 17 – 19 ]. In non-kidney organ failure, the 28-day mortality rate of ACLF patients was 6.3%, while that of patients with kidney failure alone reached as high as 18.6% [ 15 ]. Therefore, failure of a single organ other than the kidney is considered ACLF grade 0, whereas failure of the kidney alone is considered ACLF grade 1 ( Table 3 ).
Outlines and definitions of organ failure
AARC, Asian Pacific Association for the Study of the Liver ACLF Research Consortium; CLIF-C OF, CLIF Consortium Organ Failure; EASL-CLIF, European Association for the Study of the Liver Chronic Liver Failure; FiO 2 , fraction of inspired oxygen; PaO 2 , partial pressure of oxygen; SpO 2 , peripheral oxygen saturation.
ACLF, acute-on-chronic-liver failure; AARC, Asian Pacific Association for the Study of the Liver ACLF Research Consortium; EASL-CLIF, European Association for the Study of the Liver Chronic Liver Failure.
Definitions of acute kidney injury and hepatorenal syndrome in patients with cirrhosis
For diagnosis of AKI in cirrhosis patients, the definition of the International Club of Ascites (ICA) and KDIGO (Kidney Disease: Improving Global Outcomes) AKI guidelines have been most widely used [ 20 , 21 ]. In 2007, the ICA introduced the concepts of hepatorenal syndrome (HRS)-1 and -2, characterized by rapid deterioration and refractory ascites, respectively [ 22 ]; the concepts of AKI and HRS were updated in 2015 [ 20 ]. AKI was defined as serum creatinine level of 1.5 mg/dL or greater or a serum creatinine increase of 50% or greater compared to the baseline value [ 22 ]. However, AKI was redefined as a serum creatinine increase by 0.3 mg/dL or greater within 48 hours or a serum creatinine increase by 50% or greater compared to the baseline value within 1 week. This was an important change that eliminated the absolute standard of 1.5 mg/dL of serum creatinine level in the diagnosis of AKI. Table 4 summarizes the most commonly used diagnostic standards for HRS and guidelines for determining AKI stages (adapted from the ICA definition) [ 20 ]. Due to poor nutritional status and low muscle mass in cirrhotic patients, there have been persistent concerns that the creatinine-based glomerular filtration rate (GFR) formula might overestimate actual kidney function [ 23 ]. In particular, overestimation of renal function with the creatinine-based GFR formula reached up to about 50% in patients with severely impaired liver function [ 24 ]. Thus, clinicians should pay attention to deterioration of renal function and start treatment for AKI in a timely manner, well before serum creatinine level increases and reaches 1.5 mg/dL. Studies have shown that high AKI grade is associated with poor prognosis in cirrhotic patients. Even in cirrhotic patients with AKI grade I, 3-month survival rate was reduced to 84% [ 25 – 27 ].
The (ICA-AKI) definitions of AKI and HRS in patients with cirrhosis
AKI, acute kidney injury; HRS, hepatorenal syndrome; ICA, International Club of Ascites; sCr, serum creatinine; NSAIDs, nonsteroidal anti-inflammatory drugs.
Meanwhile, as the definition of AKI in cirrhosis patients was updated and the absolute value of serum creatinine is no longer involved, HRS-1, which was previously determined by an increase of serum creatinine level 2.5 mg/dL or greater, was renamed HRS-AKI [ 20 ]. These changes are primarily based on the low response rate of patients with high serum creatinine to treatment with terlipressin plus albumin for HRS [ 28 – 30 ]. The newly revised definition recommends starting treatment with vasoconstrictor and albumin in the early stage of AKI, even if serum creatinine level is less than 2.5 mg/dL. The level of serum creatinine tends to overestimate renal function in cirrhotic patients and varies greatly by day. Hence, absolute standards such as serum creatinine level of 1.5 mg/dL (AKI) or 2.5 mg/dL (HRS) should be avoided, and clinicians need to start treating AKI and HRS as early as possible in consideration of the dynamic fluctuation present in serum creatinine level.
In the same vein, attempts have been made to rename HRS type 2, which is characterized by a slow increase of serum creatinine and refractory ascites, to HRS-non-AKI (NAKI) and to have it be divided into HRS-acute kidney disease and HRS-CKD depending on the rate of exacerbation [ 31 ]. However, HRS-NAKI is rarely reported and analyzed in ACLF patients, so we will not discuss it in detail in this review.
Prevalence of acute kidney injury in patients with acute-on-chronic-liver failure
According to the CANONIC study, the prevalence of AKI in ACLF patients is 69% [ 15 ]. Among them, the proportions of kidney dysfunction (serum creatinine level of 1.5–1.9 mg/dL) and kidney failure (serum creatinine level of >2 mg/dL) cases were respectively 19.1% and 80.9% [ 15 ]. These reports were made prior to revision of the ICA-AKI standard in 2015. Thus, the values are likely to be higher if the current standards of AKI are applied. Even in the ACLF patient group based on the AARC criteria, the prevalence of AKI was estimated to be around 22.8% to 51%, much higher than that (20%) in cirrhotic patients without ACLF [ 12 ]. In research conducted in South Korea, 340 among 1,470 patients (23.1%) hospitalized for chronic liver disease had ACLF, and 49% of ACLF patients had kidney failure [ 32 ].
Differences in characteristics of acute kidney injury between acute-on-chronic-liver failure and decompensated cirrhosis
In the past, the concept of ACLF was not well established, and there was a lack of distinction between cirrhotic patients with DC and those with ACLF in terms of reported prognosis and clinical outcome. Accumulating evidence suggests that the pattern of organ failure between ACLF and DC is different [ 33 ]. Even though the incident rate of AKI was not significantly different between ACLF and DC patient groups (around 13%–25%), the phenotype of AKI was notably different [ 34 , 35 ]. The DC-AKI group tended to be functional and volume-responsive, while the ACLF-AKI group had more severe structural damage [ 33 ]. In addition, the probability of AKI progression was relatively low in the DC-AKI group, and resolution was rare [ 33 ]. On the other hand, the ACLF-AKI group had a high possibility of AKI progression, as well as of resolution [ 33 , 34 ]. The ACLF-AKI group required renal replacement therapy (RRT) more frequently and had lower response rate to terlipressin than the DC-AKI group, possibly due to severe and extensive systemic inflammation. Table 5 summarizes the differences in characteristics of the ACLF-AKI and DC-AKI groups. However, more studies are needed to better understand the difference between DC-AKI and ACLF-AKI groups.
Differences in characteristics of AKI between ACLF and DC
ACLF, acute-on-chronic-liver failure; AKI, acute kidney injury; DC, decompensated cirrhosis; RRT, renal replacement therapy.
A prior study on the difference between DC and ACLF patient groups suggests that they have different metabolic profiles such that ACLF patients showed increased skeletal muscle catabolism resulting in release of amino acid to increase nonessential amino acid/glucose and methionine compared with DC patients [ 36 ]. Also, the level of spermidine, an inducer of anti-inflammatory autophagy, was decreased in ACLF patients [ 36 ].
Another difference between DC and ACLF patient groups is the gut microbiome. Progression of cirrhosis was associated with a reduction of gene and metagenomic species richness in the gut, with maximal changes in ACLF patients [ 37 ].
Precipitating factors
Common causes of AKI in general cirrhotic patients include use of diuretics, gastrointestinal bleeding, large volume paracentesis, infection, and use of nephrotoxic drugs such as nonsteroidal anti-inflammatory drugs (NSAIDs) [ 38 – 40 ]. The precipitating factors of AKI and ACLF are similar. ACLF can be precipitated by both hepatic and nonhepatic factors [ 41 , 42 ]. In the AARC criteria, acute exacerbation caused by nonhepatic precipitating factors such as infection and gastrointestinal bleeding is excluded from the ACLF definition. However, both nonhepatic and hepatic precipitating factors are considered in the EASL-CLIF criteria. According to studies conducted in Western countries (CANONIC study, EASL-CLIF cohort), the most important causes of acute exacerbation were active alcohol drinking and bacterial infection [ 15 ]. On the other hand, according to studies conducted in Eastern countries, hepatitis B virus reactivation, hepatitis A or E virus infection, active alcohol drinking, and bacterial infection were major precipitating factors [ 43 , 44 ]. In South Korean ACLF patients, the most common precipitating factor was active alcohol drinking (41%), followed by gastrointestinal bleeding (31%) and bacterial infection (18.5%) [ 32 ]. However, the cause of acute exacerbation in about 40% to 50% of ACLF patients is not clearly elucidated in many studies [ 15 , 45 ]. In patients with unclear precipitating factors, drug-induced liver injury might be one viable reason, but studies on precipitating factors of ACLF-AKI are lacking [ 46 ].
Pathogenesis of acute kidney injury in acute-on-chronic-liver failure
The mechanism of AKI in patients with ACLF is complex and might involve multiple factors such as hemodynamic abnormality, inflammation, and oxidative stress [ 5 , 16 , 47 ]. Among these, systemic inflammation is a key factor of AKI pathogenesis in ACLF patients [ 48 ]. Systemic inflammation in ACLF is usually induced by damage-associated molecular patterns (DAMPs) and body fluids containing pathogen-associated molecular patterns (PAMPs) [ 49 – 51 ]. Bacterial translocation often occurs in cirrhotic patients due to intestinal bacterial overgrowth, increased intestinal mucosa permeability, and impaired intestinal innate immune, and these PAMPs may be a main cause of ACLF [ 52 – 54 ]. However, alcohol-induced DAMPs can be activated in the absence of infection, especially in severe alcoholic hepatitis, and can lead to increased endoplasmic reticulum stress and hepatocyte injury [ 55 , 56 ].
AKI in cirrhosis is initially caused by decreased effective vascular volume due to splanchnic vasodilatation and renal hypoperfusion. However, prolonged duration of AKI is associated with gradual renal parenchymal damage, leading to capillary leukocyte infiltration, vascular microthrombosis, and cell apoptosis [ 57 , 58 ]. In particular, parenchymal damage of the kidney is frequently found in a more advanced stage of AKI or HRS-AKI and is closely related to the low response rate to the treatment with terlipressin plus albumin (i.e., less than 50%) [ 28 , 59 ]. That is, splanchnic vasodilatation and renal artery vasoconstriction may be involved in the pathogenesis of AKI in the early stage, while renal parenchymal damage may also be an important element of AKI pathogenesis in the later stages. Recently, it has been also reported that bile cast nephropathy may play an important role in the pathogenesis of AKI in patients with prolonged jaundice, and further studies are needed [ 60 ].
Biomarkers of acute kidney injury in acute-on-chronic-liver failure
The mortality rate is higher in ACLF patients with AKI than in those without AKI [ 27 ]. Many studies on biomarkers of AKI have been conducted. However, almost all reported biomarkers for diagnosis and prognosis of AKI were identified in heterogeneous populations comprising various stages of AKI; caution is required in their interpretation. Many studies have been published on biomarkers of AKI in cirrhosis patients, while only three biomarkers of AKI in patients with ACLF have been reported: cystatin C, neutrophil gelatinase-associated lipocalin (NGAL), and serum creatinine. The analysis of 429 patients in the CANONIC study cohort showed that baseline cystatin C was a useful biomarker predicting the development of renal dysfunction and ACLF, which was directly related to mortality [ 61 ]. Also, cystatin C was a more effective predictor of renal dysfunction than creatinine in ACLF of HBV patients [ 62 ]. However, NGAL did not predict the occurrence of renal dysfunction or ACLF although it was associated with short-term mortality [ 61 ]. Interleukin-18 and kidney injury molecule-1 have been examined in cirrhosis patients without ACLF but not in ACLF patients [ 63 ]. One of the major limitations of the studies on AKI biomarkers is that cystatin C or NGAL may be affected by the degree of inflammation [ 24 ]. In addition, specific cut-off values for the overlapping phenotypes were not available, limiting clinical utility [ 63 ].
Last, serum creatinine, which has been traditionally used, can also be an important biomarker in HRS-AKI patients. In HRS-AKI patients with ACLF, a higher level of serum creatinine was associated with lower response rate to terlipressin [ 64 ]. Therefore, serum creatinine is an important predictor of response to treatment when HRS-AKI occurs in ACLF patients.
Prevention of acute kidney injury
In cirrhosis patients, AKI prevention plays a critical role in prognosis. Avoidance of nephrotoxic drugs and appropriate control of volume status are crucial. The problem is that it is very difficult to control the volume status in patients with cirrhosis and decreased renal function [ 65 ]. NSAIDs and antibiotics are highly likely to promote AKI in cirrhosis patients should be administered with extra caution and careful determination of dose [ 66 ]. There is no evidence that contrast-induced nephropathy occurs more frequently in cirrhotic patients than in the general population. Nevertheless, caution is always required because cirrhotic patients require more frequent surveillance or examination than the general population [ 67 ]. In addition, frequent follow-up with serum creatinine is necessary in cirrhotic patients with ascites because of the high risk of AKI.
Antibiotic prophylaxis may help prevent AKI. Prophylactic antibiotics are administered in patients with frequent spontaneous bacterial peritonitis (SBP) or variceal bleeding. Systemic inflammation frequently caused by bacterial translocation has been pointed out as the main cause of acute exacerbation; hence, antibiotic prophylaxis that prevents repeated SBP can also prevent AKI [ 68 , 69 ]. Antibiotic prophylaxis is also recommended in patients with varix bleeding to reduce the likelihood of systemic infection and to increase survival [ 70 , 71 ]. In both instances (i.e., SBP and variceal bleeding), there were promising findings that antibiotic prophylaxis could lower the incidence of AKI [ 72 , 73 ].
Albumin infusion may help prevent AKI in two ways. First, large volume paracentesis can induce AKI by causing circulatory dysfunction and hypovolemia; hence, albumin replacement is essential to prevent AKI during large volume paracentesis [ 74 ]. Second, albumin is also recommended to prevent AKI in patients with SBP [ 75 ]. However, the clinical utility of albumin might be limited in systemic infections other than SBP [ 8 ]. A recent study showed that weekly albumin administration can be helpful for long-term prognosis of cirrhosis, demonstrating the anti-inflammatory effect of albumin beyond its volume replacing effect [ 76 ]. However, more empirical evidence is needed to safely administer weekly albumin in patients with liver cirrhosis considering cost-effectiveness.
Last, there was a study showing that administration of N -acetylcysteine (NAC) can help prevent AKI in alcoholic hepatitis patients [ 77 ]. In addition, one study reported that short-term granulocyte colony-stimulating factor (G-CSF) plus erythropoietin (darbepoetin) can decrease AKI, sepsis, and mortality in DC patients [ 78 ]. The effectiveness of NAC or G-CSF has not been clearly demonstrated, and additional results are needed.
Treatment of acute kidney injury in acute-on-chronic-liver failure
First, if the underlying cause of AKI is evident, treatment of the underlying condition needs to be prioritized. For example, antibiotic treatment can be considered for AKI caused by bacterial infection, volume replacement can be administered to treat hypovolemia due to gastrointestinal bleeding, and any causative agent needs to be discontinued to prevent AKI. Also, prednisolone therapy can be considered for severe alcoholic hepatitis [ 79 ]. If these underlying conditions are addressed as early as possible, AKI progression may be suppressed. Subsequently, albumin infusion is recommended for volume expansion. Usually, 1 g of albumin per kg of body weight is recommended for 1 to 2 days, after which the improvement of AKI can be evaluated [ 20 ]. If there is no response to albumin infusion, additional biomarker testing such as urine NGAL might be considered [ 80 ]. If the level of urine NGAL is elevated, it is most likely a phenotype of acute tubular necrosis [ 63 , 81 ].
When AKI continues to progress despite these treatments, vasoconstrictors such as terlipressin with albumin may be considered. However, the use of vasoconstrictor is not recommended for stage I AKI [ 8 ]. Treatment response to terlipressin in patients with ACLF accompanying HRS-AKI is reported to be around 35% [ 19 , 82 ]. The response rate to terlipressin is particularly low in patients with HRS-AKI when the baseline serum creatinine is elevated, bilirubin level is heightened, or mean arterial pressure is not elevated despite terlipressin administration [ 28 , 83 ]. If there is no improvement in serum creatinine after 7 to 10 days of terlipressin treatment, it should be discontinued.
ACLF patients with AKI have a high mortality rate; hence, liver transplantation (LT) should be considered. Usually, the prognosis after LT in the presence of one or two organ failures is not different from that of patients without ACLF [ 84 ]. However, when there are three or more organ failures, the 1-year posttransplantation survival rate tends to decrease slightly to 80%. Nevertheless, it is recommended to consider LT first in patients with grade 3 ACLF, because the survival rate is less than 20% if LT is not performed [ 85 , 86 ].
Liver-kidney co-transplantation might be a viable option for patients with estimated GFR (eGFR) of <30 mL/min/1.73 m 2 or underlying CKD, who are highly likely to need dialysis later [ 80 , 87 ]. However, studies on liver-kidney co-transplantation in ACLF patients are lacking due to donor shortage. In patients who underwent LT only, the rate of renal dysfunction after transplantation was increased proportionally to the pretransplantation AKI duration [ 88 , 89 ]. In particular, patients with AKI for more than 6 weeks were more likely to experience renal dysfunction after LT [ 90 ]. Therefore, liver-kidney co-transplantation should be considered first in the earlier-mentioned conditions [ 16 ]. In updated Organ Sharing/Organ Procurement and Transplantation Network criteria, liver-kidney co-transplantation is recommended for the following three conditions [ 91 – 93 ]: 1) CKD with a measured or eGFR of <60 mL/min/1.73 m 2 for more than 90 consecutive days, 2) sustained AKI, or 3) accompanying metabolic disease such as hyperoxaluria or atypical hemolytic uremic syndrome.
RRT can be performed as a bridging therapy if AKI continues to worsen in patients for whom immediate LT is not available [ 94 , 95 ]. Studies reported that continuous RRT rather than intermittent RRT improves cardiovascular stability and reduces intracranial pressure in patients with cirrhosis [ 96 – 98 ]. However, there is no randomized controlled trial comparing continuous and intermittent RRT, and RRT by itself does not reduce mortality in ACLF or cirrhotic patients.
Last, artificial liver treatment such as that with a Molecular Adsorbent Recirculating System (MARS) can be considered as a bridging therapy in ACLF patients, although it may not improve survival [ 99 , 100 ]. Due to its limited effectiveness and high cost, MARS is not widely used.
ACLF is an acute exacerbation of preexisting liver disease, resulting in high mortality and high socioeconomic burden. In patients with ACLF, the kidney is the most common site of organ failure, and the prognosis is poor when AKI occurs. AKI in patients with ACLF is clinically different from AKI in patients with decompensated liver cirrhosis, but more research is needed to elucidate its mechanism. AKI associated with ACLF develops through multifactorial pathogeneses involving both hemodynamic abnormality and inflammation, especially systemic inflammation, bacterial translocation, and infection. Prophylactic antibiotic administration or albumin replacement might be effective in preventing AKI. Treatment for AKI includes management of underlying disease, volume replacement, vasoconstrictor with albumin, RRT, and LT. More studies are needed to advance the understanding of ACLF-AKI and its prevention and treatment.
Conflicts of interest
All authors have no conflicts of interest to declare.
This research was funded by the Basic Science Research Program through the National Research Foundation of Korea (NRF), funded by the Ministry of Education, Science, and Technology (2021R1G1A1007886), and in part by the Soonchunhyang University Research Fund.
Data sharing statement
The data presented in this study are available on request from the corresponding author.
Authors’ contributions
Conceptualization, Funding acquisition: SGK
Investigation: JJY
Resources: MYP
Writing–original draft: JJY
Writing–review & editing: SGK
All authors read and approved the final manuscript.
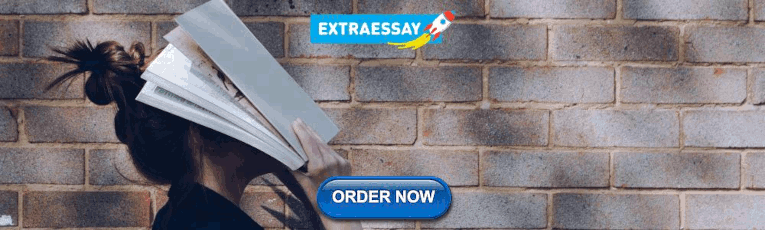
IMAGES
VIDEO
COMMENTS
Acute kidney injury (AKI) is a complex syndrome for which no effective treatment exists, and our understanding of this condition is limited. If the acute kidney injury is severe enough to ... This thesis is based on the following papers, which will be referred to by their Roman
Background Acute kidney injury (AKI) is a major global health problem. We aim to evaluate the epidemiology, risk factors and outcomes of AKI episodes in our single centre. Methodology We prospectively identified 422 AKI and acute on chronic kidney disease episodes in 404 patients meeting KDIGO definitions using electronic medical records and clinical data from 15th July to 22nd October 2016 ...
INTRODUCTION. Acute kidney injury (AKI) is an acute decrease in kidney function defined by an increase in serum creatinine (SCr) or a decrease in urine output (UO) [1, 2].The incidence of AKI has increased in the past decades, reflecting the increased recognition of this diagnosis, patient ageing and increase in AKI risk factors and co-morbidities [diabetes, hypertension, chronic kidney ...
Acute Kidney Injury (AKI) is the term that has recently replaced the term ARF. AKI is defined as an abrupt (within hours) decrease in kidney function, which encompasses both injury (structural damage) and impairment (loss of function). It is a syndrome that rarely has a sole and distinct pathophysiology. Many patients with AKI have a mixed ...
1. Introduction. Acute kidney injury (AKI) is a frequent diagnosis with an incidence that varies from 5.0% to 7.5% in hospitalized patients and that reaches up to 50-60% in critically ill patients [1,2,3,4,5,6].AKI is characterized by an acute decrease in renal function that can be multifactorial in its origin and is associated with complex pathophysiological mechanisms [1,7].
Acute kidney injury (AKI) is associated with adverse long-term outcomes, but many studies are retrospective, focused on specific patient groups or lack adequate comparators. The ARID (AKI Risk in ...
Introduction. Acute kidney injury (AKI) is a common and serious clinical event that affects up to 15.0% of all hospitalized and up to 50.0% intensive care unit patients [].In studies using definitions conforming to the Kidney disease: improving global outcomes criteria (KDIGO), the pooled rate of AKI was 23.2% worldwide, and the AKI-associated mortality was 23.0% [].
Several studies and meta-analyses have been performed in the last 10 years examining the impact of chronic kidney disease in rates of acute kidney injury in hospital inpatients, and the impact of de novo acute kidney injury on subsequent kidney function and rates of end stage renal disease in survivors. n=233,803 Inpatients aged>67yrs.
A dissertation submitted in part fulfilment of the requirements for the degree of Master of Medicine In Internal Medicine Dar es Salaam, Tanzania 17/06/2022. ii . Aga Khan University . ... Acute kidney injury is a potentially fatal but preventable outcome of percutaneous coronary
Acute kidney injury (AKI) is an essential but complex syndrome that results from heterogeneous mechanisms and carries considerable morbidity and mortality [1,2]. AKI occurs in 10%-15% of hospitalized patients and 50% in the intensive care unit (ICU) set-ting [3,4]. AKI is recognized as a decrease in glomerular filtration rate (GFR), and the
Advances in pediatric acute kidney injury. Rupesh Raina1,2, Ronith Chakraborty1,2, Abhishek Tibrewal2, Sidharth K. Sethi3 and Timothy Bunchman4. The objective of this study was to inform the ...
Other consequences of acute renal failure include metabolic acidosis, electrolyte abnormalities such as hyperkalaemia and changes in body fluid balance. All these, if severe, may be life threatening (1). In population studies, the incidence of acute kidney injury is rising.
Acute kidney injury (AKI) superimposed on chronic liver disease and cirrhosis is common and consists of varying phenotypes. Prerenal renal dysfunction caused by severe hypoalbuminemia is the most common clinical syndrome in patients with advanced liver disease. Although prerenal azotemia seems to be the first phase of AKI, it is difficult to ...
Sepsis-associated acute kidney injury (S-AKI) is a frequent complication of the critically ill patient and is associated with unacceptable morbidity and mortality. Prevention of S-AKI is difficult because by the time patients seek medical attention, most have already developed acute kidney injury. Thus, early recognition is crucial to provide supportive treatment and limit further insults ...
Acute kidney injury (AKI) is a common complication in cardiac surgery patients, with a reported incidence of 20 to 30%. The development of AKI is associated with worse short- and long-term mortality, and longer hospital length of stay. The pathogenesis of cardiac surgery-associated AKI is poorly understood but likely involves an interplay ...
Acute kidney injury (AKI) is a rapid deterioration in kidney function that occurs within 48 hours of an initiating event and is associated with an absolute increase in serum creatinine of ≥26.4 ...
Introduction. The incidence of acute kidney injury (AKI), a serious and common problem associated with a high mortality rate, is increasing [1-5].The risk of chronic kidney disease (CKD) and end-stage renal disease (ESRD) increases by 8.8-fold and 3.3-fold, respectively, in surviving and discharged patients with AKI [].To enable early AKI recognition and sufficient response, several damage ...
Acute kidney injury . Acute kidney injury is defined by the KDIGO classification as one of the following (KDIGO, 2012; Mehta et al., 2007); • An increase in serum creatinine by 26.53 micromole/l (0.3 mg/dl) or more within 48 hours OR • An increase in serum creatinine to 1.5 times the baseline or more within the last 7 days OR
Acute kidney injury (AKI) is common in critically ill patients, affecting almost one in four critically ill children and one in three neonates. Higher stages of AKI portend worse outcomes. Identifying AKI timely and instituting appropriate measures to prevent and manage severe AKI is important, since it is independently associated with mortality. Methods to predict severe AKI should be applied ...
Introduction. Acute kidney injury (AKI) is one of the most frequent organ failure encountered in intensive care units (ICU). Since his first definition by Homer W. Smith in the fifties [ 1 ], more than 30 different definitions have been used, leading to a sizeable epidemiological heterogeneity [ 2] with incidence ranging from 5 [ 3] to 25% [ 4 ].
Acute kidney injury (AKI) is a major global health problem in both developed and developing nations, negatively affecting patient morbidity and responsible for an estimated 1.4 million deaths per ...
Treatment of acute kidney injury in acute-on-chronic-liver failure. First, if the underlying cause of AKI is evident, treatment of the underlying condition needs to be prioritized. For example, antibiotic treatment can be considered for AKI caused by bacterial infection, volume replacement can be administered to treat hypovolemia due to ...