Thank you for visiting nature.com. You are using a browser version with limited support for CSS. To obtain the best experience, we recommend you use a more up to date browser (or turn off compatibility mode in Internet Explorer). In the meantime, to ensure continued support, we are displaying the site without styles and JavaScript.
- View all journals
- Explore content
- About the journal
- Publish with us
- Sign up for alerts
- Perspective
- Published: 27 April 2022
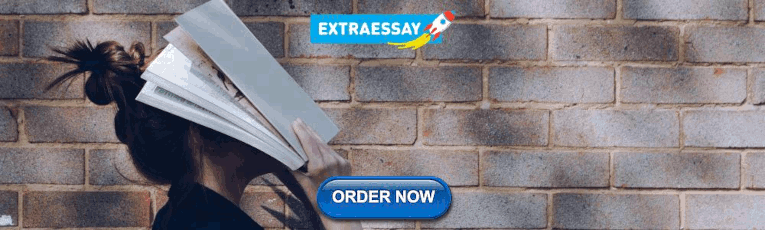
FAIR data enabling new horizons for materials research
- Matthias Scheffler ORCID: orcid.org/0000-0002-1280-9873 1 , 2 ,
- Martin Aeschlimann ORCID: orcid.org/0000-0003-3413-5029 3 ,
- Martin Albrecht 4 ,
- Tristan Bereau 5 ,
- Hans-Joachim Bungartz 6 ,
- Claudia Felser ORCID: orcid.org/0000-0002-8200-2063 7 ,
- Mark Greiner ORCID: orcid.org/0000-0002-4363-7189 8 ,
- Axel Groß ORCID: orcid.org/0000-0003-4037-7331 9 ,
- Christoph T. Koch ORCID: orcid.org/0000-0002-3984-1523 1 ,
- Kurt Kremer 5 ,
- Wolfgang E. Nagel 10 ,
- Markus Scheidgen 1 ,
- Christof Wöll ORCID: orcid.org/0000-0003-1078-3304 11 &
- Claudia Draxl ORCID: orcid.org/0000-0003-3523-6657 1 , 2
Nature volume 604 , pages 635–642 ( 2022 ) Cite this article
13k Accesses
81 Citations
98 Altmetric
Metrics details
- Materials science
The prosperity and lifestyle of our society are very much governed by achievements in condensed matter physics, chemistry and materials science, because new products for sectors such as energy, the environment, health, mobility and information technology (IT) rely largely on improved or even new materials. Examples include solid-state lighting, touchscreens, batteries, implants, drug delivery and many more. The enormous amount of research data produced every day in these fields represents a gold mine of the twenty-first century. This gold mine is, however, of little value if these data are not comprehensively characterized and made available. How can we refine this feedstock; that is, turn data into knowledge and value? For this, a FAIR (findable, accessible, interoperable and reusable) data infrastructure is a must. Only then can data be readily shared and explored using data analytics and artificial intelligence (AI) methods. Making data 'findable and AI ready' (a forward-looking interpretation of the acronym) will change the way in which science is carried out today. In this Perspective, we discuss how we can prepare to make this happen for the field of materials science.
You have full access to this article via your institution.
Similar content being viewed by others
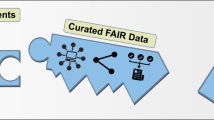
Generating FAIR research data in experimental tribology
Nikolay T. Garabedian, Paul J. Schreiber, … Christian Greiner
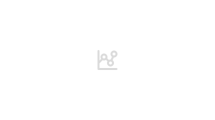
OPTIMADE, an API for exchanging materials data
Casper W. Andersen, Rickard Armiento, … Xiaoyu Yang
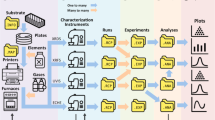
Tracking materials science data lineage to manage millions of materials experiments and analyses
Edwin Soedarmadji, Helge S. Stein, … John M. Gregoire
The number of possible materials is practically infinite. But even for the so-far known materials, our knowledge about their properties and their synthesis is very shallow. There is no doubt that forms of condensed matter exist, or can be created, that show better, or even new, properties and functions than the materials that are known and used today. How can we find them? High-throughput screening of materials — experimentally or theoretically — collects important information. These results will boost new discoveries, but the immensity of possible materials cannot be covered by such explicit searches. Moreover, in current purpose-focused research, only a small fraction of the data produced in the studies is published, and many data are not fully characterized. Furthermore, the metadata (the information that explains and characterizes the measured or calculated data), ontologies (the relationships in metadata) and workflows of different research groups cannot be easily reconciled. Thus, most research data are neither findable nor interoperable.
A FAIR data infrastructure will foster the exchange of scientific information. The meaning of the acronym—that data should be findable, accessible, interoperable and reusable—is explained in the original publication by Wilkinson et al. 1 and elaborated on, for example, at the GO FAIR web pages ( https://go-fair.org/fair-principles/ ). The crucial and very laborious first step towards the FAIRification of data concerns the need to comprehensively describe data by metadata; that is, to characterize data fully and unambiguously so that the research is reproducible. Then scientists, engineers and others can also combine data and metadata from different studies and use them in different contexts. This will open synergies between materials science subdomains and facilitate inter-institute and cross-discipline research. It will also enable data to be used for deeper analyses and for training AI models. Clearly, a FAIR data infrastructure will also show data provenance.
The US Materials Genome Initiative (MGI, https://mgi.gov/ ) was announced in 2011 for “discovering, manufacturing, and deploying advanced materials twice as fast and at a fraction of the cost compared to traditional methods.” It markedly boosted collaborations and high-throughput experiments and computations. FAIRmat ( https://FAIRmat-NFDI.eu/ ) develops the original MGI concept further by implementing a FAIR data infrastructure for condensed-matter physics and the chemical physics of solids. It is a consortium of the German National Research Data Infrastructure programme ( https://nfdi.de ). FAIRmat interweaves data and tools from and for materials synthesis, experiments, theory and computation, and makes all data available to the whole materials science community and beyond. In this endeavour, it unites researchers from condensed matter physics, the chemical physics of solids and computer science and IT experts.
Materials science is strongly affected by all the four Vs (4V) of big data: volume (the amount of data), variety (the heterogeneity of form and meaning of data), velocity (the rate at which data may change or new data arrive) and veracity (the uncertainty of data quality). The various experimental and theoretical examples provided below will illustrate these different aspects. In general, a FAIR data infrastructure requires an in-depth description of how the data have been obtained, addressing metadata, ontologies and workflows. Obviously, only the experts (those creating the samples or computer codes and performing measurements or calculations that is, producing the data) have the insight and knowledge to provide this critical information.
The topic of this Perspective, as outlined above, includes the request for a notable change in scientific culture. Thinking beyond our present research focus on effect, phenomenon or application requires us to accept publishing ‘clean data’—that is, well-characterized and clearly annotated data—represents a value of similar importance to a standard publication, or even higher. This concept carries analogies to Tycho Brahe, who created the data that enabled Johannes Kepler to find his equations and finally led Newton to formulate his theory of gravitation.
Eventually, after having installed an efficient, FAIR research data infrastructure, hosting all data from synthesis, experimental and theoretical studies for a wide range of materials, we also need to pave the way for carrying out new research. Our scientific vision is to build maps of material properties that will guide us in designing and finding new materials for a desired function. This concept follows the spirit of the creation of the periodic table of elements; organizing the roughly 60 atoms known at the time enabled Mendeleev to predict the existence and properties of yet-to-be discovered elements.
In the following, we will describe the state of the art, highlight the challenges and put forward FAIRmat’s envisaged solutions.
Data-centric materials science
Science is and always has been based on data, but the term ‘data-centric’ indicates a radical shift in how information is handled and research is performed. It refers to extensive data collections, digital repositories and new concepts and methods of data analytics. It also implies that we complement traditional purpose-oriented research by using data from other studies.
Some progress in this direction has been made in recent years in terms of collecting data from the many research groups across the planet (all the data, not just what is published in research manuscripts) and making the data FAIR 1 , 2 . This should be good scientific practice in any case 3 , 4 . Since 1965, data repositories in materials science have moved towards digitization. A comprehensive list can be found in ref. 5 . Among them, the NOMAD (Novel Materials Discovery) Laboratory (a database for computational materials science; online since 2014, https://nomad-lab.eu/ ) is unique as it accepts data from practically all computational materials science codes. As it provides the blueprint for FAIRmat, we will summarize its basic concept (for details, see refs. 4 , 6 ). A key guideline of NOMAD (and FAIRmat) is to help scientists and students to upload and download data in the easiest way. In simple terms, data stored at NOMAD are treated analogously to publications at a journal archive, such as https://arxiv.org/ . Unlike journal archives, an embargo period can be used for collaborations with selected colleagues or may even be crucial for collaborations with industry. At the time of writing (August 2021), NOMAD contains results from more than 100 million open-access calculations. These are from individual researchers all over the world and include entries from other computational materials databases, such as AFLOW ( http://aflow.org ), the Materials Project ( https://materialsproject.org ) and OQMD (the Open Quantum Materials Database, http://oqmd.org ). NOMAD converts the data into a common form and provides an easy materials view presentation by means of the NOMAD Encyclopedia ( https://nomad-lab.eu/encyclopedia ). This allows users to see, compare, explore and understand computed materials data. Furthermore, the NOMAD Artificial Intelligence Toolkit ( https://nomad-lab.eu/AIToolkit ) offers tools for data analytics and predictions.
The overall challenges of FAIRmat are sketched in Fig. 1 : besides organizing and — equally importantly — convincing the community (top left), a critical task concerns the development of metadata standards and ontologies (top right). At present, in materials science, such standards are either totally missing or incomplete. Numerous attempts from standards organizations, such as the International Standards Organization ( https://iso.org/ ), to provide controlled vocabularies, standards for data formats and data handling, have so far failed to reach community-wide adoption.
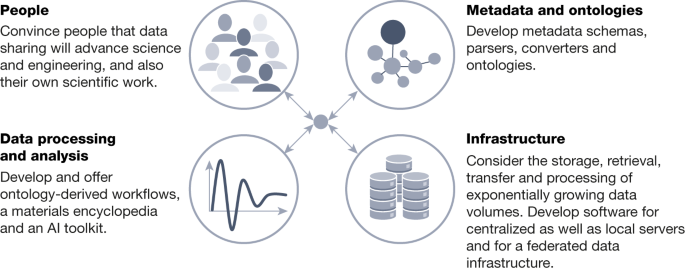
This schematic summarizes the four main categories of challenge faced in implementing FAIRmat.
FAIRmat has already started to establish metadata and dictionaries for digital translations of the vocabulary used in different domains. The next step concerns the description of relations between them, hence, the development of ontologies. They will become particularly important when involved workflows are needed. The NOMAD Meta Info 7 ( https://nomad-lab.eu/metainfo ) stores descriptive and structured information about materials science data and some interdependencies. Thus, it represents an ontology precursor. There are a lot of discussions regarding ontology within the community; see, for example, refs. 7 , 8 , 9 and the metadata and ontology activities at NIST ( https://data.nist.gov/od/dm/nerdm/ ) and the Materials Ontologies RDA Task Group ( https://rd-alliance.org ). This also concerns collaborations of FAIRmat with EMMC ( https://emmc.info ), OPTIMADE 9 ( https://optimade.org ) and NIST ( https://data.nist.gov/ ).
As illustrated in Fig. 1 , data-centric materials science requires a complex infrastructure (bottom right). Established standards for data models in materials science will be considered; for example, CIF (Crystallographic Information Framework, https://iucr.org/resources/cif ), CSMD (Core Scientific Metadata Model, http://icatproject-contrib.github.io/CSMD ) and NeXus ( https://nexusformat.org/ ). Last but not least, acceptance by researchers requires that the infrastructure also offer support and efficient tools for data processing and analysis (Fig. 1 , bottom left).
Other research fields are facing different yet analogous challenges. International contacts, coordination and collaborations of the various fields are promoted by the GO FAIR initiative ( https://go-fair.org/ ), the Research Data Alliance (RDA, https://rd-alliance.org/ ), the association FAIR-DI ( https://fair-di.eu ), CODATA ( https://codata.org/ ) and others. A recent publication 10 by Wittenburg et al. on ‘FAIR practices in Europe’ describes the situation in the areas of humanities, environmental sciences and natural sciences. Although basic concepts and IT tasks are being discussed, true collaborations and reaching the final goal of growing together still need time.
Preparing the research of tomorrow
Putting what is outlined above into practice is a rocky road. To motivate the community to join a culture of extensive data sharing, FAIRmat’s policy is to lead by example. Two issues are obviously important to speed up the process and trigger active support: (1) successful, living examples of daily data-centric research 11 to demonstrate what and how things work; and (2) outreach to the wider community, including the education of future scientists and engineers.
To cope with the first point, FAIRmat will demonstrate its approach with specific examples from diverse research fields, including battery research, heterogeneous catalysis, optoelectronics, magnetism and spintronics, multifunctional materials and biophysics. In all of this, FAIRmat will demonstrate the synergistic interplay of materials synthesis, sample preparation and experiments, as well as theory and computation, and provide a much more comprehensive picture than the single subcommunities can achieve. As such, FAIRmat will bring together not only data and tools but, most notably, also people, who will learn each other’s ‘language’. In fact, the necessary width of competences goes along with a diversity in the nomenclature, which can hamper communication as well as the definition of metadata and ontologies. Likewise, electronic lab notebooks (ELNs) must be standardized to allow seamless integration of data into automatic workflows. Dedicated data-analysis and AI tools will be developed and demonstrated that help to identify the key descriptive physicochemical parameters 12 , 13 , 14 , 15 . This will allow for predictions that go beyond the immediately studied systems and will show trends and enable the identification of materials with statistically exceptional properties 16 . Combining data from different repositories opens further opportunities.
Let us exemplify with two emerging classes of materials that the exploitation of an efficient data infrastructure will be not only helpful but simply mandatory for the digitization of materials research 17 . These examples are high-entropy alloys (HEAs) and metal–organic frameworks (MOFs). For these classes, the sheer number of possible materials is so large that conventional approaches will never be able to unleash even a small part of their full potential. For HEAs, a number of 10 9 possible composite materials with distinctly different properties has been estimated 18 , with many of them showing, for example, mechanical properties that exceed by far those of conventional alloys. This huge space of materials further contains HEA oxides with interesting properties in catalysis and energy storage. In the case of MOFs, the situation is even more pronounced. As a result of the huge diversity of MOF building blocks, inorganic clusters and multitopic molecules, the number of compounds is unlimited. Even if one limits the building block weight to that of fullerene (C 60 ), synthesizing only one replica of each compound would already need more atoms than are available on planet Earth. Using AI to analyse the huge amount of experimental information (data for about 100,000 MOFs are stored in databases 19 ), we will be able to identify or predict MOFs with particular properties dictated by conceived applications 20 ; for example, in optoelectronics 21 , biomedicine or catalysis 22 .
Turning to the second point—to foster awareness of the importance of FAIR scientific data management and stewardship 1 —FAIRmat will reach out to current students of physics, chemistry, materials science and engineering. We aim to educate a new generation of interdisciplinary researchers, offering classes and lab courses, and to introduce new curricula. A necessary requirement is to convince teachers, professors and other decision makers. The FAIRmat consortium will initiate and organize focused, crosscutting workshops together with, for example, colleagues from chemistry and biochemistry, astroparticle and elementary particle physics, mathematics and engineering. Some topics may be general, such as ontologies or data infrastructure, whereas others will be more specific, including particular experimental techniques or specific simulation methods. Hands-on training, schools and hackathons, as well as the usual online tutorials, will be part of our portfolio. Listening to the needs of small communities or groups will make sure that no one is left behind.
Although industry is very interested in the availability of data, the materials encyclopedia and the AI tools, most investigators hesitate to contribute their own data. Understandably, a company can survive only if they create products that are better or cheaper than those of their competitors. FAIRmat accepts these worries, for example, by allowing for an embargo of uploaded data (see above). The NOMAD Oasis (see also below), which is a key element of the federated FAIRmat infrastructure, can also be operated behind industrial firewalls as a stand-alone server with full functionality.
Science is an international, open activity. So, clearly, all the concepts and plans are and will be discussed, coordinated and implemented together with our colleagues worldwide. In fact, the first FAIR-DI Conference on a FAIR Data Infrastructure for Materials Genomics had 539 participants from all over the world ( https://th.fhi-berlin.mpg.de/meetings/fairdi2020/ ).
Let us end this section by noting that individual researchers already profit from the data infrastructure, even though we are at an early stage in progressing towards the next level of research. For example, countless CPU hours are being saved because computational results are well documented and accessible and do not need to be repeated. Consequently, human time is saved as well and scientists can concentrate on new studies. Students learn faster as they can access extensive reference data. Error or uncertainty estimates are possible and more robust when using well-documented databases. Further results not documented in publications are available in the uploaded data. Studies that were designed for a specific target can now be used for a different topic (repurposing). After receiving a digital object identifier (DOI), uploaded data become citable. This also applies to analytics tools. Although the full potential of FAIRmat will require a larger community to realize and join, the spirit of findable and AI-ready research data has already attracted substantial attention.
FAIR data infrastructure for materials science
FAIRmat will build a federated infrastructure of many domain-specific data-repository solutions: as few as possible but as many as are needed. In NOMAD 6 , such individual repositories are called ‘oases’ and support the different users’ local, domain-specific, individual needs to acquire, manage and analyse their data. An oasis is a stand-alone service typically connected to a central server, called the portal, but can also be run independently. As such, it is being tested at present as a building block of FAIRmat’s federated data infrastructure.
All participating groups or institutions will manage their data using the FAIRmat frame, a common compute, management and storage concept, with a central metadata repository. To enable 4V data processes, ‘federated data with centralized metadata’ will be the general principle. Selected data may also be stored centrally, if it is functionally beneficial for users or increases the availability of high-value datasets (see Fig. 2 ).
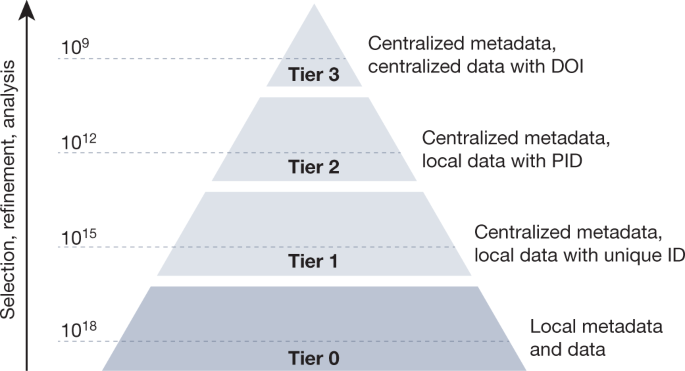
The left axis is the volume in bytes. As part of the research process, acquired data are filtered, analysed and refined. This generally produces smaller and smaller datasets of higher value. Owing to the expected data volume, the same level of availability for all data cannot be guaranteed. Therefore, data are categorized into four tiers with different levels of availability, from not shared at all (tier 0) towards published with a DOI (tier 3).
The portal will be the gateway for users to access all materials science data. Although popular search engines such as Google search for phrases in generic and mostly text-based properties (domain agnostic), we need to search for precise criteria in materials-science-specific metadata with their individual scientific notations and semantics. Thus, FAIRmat searches are domain-aware.
We will implement a common schema for all FAIRmat metadata and data. However, the data properties that are available for a given type of data differ from method to method and from domain to domain. There will be subsets of common properties for each subdomain, and these subsets form a hierarchy. For example, experiments and synthesis share a common notion of material, measurement or sample. This includes tagging samples with RFID (radio-frequency identification) or QR (quick response) code labels that are linked to every dataset acquired from them. On top of this hierarchy, and even outside the materials science domain, we will always have Dublin Core-style ( https://dublincore.org/ ) metadata about who, where and when.
This bottom-up harmonizing of metadata from different subdomains requires the development of data converters and a shared data schema. This will provide more flexibility when connecting many laboratories and new subdomains than top-down forced adoption of a new data format.
This hierarchy of common properties will also form the basis for exploring all materials science data. Similar to an online shop that allows customers to browse different categories of product, with varying criteria depending on the type of product, the central user interface will allow one to browse different subdomains of materials science on the basis of varying availabilities of data properties. On top of this, one may specify general properties, such as a material’s chemical composition and a scientific method. Then, more criteria will be made available. In this way we will design a common encyclopedia that supports the specific needs of the various materials science subdomains but will also provide more general information to non-experts.
Offering convenient tools for data analysis is an overall goal of FAIRmat. An example is the NOMAD Artificial Intelligence Toolkit ( https://nomad-lab.eu/AIToolkit ). At present, it provides several Jupyter Notebooks, some of them associated with a publication. It is recommended that researchers publish their AI analysis as well or modify or advance existing notebooks for their studies. Uploaded notebooks can obtain a DOI so that they are citable. As some data files will be huge, and may be distributed across several servers and cities, the analysis software will use the centralized metadata and extract the needed information from the (huge) data files. For the latter, we will bring the software to the data, avoiding the transfer of large files.
Other critical issues are long-term and 24/7 data availability (especially in a federated network), safety and security (especially when dealing with published versus unpublished data), data lifecycle (for example, from raw instrument readings to fully analysed and published datasets), linking data between domains, annotating data with a common user identity (for example, through ORCID; https://orcid.org ) and more.
FAIR, reproducible synthesis
Synthesizing materials with well-defined properties in a reproducible fashion is of utmost importance to materials science. Unfortunately, this desire is not always fulfilled because it requires control of a large number of experimental details, and the full entirety of the relevant parameters is typically not known. The concept of data-centric science and the development of AI tools promise to model synthesis more reliably and to identify the relevant set of descriptive parameters and their mutual interdependencies, or at least their correlations. Linking synthesis data to data from experimental materials science and theory using common metadata schemas and ontologies will create a new level of the science of materials synthesis.
Publicly accessible databases such as Landolt-Börnstein/Springer Materials ( https://materials.springer.com ) and the Inorganic Crystal Structure Database ( https://icsd.products.fiz-karlsruhe.de ) contain huge numbers of entries on the properties of crystalline materials but they lack information on their synthesis. Recently, work on the basis of machine-learning and natural-language-processing techniques has started to codify materials synthesis conditions and parameters that are published in journal articles 23 . The auto-generated open-source dataset at Berkeley ( https://ceder.berkeley.edu/text-mined-synthesis ) consisted of 19,744 chemical reactions retrieved from 53,538 solid-state synthesis paragraphs by March 2021. However, typically, this information is incomplete, and published information is biased towards reports of successful studies, omitting failed attempts.
This unsatisfactory situation is rooted in the complexity of the synthesis processes, including elaborate workflows and a large diversity of instruments for characterization. In the realm of FAIRmat, we follow the relevant phase transformations that occur during synthesis from the melt, from the gas phase, from solid phases and from solution. Synthesis by assembly complements these classical approaches. The nature of the assembly method is very different, as collective behaviour gives rise to new properties, such as the formation of aggregates or self-assembly. Figure 3 depicts the variety of crystal growth methods. Even though Czochralski, Bridgman, metal flux growth and optical floating zone are all melt-growth techniques—that is, they belong to the same type of phase transition—they are distinguished by the contact of the melt with the crucible, by the seeding of the single crystal and by thermal gradients, with great influence on crystallinity and impurity content. But even fine details matter. For example, the geometry of the reactors, fluctuations in the impurity content of the source material, the flow of precursors in the reactor or the miscut and pretreatment of substrates in epitaxial growth may have detrimental effects. At this point, synthesis is often based on experience and tricks, which are not readily shared with others. Obviously, this makes the development of metadata schemas and ontologies a formidable task and—with respect to the four Vs—synthesis struggles mainly with variety and veracity.
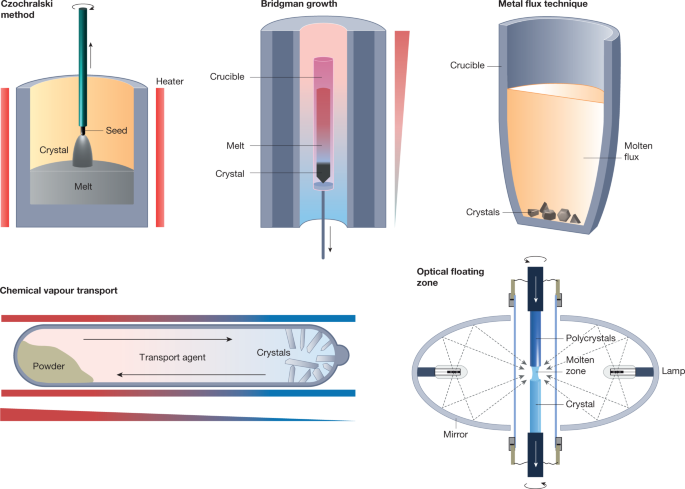
Simplified sketches of the five most common crystal growth methods from the melt (Czochralski, Bridgman, optical floating zone and metal flux growth) and the gas phase (chemical vapour transport).
We started to establish metadata and ontologies following the above-mentioned phase transformations. To connect to the other experimental disciplines (for example, sample characterization) we aim at a common ELN scheme and laboratory information management system (LIMS) and uniquely identify the samples, as noted in the infrastructure section. Thereby, we link the measured physical and chemical properties of a specimen to the synthesis workflow. The ELN and LIMS data are automatically fed into a prototype repository that is now being developed at the Leibniz Institute for Crystal Growth ( https://ikz-berlin.de/ ).
Once a structured database on synthesis has been established, this will allow computer-aided development of synthesis recipes to fabricate as yet unknown materials with tailored properties. Moreover, it will enable comparison of different synthesis methods for the same material in terms of generalized physical and chemical parameters, also linking them to theoretical predictions.
FAIR data in experimental disciplines
Experimental materials science is concerned with the characterization of the atomic and electronic structures of compounds, as well as with determining their electrical, optical, magnetic, thermal or mechanical properties. Typically, terabytes (sometimes petabytes) of data from one study result in a few plots in a publication. Only FAIR data management of all results, both successful and failed, makes experimental studies reproducible and obviates the necessity to repeat the experiments for a different but related project. In addition, by making all these data available to the community, everyone will benefit from statistically more reliable quantification of measurement errors and calibrations.
In experimental materials science, the variety of characterization methods is very diverse, and each class of methods has its own equipment and workflows for generating data. The diversity in data formats specific to vendors, labs, instruments, communities and operators presents a substantial challenge with regard to integrating this information into a FAIR infrastructure. For the initial period, we concentrate on five experimental techniques (see Fig. 4 ) with very different frontiers in terms of the 4V challenges, and largely disjunct and differently structured communities. These are electron microscopy and spectroscopy, angle-resolved photoemission spectroscopy, core-level photoemission spectroscopy, optical spectroscopy and atom-probe tomography. The amount of generated data ranges from a few kilobytes to terabytes per dataset, and the data rates and data structures also differ substantially. With some modern detectors delivering several gigabytes of data per second, the volume and velocity challenge is to preprocess, compress and evaluate or visualize these data. This becomes a more severe velocity issue in time-resolved experiments, for which the duration may not even be fixed but being decided during the observation. Disturbingly, overall, we observe a lack of efficient and reliabe recording of metadata in a digital form, posing a severe data-veracity challenge.
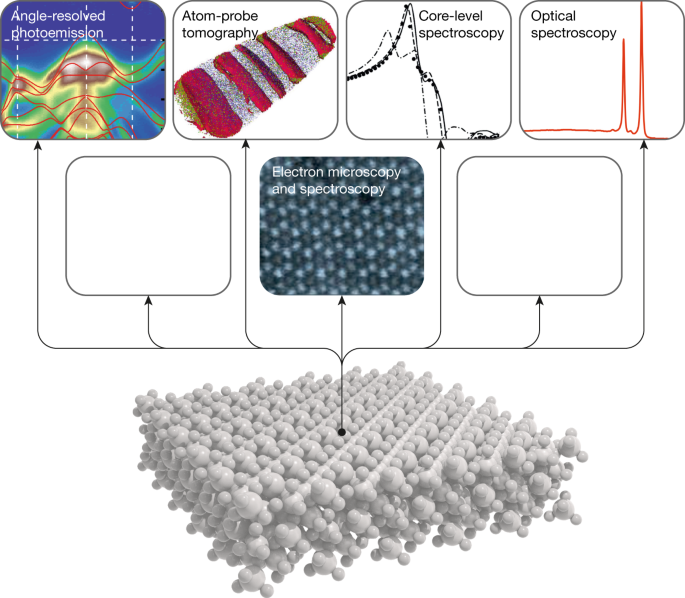
Optical spectroscopy, atom-probe tomography, angle-resolved photoelectron spectroscopy, electron microscopy and X-ray photoemission spectroscopy are noted explicitly, and the large number of other experimental methods is indicated by the empty ‘perspectives’.
Analogously to establishing FAIR data in synthesis, a strong focus is the customization of inter-operating ELNs and LIMSs, their integration into experimental workflows and their direct connection to the data repository.
In each of the five selected experimental techniques, activities have also started to define domain-specific metadata catalogues and ontologies. In some labs (transmission electron microscopy and spectroscopy), a first, rudimentary prototype of a NOMAD Oasis has recently been installed, with the aim of exploring how it should be further developed towards the requirements of the different subdomains. Integration of ELNs into the experimental workflow is at different stages of development, ranging from first implementation concepts in atom-probe tomography to a working integration of an ELN database with the data acquisition software in some transmission electron microscopy labs. This also includes the tagging of samples with QR labels and automated linking of sample IDs with links to experimental data and time-stamped notes generated by the data acquisition software. Several angle-resolved photoemission spectroscopy groups are reorganizing their labs at present, switching from paper lab books to ELNs. In this context, we note that, in a joint effort of different labs, we were able to make vendors of complex equipment reconsider their previously restrictive and closed data-format policies.
FAIR theory and computations
Materials modelling, in particular, including digital twins, is enjoying ever-growing attention thanks to a timely combination of hardware and algorithmic developments 24 . The NOMAD Laboratory 6 has already implemented a materials data infrastructure for quantum-mechanical ground-state calculations and ab initio molecular dynamics (see the summary in the section on ‘Data-centric materials science’). However, materials modelling also requires force fields and particle-based methods, to capture larger length scales and longer timescales (see Fig. 5 ). The implementation of such multiscale materials data infrastructure faces several outstanding challenges 25 , 26 . By considering trajectories, we need to account for both instantaneous and ensemble properties. Also, the heterogeneity of simulation setups, solvers, force fields and observables requires an ambitious and coherent strategy to make multiscale modelling FAIR. The development of metadata for this field has only just started.
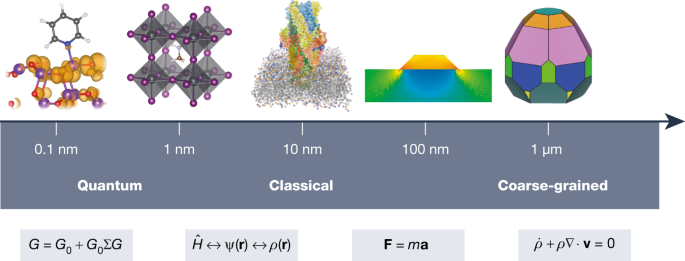
Approaches range from excited states, ground-state density functional theory and force-field-based molecular dynamics to continuum. The data infrastructure has to meet the needs of the different methods. These are outlined by the equations at the bottom.
Another crucial task is the response of matter to external stimuli. The physical objects of interest obtained from theory are excitation energies and lifetimes, electronic band gaps, dielectric tensors, various excitation spectra and ionization potentials, all of which have experimental counterparts. The leading methodologies 27 comprise time-dependent density functional theory, Green function techniques and dynamical mean-field theory, implemented in a huge number of different computer codes. The predicted FAIRmat infrastructure will foster the often incomplete documentation at present and facilitate benchmarking and curation of results.
Concerning the four Vs, the area of theory and computation is severely affected by variety (that is, the heterogeneity of the meaning of the produced data). This refers to the fact that there are many physical equations, even more algorithms and yet more approximations that are implemented in the numerous, very different software packages. Although ab initio computational materials science has largely assumed a common nomenclature, for example, for the several hundred exchange-correlation approximations (see, for example, Libxc, a library of exchange-correlation and kinetic energy functionals for density functional theory; https://tddft.org/programs/libxc/ ), this is not yet the case for force fields, dynamical mean-field theory or calculations of fluid dynamics, and so on.
Related to the variety challenge is veracity. Note that we differentiate between accuracy and precision; the latter can be checked by comparing results from different software that addresses the same equations and uses the same approximations. Although for ab initio computational materials science the first important steps have been made 28 , for other theoretical approaches, such efforts are still missing. Accuracy, in turn, refers to the equations and basic approximations (for example, the exchange-correlation functional used or the force field). Here, error bars are largely missing so far, but for interoperability with experimental results, such error estimates need to be developed. Concerning the data volume, for molecular dynamics calculations, it is hardly possible to store all the information—that is, the detailed time evolution of the positions of all the atoms (if these are several thousand in number, as in force field studies) or the electronic charge density (in ab initio studies). Here, selection and compression strategies will be developed.
Making the data revolution happen
Fourteen years after Jim Gray’s explication on data-intensive scientific discovery 29 , materials science is still dominated by the first three research paradigms: experiment, theory and numerical simulations 2 . However, there is now wide consensus that data-centric research and the fourth paradigm (data mining, new ways of analysis (largely by AI) and visualization) will change, if not revolutionize, the sciences. We stress that the fourth paradigm represents a new way of thinking 2 . It complements but does not replace the previous concepts and approaches. Implementation of this paradigm not only creates new opportunities but also enhances the traditional approaches through efficient data exchange, better documentation and a more detailed understanding of what other groups are doing. This will open new horizons for research in the basic and engineering sciences, reaching out to industry and society.
So, let us summarize what we need to make the data revolution happen in materials science:
Hardware for data storage and handling, advanced analytics and high-speed networks. The availability of appropriate hardware is the basic prerequisite for building the described data infrastructure. We also need middleware, for example, for the efficient exchange of data that are created in or by different digital environments. In addition, efficient, near-real-time data analytics will also require advanced hardware, as well as software and hardware co-design.
Development and support of software tools. New tools are already being invented; for example, for fitting data, removing noise from data, learning rules that are behind patterns in data and identifying ‘statistically exceptional’ data groups 16 . With such rules, one will also identify ‘materials genes’—physical parameters that are related to the processes that trigger, facilitate or hinder a certain materials property or function. FAIRmat will foster the international coordination of the development of such tools in the wider materials science community.
The development of ELNs and LIMSs. Such necessary changes of current scientific procedures seem minor if one accepts that it is good scientific practice to document the experimental (or computational) conditions and the results in full detail, so that studies are reproducible. Thus, data collection (including the comprehensive characterization of the experimental setup) should become as automatic as possible. This sounds like an outdated request, but it has not been executed properly so far and, for data-centric science, it is essential. Unfortunately, for some—maybe many—studies, an immediate realization is not fully possible and even the first approximation requires a ‘phase transition’. Owing to the complexity of the field, there is no one-size-fits-all solution.
Close collaboration between experts from data science, IT infrastructure, software engineering and the materials science domain as equal partners. In FAIRmat, this will be realized by a centralized hub of specialists at the Physics Department of the Humboldt-Universität in Berlin.
Changing the publication culture and advancing digital libraries. As noted above, the basic scientific requirement of reproducibility of experimental work is often lacking. This is rooted in the complexity and intricacy of materials synthesis. FAIRmat will change this situation. The concept of ‘clean data’—that is, data that are comprehensively annotated—is being developed (see ref. 30 and references therein). This is much more elaborate than it sounds, and publications that ‘just’ present and describe such data comprehensively should be appreciated by the community as much as a standard publication in a high-impact journal.
Digital libraries have been built and advanced over the past decade, and this work continues. Although there have been ample developments in the field of life sciences, the situation in materials science is less advanced. However, it is improving (for example, at https://tib.eu/ or https://openaire.eu/ ) and, in this field, metadata catalogues are typically too unspecific to allow the identification of suitable datasets (for example, for AI analysis).
The German National Research Data Infrastructure project ( https://nfdi.de ) promotes all of the points discussed above, with the exception of the necessary hardware. Although a national effort, it is obviously part of an international activity, and FAIRmat has established respective collaborations already. We will support scientists and confirm them in their responsible handling of research data, and we will strive to educate the next generation of researchers and engineers to actively engage in order to achieve these goals in a timely manner.
The field is changing and the research community seems mostly convinced about the direction of this change, but it is still mostly in the role of an observer. If active scientists don’t sign on, the infrastructure will develop without them. Then, in a few years, they will need to accept what is there, and it may—unfortunately—not fully serve their needs. The consequences of the whole endeavour may be summarized as follows: the predicted changes brought about by a FAIR data infrastructure will not replace scientists, but scientists who use such an infrastructure may replace those who don’t.
Wilkinson, M. D. et al. The FAIR Guiding Principles for scientific data management and stewardship. Sci. Data 3 , 160018 (2016). This work coined the acronym FAIR, which is now used worldwide .
Article Google Scholar
Draxl, C. & Scheffler, M. In Handbook of Materials Modeling (eds Andreoni, W. & Yip, S.) 49–73 (Springer, 2020). Addresses the fourth paradigm of materials research and highlights the related challenges .
Editorial. Empty rhetoric over data sharing slows science. Nature 546 , 327 (2017).
Google Scholar
Draxl, C., Illas, F. & Scheffler, M. Open data settled in materials theory. Nature 548 , 523 (2017).
Article ADS CAS Google Scholar
Himanen, L., Geurts, A., Foster, A. S. & Rinke, P. Data-driven materials science: status, challenges, and perspectives. Adv. Sci. 6 , 1900808 (2019).
Draxl, C. & Scheffler, M. The NOMAD laboratory: from data sharing to artificial intelligence. J. Phys. Mater. 2 , 036001 (2019).
Article CAS Google Scholar
Ghiringhelli, L. M. et al. Towards efficient data exchange and sharing for big-data driven materials science: metadata and data formats. NPJ Comput. Mater. 3 , 46 (2017).
Article ADS Google Scholar
Talriz, L. et al. Materials Cloud, a platform for open computational science. Sci. Data 7 , 299 (2020).
Andersen, C. W. et al. OPTIMADE, an API for exchanging materials data. Sci. Data 8 , 217 (2021).
Wittenburg, P., Lautenschlager, M., Thiemann, H., Baldauf, C. & Trilsbeek, P. FAIR practices in Europe. Data Intell. 2 , 257–263 (2020).
Tanaka, I., Rajan, K. & Wolverton, C. Data-centric science for materials innovation. MRS Bull. 43 , 659–663 (2018). Describes the status of data-centric research in materials science .
Ghiringhelli, L. M., Vybiral, J., Levchenko, S. V., Draxl, C. & Scheffler, M. Big data of materials science: critical role of the descriptor. Phys. Rev. Lett. 114 , 105503 (2015).
Isayev, O. et al. Universal fragment descriptors for predicting properties of inorganic crystals. Nat. Commun. 8 , 15679 (2017).
Jäckle, M., Helmbrecht, K., Smits, M., Stottmeister, D. & Groß, A. Self-diffusion barriers: possible descriptors for dendrite growth in batteries? Energy Environ. Sci. 11 , 3400–3407 (2018).
Ward, L., Agrawal, A., Choudhary, A. & Wolverton, C. A general-purpose machine learning framework for predicting properties of inorganic materials. NPJ Comput. Mater. 2 , 16028 (2016).
Kulik, H. et al. Roadmap on machine learning in electronic structure. Electron. Struct. https://doi.org/10.1088/2516-1075/ac572f Section 1.4 (2022).
Kraft, O., Wenzel, W. & Wöll, C. Materials research in the information age. Adv. Mater. 31 , 1902591 (2019).
Cantor, B. Multicomponent and high entropy alloys. Entropy 16 , 4749 (2014).
Chung, Y. G. et al. Advances, updates, and analytics for the computation-ready, experimental metal–organic framework database: CoRE MOF 2019. J. Chem. Eng. Data 64 , 5985–5998 (2019).
Ahmad, M., Luo, Y., Wöll, C., Tsotsalas, M. & Schug, A. Design of metal-organic framework templated materials using high-throughput computational screening. Molecules 25 , 4875 (2020).
Haldar, R. et al. A de novo strategy for predictive crystal engineering to tune excitonic coupling. Nat. Commun. 10 , 2048 (2019).
Rosen, A. S., Notestein, J. M. & Snurr, R. Q. Structure–activity relationships that identify metal–organic framework catalysts for methane activation. ACS Catal. 9 , 3576–3587 (2019).
Kim, E. et al. Materials synthesis insights from scientific literature via text extraction and machine learning. Chem. Mater. 29 , 9436–9444 (2017).
Ceder, G. & Persson, K. The stuff of dreams. Sci. Am. 309 , 36–40 (2013).
Bereau, T. Computational compound screening of biomolecules and soft materials by molecular simulations. Model. Simul. Mater. Sci. Eng. 29 , 023001 (2021).
Jackson, N. E., Webb, M. A. & de Pablo, J. J. Recent advances in machine learning towards multiscale soft materials design. Curr. Opin. Chem. Eng. 23 , 106–114 (2019).
Martin, R. M., Reining, L. & Ceperley, D. M. Interacting Electrons. Theory and Computational Approaches 1st edn (Cambridge Univ. Press, 2016).
Lejaeghere, K. et al. Reproducibility in density-functional theory calculations of solids. Science 351 , aad3000 (2016).
Hey, T., Tansley, S. & Tolle, K. (eds) The Fourth Paradigm: Data-Intensive Discovery (Microsoft, 2009).
Trunschke, A. et al. Towards experimental handbooks in catalysis. Top. Catal. 63 , 1683–1699 (2020).
Download references
Acknowledgements
This work received funding from the Max Planck Research Network BiGmax, the Humboldt-Universität zu Berlin and the European Union’s Horizon 2020 research and innovation programme under grant agreement no. 951786, the NOMAD CoE. We thank S. Auer for his feedback on digital libraries, V. Coors for her thoughtful work on the figures and the entire FAIRmat team for shaping the concept and first steps towards its implementation. FAIRmat is funded by the Deutsche Forschungsgemeinschaft (DFG, German Research Foundation), project 460197019.
Author information
Authors and affiliations.
Physics Department and IRIS Adlershof, Humboldt-Universität zu Berlin, Berlin, Germany
Matthias Scheffler, Christoph T. Koch, Markus Scheidgen & Claudia Draxl
The NOMAD Laboratory at the Fritz Haber Institute of the Max Planck Society, Berlin, Germany
Matthias Scheffler & Claudia Draxl
Department of Physics and Research Center OPTIMAS, University of Kaiserslautern, Kaiserslautern, Germany
Martin Aeschlimann
Leibniz-Institut für Kristallzüchtung, Berlin, Germany
Martin Albrecht
Max-Planck-Institut für Polymerforschung, Mainz, Germany
Tristan Bereau & Kurt Kremer
Department of Informatics, Technical University of Munich, Munich, Germany
Hans-Joachim Bungartz
Max Planck Institute for Chemical Physics of Solids, Dresden, Germany
Claudia Felser
Max Planck Institute for Chemical Energy Conversion, Mülheim an der Ruhr, Germany
Mark Greiner
Institute of Theoretical Chemistry, Ulm University and Helmholtz-Institute Ulm, Ulm, Germany
Computer Science Department, Technical University Dresden, Dresden, Germany
Wolfgang E. Nagel
Institute of Functional Interfaces, Karlsruhe Institute of Technology, Karlsruhe, Germany
Christof Wöll
You can also search for this author in PubMed Google Scholar
Contributions
C.D. (chairperson of FAIRmat) and M. Scheffler (co-chair) created the general concept, led the writing and edited the whole paper, in close discussion with all the other authors. They also wrote the abstract, introduction, ‘Data-centric materials science’ and ‘Making the data revolution happen’ sections. M. Albrecht and C.F. took the lead on the section ‘FAIR, reproducible synthesis’ (Area A of FAIRmat); M.G. and C.T.K. on the section ‘FAIR data in experimental disciplines’ (Area B of FAIRmat); M. Scheffler., K.K. and T.B. on the section ‘FAIR theory and computations’ (Area C of FAIRmat); and H.-J.B., M. Scheidgen and W.E.N. on the section ‘FAIR data infrastructure for materials science’ (Area D of FAIRmat). C.D., together with C.W. and A.G. (Area E of FAIRmat) and M. Scheffler and M. Aeschlimann (Area F of FAIRmat), created the section ‘Preparing the research of tomorrow’. All authors carefully read and edited the whole paper.
Corresponding author
Correspondence to Claudia Draxl .
Ethics declarations
Competing interests.
The authors declare no competing interests.
Peer review
Peer review information.
Nature thanks Kerstin Kleese van Dam, Brian Matthews and the other, anonymous, reviewers for their contribution to the peer review of this work.
Additional information
Publisher’s note Springer Nature remains neutral with regard to jurisdictional claims in published maps and institutional affiliations.
Rights and permissions
Reprints and permissions
About this article
Cite this article.
Scheffler, M., Aeschlimann, M., Albrecht, M. et al. FAIR data enabling new horizons for materials research. Nature 604 , 635–642 (2022). https://doi.org/10.1038/s41586-022-04501-x
Download citation
Received : 08 March 2021
Accepted : 28 January 2022
Published : 27 April 2022
Issue Date : 28 April 2022
DOI : https://doi.org/10.1038/s41586-022-04501-x
Share this article
Anyone you share the following link with will be able to read this content:
Sorry, a shareable link is not currently available for this article.
Provided by the Springer Nature SharedIt content-sharing initiative
This article is cited by
Semantics-enabled data federation: bringing materials scientists closer to fair data.
- Kareem S. Aggour
- Vijay S. Kumar
- Varish Mulwad
Integrating Materials and Manufacturing Innovation (2024)
Language models and protocol standardization guidelines for accelerating synthesis planning in heterogeneous catalysis
- Manu Suvarna
- Alain Claude Vaucher
- Javier Pérez-Ramírez
Nature Communications (2023)
3DSC - a dataset of superconductors including crystal structures
- Timo Sommer
- Roland Willa
- Pascal Friederich
Scientific Data (2023)
Designing active oxides for a durable oxygen evolution reaction
- Sebastian Z. Oener
- Arno Bergmann
- Beatriz Roldan Cuenya
Nature Synthesis (2023)
Fatigue database of complex metallic alloys
- Haoxuan Tang
By submitting a comment you agree to abide by our Terms and Community Guidelines . If you find something abusive or that does not comply with our terms or guidelines please flag it as inappropriate.
Quick links
- Explore articles by subject
- Guide to authors
- Editorial policies
Sign up for the Nature Briefing newsletter — what matters in science, free to your inbox daily.

- Our Program Divisions
- Our Three Academies
- Government Affairs
- Statement on Diversity and Inclusion
- Our Study Process
- Conflict of Interest Policies and Procedures
- Project Comments and Information
- Read Our Expert Reports and Published Proceedings
- Explore PNAS, the Flagship Scientific Journal of NAS
- Access Transportation Research Board Publications
- Coronavirus Disease 2019 (COVID-19)
- Diversity, Equity, and Inclusion
- Economic Recovery
- Fellowships and Grants
- Publications by Division
- Division of Behavioral and Social Sciences and Education
- Division on Earth and Life Studies
- Division on Engineering and Physical Sciences
- Gulf Research Program
- Health and Medicine Division
- Policy and Global Affairs Division
- Transportation Research Board
- National Academy of Sciences
- National Academy of Engineering
- National Academy of Medicine
- Publications by Topic
- Agriculture
- Behavioral and Social Sciences
- Biography and Autobiography
- Biology and Life Sciences
- Computers and Information Technology
- Conflict and Security Issues
- Earth Sciences
- Energy and Energy Conservation
- Engineering and Technology
- Environment and Environmental Studies
- Food and Nutrition
- Health and Medicine
- Industry and Labor
- Math, Chemistry, and Physics
- Policy for Science and Technology
- Space and Aeronautics
- Surveys and Statistics
- Transportation and Infrastructure
- Searchable Collections
- New Releases
VIEW LARGER COVER
New Worlds, New Horizons in Astronomy and Astrophysics
Driven by discoveries, and enabled by leaps in technology and imagination, our understanding of the universe has changed dramatically during the course of the last few decades. The fields of astronomy and astrophysics are making new connections to physics, chemistry, biology, and computer science. Based on a broad and comprehensive survey of scientific opportunities, infrastructure, and organization in a national and international context, New Worlds, New Horizons in Astronomy and Astrophysics outlines a plan for ground- and space- based astronomy and astrophysics for the decade of the 2010's.
Realizing these scientific opportunities is contingent upon maintaining and strengthening the foundations of the research enterprise including technological development, theory, computation and data handling, laboratory experiments, and human resources. New Worlds, New Horizons in Astronomy and Astrophysics proposes enhancing innovative but moderate-cost programs in space and on the ground that will enable the community to respond rapidly and flexibly to new scientific discoveries. The book recommends beginning construction on survey telescopes in space and on the ground to investigate the nature of dark energy, as well as the next generation of large ground-based giant optical telescopes and a new class of space-based gravitational observatory to observe the merging of distant black holes and precisely test theories of gravity.
New Worlds, New Horizons in Astronomy and Astrophysics recommends a balanced and executable program that will support research surrounding the most profound questions about the cosmos. The discoveries ahead will facilitate the search for habitable planets, shed light on dark energy and dark matter, and aid our understanding of the history of the universe and how the earliest stars and galaxies formed. The book is a useful resource for agencies supporting the field of astronomy and astrophysics, the Congressional committees with jurisdiction over those agencies, the scientific community, and the public.
RESOURCES AT A GLANCE
- Press Release
- Report Brief
- 2020 VISION PDF Booklet (36 Pages)
- Space and Aeronautics — Astronomy and Astrophysics
- Space and Aeronautics — Space Exploration and Development
Suggested Citation
National Research Council. 2010. New Worlds, New Horizons in Astronomy and Astrophysics . Washington, DC: The National Academies Press. https://doi.org/10.17226/12951. Import this citation to: Bibtex EndNote Reference Manager
Publication Info
- Paperback: 978-0-309-15799-5
- Hardcover: 978-0-309-15802-2
- Ebook: 978-0-309-18685-8
What is skim?
The Chapter Skim search tool presents what we've algorithmically identified as the most significant single chunk of text within every page in the chapter. You may select key terms to highlight them within pages of each chapter.
- 2020 VISION PDF Booklet (36 Pages) Read Description: This booklet provides an overview of the sixth, most recent, National Research Council decadal survey of astronomy and astrophysics, New Worlds, New Horizons in Astronomy and Astrophysics.
Copyright Information
The National Academies Press (NAP) has partnered with Copyright Clearance Center's Marketplace service to offer you a variety of options for reusing NAP content. Through Marketplace, you may request permission to reprint NAP content in another publication, course pack, secure website, or other media. Marketplace allows you to instantly obtain permission, pay related fees, and print a license directly from the NAP website. The complete terms and conditions of your reuse license can be found in the license agreement that will be made available to you during the online order process. To request permission through Marketplace you are required to create an account by filling out a simple online form. The following list describes license reuses offered by the NAP through Marketplace:
- Republish text, tables, figures, or images in print
- Post on a secure Intranet/Extranet website
- Use in a PowerPoint Presentation
- Distribute via CD-ROM
Click here to obtain permission for the above reuses. If you have questions or comments concerning the Marketplace service, please contact:
Marketplace Support International +1.978.646.2600 US Toll Free +1.855.239.3415 E-mail: [email protected] marketplace.copyright.com
To request permission to distribute a PDF, please contact our Customer Service Department at [email protected] .
What is a prepublication?

An uncorrected copy, or prepublication, is an uncorrected proof of the book. We publish prepublications to facilitate timely access to the committee's findings.
What happens when I pre-order?
The final version of this book has not been published yet. You can pre-order a copy of the book and we will send it to you when it becomes available. We will not charge you for the book until it ships. Pricing for a pre-ordered book is estimated and subject to change. All backorders will be released at the final established price. As a courtesy, if the price increases by more than $3.00 we will notify you. If the price decreases, we will simply charge the lower price. Applicable discounts will be extended.
Downloading and Using eBooks from NAP
What is an ebook.
An ebook is one of two file formats that are intended to be used with e-reader devices and apps such as Amazon Kindle or Apple iBooks.
Why is an eBook better than a PDF?
A PDF is a digital representation of the print book, so while it can be loaded into most e-reader programs, it doesn't allow for resizable text or advanced, interactive functionality. The eBook is optimized for e-reader devices and apps, which means that it offers a much better digital reading experience than a PDF, including resizable text and interactive features (when available).
Where do I get eBook files?
eBook files are now available for a large number of reports on the NAP.edu website. If an eBook is available, you'll see the option to purchase it on the book page.
View more FAQ's about Ebooks
Types of Publications
Consensus Study Report: Consensus Study Reports published by the National Academies of Sciences, Engineering, and Medicine document the evidence-based consensus on the study’s statement of task by an authoring committee of experts. Reports typically include findings, conclusions, and recommendations based on information gathered by the committee and the committee’s deliberations. Each report has been subjected to a rigorous and independent peer-review process and it represents the position of the National Academies on the statement of task.
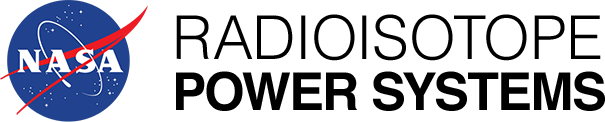
News | July 14, 2022
Great exploration revisited: new horizons at pluto and charon.
July 14, 2022 -- Seven years ago today, NASA's New Horizons spacecraft made history with the first up-close exploration of the Pluto system – providing breathtaking views and detailed data on Pluto and its largest moon, Charon, revealing the surfaces of these distant, mysterious worlds at the outer reaches of our solar system.
These simulated flights over Pluto and Charon include some of the sharpest images and topographic data that New Horizons gathered during its historic flyby on July 14, 2015. These are the first “movies" of Pluto and Charon made from the highest-resolution black-and-white image strips, taken by New Horizons' Long Range Reconnaissance Imager (LORRI), as the spacecraft zipped by at more than 30,000 miles per hour.
“These new high-resolution flyover videos are incredible," said New Horizons Principal Investigator Alan Stern, of the Southwest Research Institute. “They aren't just scientifically valuable, but they are also engaging, which is why we want to share them with the public. Enjoy flying over a planet named Pluto and its giant moon Charon, both more than three billion miles from Earth!"
“These new high-resolution flyover videos are incredible. They aren't just scientifically valuable, but they are also engaging, which is why we want to share them with the public." - Alan Stern, New Horizons Principal Investigator
Features as small as about 230 feet (70 meters) are visible on Pluto's icy, rocky surface. Moviemaker and New Horizons science team member Paul Schenk, from the Lunar and Planetary Institute, used high-resolution topographic mapping analysis to show surface relief in the nitrogen-laden ice sheet in the Sputnik Planitia impact basin – half of Pluto's famous “heart" feature.
This simulated flight starts near the center of the ice sheet and ends on the rugged ice-carved southeastern rim of the basin 300 miles (500 kilometers) away, where the difference between the highest and lowest points is more than 2 miles (3.5 kilometers). Also prominently visible are the small pits that cover the surface of the otherwise low-relief ice sheet. Schenk also added color data from New Horizons' Multispectral Visible Imaging Camera (MVIC) to bring out the reddish hues in Pluto's highlands.
The simulated Charon flyover starts in the low-lying, icy volcanic plains of Vulcan Planitia and ends in fractured northern plains some 300 miles (500 kilometers) away. Prominently visible are several mountains that rise about 1.5-2.5 miles (3-4 kilometers) above the volcanic plains. The images in this narrow strip show surface details as small as about 450 feet (140 meters) across.
The Johns Hopkins Applied Physics Laboratory in Laurel, Maryland, designed, built and operates the New Horizons spacecraft, and manages the mission for NASA's Science Mission Directorate. Southwest Research Institute, in San Antonio and Boulder, Colorado, directs the mission via Principal Investigator Stern, and leads the science team, payload operations and encounter science planning. New Horizons is part of the New Frontiers Program managed by NASA's Marshall Space Flight Center in Huntsville, Alabama. The Lunar and Planetary Institute in Houston is operated by USRA under a cooperative agreement with NASA's Science Mission Directorate.
- Story by the New Horizons team, Johns Hopkins University Applied Physics Laboratory
You Might Also Like


An official website of the United States government
The .gov means it's official. Federal government websites often end in .gov or .mil. Before sharing sensitive information, make sure you're on a federal government site.
The site is secure. The https:// ensures that you are connecting to the official website and that any information you provide is encrypted and transmitted securely.
- Publications
- Account settings
- Browse Titles
NCBI Bookshelf. A service of the National Library of Medicine, National Institutes of Health.
National Research Council (US) Committee on Future Directions for Behavioral and Social Sciences Research at the National Institutes of Health; Singer BH, Ryff CD, editors. New Horizons in Health: An Integrative Approach. Washington (DC): National Academies Press (US); 2001.

New Horizons in Health: An Integrative Approach.
- Hardcopy Version at National Academies Press
1 Introduction
H istorically speaking, human health has been a tale of ever-shifting horizons. For much of the distant past, health was equivalent to short-term survival in the face of food scarcity, predators, and pestilence. With gains in agriculture, sanitation, and the growth of community, length of life was extended somewhat. Later, the scientific revolution transformed health into a biological realm that was primarily the purview of medical fields, at least in Western and economically developed cultures. The past century has witnessed dramatic gains in longevity, thanks to unprecedented advances in diagnosing, treating, and preventing disease, along with unimaginable gains in technology that facilitate understanding health at molecular, cellular, and genetic levels. Still, there remains significant distance to travel in the journey toward optimal human health. The horizon before us is one in which health encompasses not only the workings of biology, the brain, and the body but also the human mind, its thoughts and feelings, human actions and behavior, as well as the nature of social ties, friendships, family, and community life.
With this vision before us, we call for a new era of research and practice at the National Institutes of Health (NIH) that integrates biomedical and social behavioral fields of inquiry to promote the nation's health. Increasing evidence documents the role of behavioral, psychological, social, and environmental factors as causes of death. In a widely cited paper, McGinnis and Foege (1993) showed that unhealthy behaviors and environmental exposures were the “actual causes of death” that accounted for 50 percent of all U.S. mortality. Moreover, modern scientific tools afford far-reaching opportunities for unraveling mechanistic processes (e.g., environmentally induced gene expression, overload of physiological systems) through which behavioral and psychosocial factors contribute to illness and disease. In counterpoint to such maladaptive biobehavioral interactions, people's positive daily practices (e.g., getting proper nutrition, engaging in physical activity, avoiding cigarettes, alcohol, and drugs), along with the quality of their social relationships, psychological outlooks, and community supports are emerging as key ingredients to health and well-being over the life course. In short, the behavioral and social sciences are unavoidably implicated in making sense of both illness and good health, although much as yet is unknown about how these effects occur, particularly in terms of what can be done to avoid their deleterious impacts as well as promote their salubrious benefits for ever larger segments of the population.
This chapter sets the stage for the proposed new era of integrative health research. We first briefly review the history of the behavioral and social sciences at NIH and then describe the specific charge to the committee and our interpretation of this charge.
The integrative approach to health is the overarching theme of this report. In support of it, we revisit the distinguished intellectual history of those who have called for such bringing together of multiple levels of analysis. In numerous corners of science, the need to embark on new inquiries that put the disciplines together is a growing refrain. This synthesis—the move toward “consilience” ( Wilson, 1998 )—is particularly essential if we are to achieve comprehensive understanding of how good health at the level of the individual and of society is realized or lost. Much has been learned, and will continue to be gained, by focusing on single diseases and single mechanistic processes, but we bring into high relief the reality that many illnesses co-occur, as do many risk factors (behavioral and biological). What is needed, thus, are new studies that delineate the biopsychosocial pathways through which converging processes contribute to diverse health outcomes.
Each of the following chapters is broad and integrative in scope. Collectively, the chapters comprise key elements required for integration, from molecular, cellular, and genetic levels through behavioral, psychological, social, and environmental levels to multiple health outcomes. Stated otherwise, the chapters embody what the committee deemed critical influences that are essential to understanding the pathways to health.
- THE CONTEXT: BEHAVIORAL AND SOCIAL SCIENCES AT THE NATIONAL INSTITUTES OF HEALTH
The behavioral and social sciences are increasingly recognized as vital contributors to understanding and improving the nation's health. In this regard, it is important to note the long history of behavioral and social science research at NIH. For example, the National Heart Institute, predecessor of the National Heart, Lung, and Blood Institute, was founded in 1948 and funded its first behavioral science research grant in 1955. The study was focused on psychological factors related to high blood pressure and coronary heart disease. The National Cancer Institute, established in 1937, also has an extensive behavioral research program emphasizing cancer prevention and control. The historical roots of this broad agenda reside in the mandate of the National Cancer Act, passed by Congress in 1971. More generally, many of the institutes have longstanding and well-developed behavioral and social science programs. Trans-institute initiatives with linkages to basic biology are also appearing with increasing frequency, such as the recent call for proposals on socioeconomic status and health as well as the recent establishment of five new mind/body centers around the country.
At the same time, the behavioral and social sciences have limited presence at some institutes or are seen as peripheral to primary agendas. Also, when considered at all, behavioral, psychological, and social priorities are sometimes restricted to a narrow focus on their role as risk factors for particular disease outcomes. To facilitate the growth and development of these important fields, Congress established the Office of Behavioral and Social Sciences Research (OBSSR) at NIH in 1995. A central message of OBSSR, and the background for this report, is that behavioral, psychosocial, and environmental factors have broad significance at NIH and are fundamental to comprehensive understanding of diverse disease etiologies as well as to positive health promotion.
- THE CHARGE TO THE COMMITTEE
In 1999 the director of OBSSR requested assistance from the National Research Council (NRC) to develop a research plan to guide NIH in supporting areas of high priority in the social and behavioral sciences. Three principal goals shaped the OBSSR planning efforts: (1) enhancement of behavioral and social sciences research and training, (2) integration of biobehavioral interdisciplinary perspectives across NIH, and (3) improvement of communication between those conducting scientific research and the general public. The OBSSR sought to use the priorities requested from the NRC as a framework within which to implement these goals.
Within the NRC and its Commission on Behavioral and Social Sciences and Education (CBASSE), the Board on Behavioral, Cognitive, and Sensory Sciences chose to undertake a brief, highly focused study in response to the OBSSR request. The board established our committee to carry out this activity. Drawing on the existing social behavioral research base, the committee was asked to frame its discussion around four key areas:
- behavioral and social risk and protective factors;
- biological, behavioral, and social interactions as they affect health;
- behavioral and social treatment and prevention approaches;
- basic behavioral and social processes.
In addition, the committee was encouraged to consider the following issues in shaping its response: (a) health problems for which behavioral and social sciences research might offer solutions with respect to treatment and prevention, (b) areas of scientific opportunity in the behavioral and social sciences where a substantial investment might pay large dividends in the near future, (c) the public's chief health concerns.
Finally, the committee was asked to give special attention to collaborative research, interdisciplinary projects, and trans-institute initiatives that would have general application to broad areas of illness and health and would be sensitive to perspectives of the various NIH institutes. In considering this charge, the committee decided not to undertake a thorough review of all extant social behavioral research at NIH, a behemoth task beyond the scope of this report. Rather, guided by its original charge, the committee set itself to charting promising future directions where the behavioral and social sciences are well poised to connect with extant biomedical and/or intervention agendas (at individual, community, or population levels). Importantly, the members decided this could best be accomplished not by organizing the report around specific diseases or institutes, thereby following the current structure of NIH, but by providing a broader, more integrative approach.
It should be noted that the behavioral and social sciences, as applied to health, have never been organized around specific diseases. This is understandable, given that many behavioral risk factors (e.g., smoking, obesity, sedentary lifestyles, risky sexual practices) not only themselves co-occur but are also precursors to multiple physiological risk factors and multiple adverse health outcomes. The integrative approach thus gives much greater emphasis to the empirical realities of co-occurring risk and comorbidity, both of which are better understood with an integrative approach. The committee's essential task was to identify key components of a comprehensive approach as to how health outcomes, broadly defined, come about.
It is important to underscore three aspects of the committee's approach to its task. First, the committee covered a huge scientific territory in a very limited period of time and yet was able to quickly achieve consensus regarding the overall structure of the report and the content of the chapters. This efficient exchange was greatly facilitated by the prior experience of committee members in carrying out multidisciplinary science. There was little, if any, disciplinary turf guarding or vying for preeminence; instead, the targeted objective from the moment the work began was to find the best framework for integrating multiple fields and agendas.
Second, the committee had no intention of producing an exhaustive set of future research opportunities. Indeed, it is doubtful that such a comprehensive formulation could be developed by any committee. There was also no attempt made to cover extant programs of every institute within NIH. Stated otherwise, the committee was faced with the unavoidable requirement for selectivity. Nonetheless, the integrative research opportunities that it formulated do represent promising trans-institute initiatives, but they are put forth only as illustrations of the kinds of studies for which there could be substantial scientific payoff and opportunity to improve the public's health. Many vibrant areas of current NIH research are, therefore, inevitably missing from the chapters that follow. We state explicitly that what is not in the report is by no means an indirect message about low-priority status.
Third, the committee wrote this report with the scientific audience at NIH, and not the general public, in mind. Our goal is to communicate a new vision of integrative health to those who will carry out the future research and practice. A critical feature of such integration is the need to demonstrate command of complex areas and their interrelationships. Thus, we have not eliminated all technical details but tried to write about them so as to maximize their accessibility to our audience.
- THE INTEGRATIVE APPROACH TO HEALTH
In the past 25 years, the study of human health has included a distinguished, but neglected, intellectual tradition put forth by numerous investigators, who saw the need for broad integrative frameworks that capture complex pathways to illness and disease. Engel (1977) , for example, formulated a multifactorial model of illness, later subsumed under the rubric “biopsychosocial” that views illness as a result of interacting systems at cellular, tissue, organismic, interpersonal, and environmental levels. As a result, the study of every disease must include the individual, the body, and the surrounding environment as essential components. Lipowski (1977) and Fava and Sonino (2000) set the scope, mission, and methods of psychosomatic medicine as also involving interrelated facets of biological, psychological, and social determinants of health and disease. Around the same time, Henry and Stephens (1977) advanced a sociobiological approach to medicine and health that integrated not only biological, psychological, social, and physical environmental factors but also presented comparative studies of pathways to illness and disease between rodents, nonhuman primates, and humans.
More recently, Worthman (1999) combines human biology, life history theory, and epidemiology to consider variations in human development, giving particular emphasis to the role of hormones in the physiological architecture of the life course. Weiner (1998) offers “notes” toward a comprehensive evolutionary theory that integrates the roles of physical, social, environmental, and psychological factors in the maintenance of good health and the pathogenesis of disease. Keating and Hertzman (1999) assemble a cohesive set of essays that are designed to provide an “integration of knowledge about the determinants of health and human development.” McEwen and Stellar (1993) introduce a multisystem approach to the cumulative physiological toll exacted by adverse behavioral, psychological, social, and environmental influences over the life course. This formulation of cumulative physiological risk is linked to unfolding interactions between genetic and environmental influences over time.
At an even broader level of thinking, E.O. Wilson has adapted and expanded on William Whewell's 1840 notion of consilience ( Wilson, 1998 , p. 8) as a “jumping together” of knowledge by the linking of facts and factbased theory across disciplines to create a common groundwork of explanation. Wilson emphasized that “a unified system of knowledge is the surest means of identifying the still unexplored domains of reality. It provides a clear map of what is known, and it frames the most productive questions for future inquiry.” Wilson's integration includes not only the full range of scientific disciplines but also the humanities and, as such, represents even more distant horizons for promoting health and well-being.
These perspectives collectively provide conceptual background to the theme of integration that guides this report and our related efforts to characterize pathways to multiple health outcomes. The time for this larger synthesis of scientific disciplines in pursuit of human health has come.
- KEY INFLUENCES ON PATHWAYS TO HEALTH
Our task as a committee was one of identifying key elements that comprise an integrated and comprehensive approach to health. When the behavioral and social sciences are emphasized and linked to health, one is automatically led away from a disease-specific emphasis and into a view of multiple pathways to multiple outcomes. For example, smoking is a behavior linked to lung cancer, chronic bronchitis and emphysema, and cardiovascular diseases. Quality of social relationships, in turn, has been linked to cardiovascular diseases, later-life cognitive functioning, and recovery from a variety of illnesses. In both examples, and numerous others documented in this report, there is a need for understanding the pathways underlying these coarse-grained linkages. Moreover, full understanding of pathways requires a long time horizon that includes genetic predispositions and early life antecedents that contribute to later-life health and disease. It requires a multilevel view of life histories in which, for example, gene expression is seen as a dynamic process linked to psychosocial experience and community-level structures.
A behavioral and social science emphasis also leads naturally to a focus on prevention. This is not to detract from the social behavioral contributions to disease etiology, clinical medicine, and the organization and operation of the health care system. However, when the objective is to understand the mechanisms that explain how a range of health outcomes come about, it is appropriate and meaningful to identify health-promoting practices that can prevent or delay illness and disability and reduce the demand for curative health services.
With these observations in mind, the committee identified 10 priority areas for research investment that would integrate the behavioral, social, and biomedical sciences at NIH. These are briefly noted below, with emphasis on why they were selected and what they contribute to the larger mosaic of health.
Predisease Pathways: identification of early and long-term biological, behavioral, psychological, and social precursors to disease. This priority is intended to broaden the time horizons that guide research on disease etiology as well as underscore agendas that may lead to early preventive strategies.
Positive Health: identification of biological, behavioral, and psychosocial factors that contribute to resilience, disease resistance, and wellness. This priority draws attention to the need for greater emphasis throughout NIH on the biopsychosocial factors that help individuals maintain or regain good health throughout the life course.
Environmentally Induced Gene Expression: emphasis on the need to connect modern advances in genetic analysis to environmental factors (behavioral, psychological, social) to clarify their interactions in understanding positive and negative health outcomes.
Personal Ties: the growing body of literature that connects the social world to health and calls for greater explication of the biobehavioral mechanisms by which relationships with significant others (family, friends, co-workers) influence health and disease.
Collective Properties and Healthy Communities: greater emphasis on neighborhood and community-level variables, such as residential instability or social cohesion, and how they contribute to positive or negative health practices and outcomes.
Inequality and Health: builds in the growing awareness that socioeconomic hierarchies, racism, discrimination, and stigmatization are linked with differences in health and illness and calls for greater understanding of the mechanisms through which these effects occur and how they can be reversed.
Population Health: greater understanding of macro-level trends in health status, how the macroeconomy and population health are linked, and the performance of the health care system.
Interventions: expansion of the scope and effectiveness of behavioral, psychosocial, and biological strategies for improving health, including multilevel (individual, family, organizational, population) initiatives.
Methodology: emerges from the recognition that new measurement techniques and study designs are required to link information across diverse levels of analysis (molecular, cellular, behavioral, psychosocial, community) and across time.
Infrastructure: refers to the need for future structures and resources to maintain long-term study populations and train new generations of scientists to integrate health-related knowledge across multiple disciplines.
The scope of these priorities is expansive and integrative, with each encompassing wide areas of research. Some represent phenomena at the individual level, while others deal with macro-level (e.g. population) issues. The chapters that follow elaborate each of these priorities and identify principal recommendations associated with them. Collectively, they comprise the integrated pathway approach to health that is the guiding theme of this report.
- Engel GL. “The need for a new medical model: A challenge for biomedicine” Science. 1977; 196 :129–136. [ PubMed : 847460 ]
- Fava GA, Sonino N. “Psychosomatic medicine: Emerging trends and perspectives” Psychotherapy and Psychosomatics. 2000; 60 :184–197. [ PubMed : 10867586 ]
- Henry JP, Stephens P. Stress, Health and the Social Environment: A Sociobiologic Approach to Medicine. New York: Springer-Verlag; 1977.
- Keating DP, Hertzman C. Developmental Health and the Wealth of Nations: Social, Biological, and Educational Dynamics. New York: The Guilford Press; 1999.
- Lipowski ZJ. Psychosomatic medicine in the seventies: An overview. American Journal of Psychiatry. 1977; 134 :233–244. [ PubMed : 320882 ]
- McEwen BS, Stellar E. “Stress and the Individual: Mechanisms leading to disease” Archives of Internal Medicine. 1993; 153 :2093–2101. [ PubMed : 8379800 ]
- McGinnis JM, Foege WH. “Actual causes of death in the United States” Journal of the American Medical Association. 1993; 270 :2207–2212. [ PubMed : 8411605 ]
- Weiner H. “Notes on an evolutionary medicine” Psychosomatic Medicine. 1998; 60 :510–520. [ PubMed : 9710299 ]
- Wilson EO. New York: Alfred A. Knopf; 1998. Consilience: The Unity of Knowledge.
- Worthman C. “Epidemiology of Human Development” In: Panter-Brick C, Worthman C, editors. Hormones, Health, and Behavior. Chapter 3. Cambridge U.K.: Cambridge University Press; 1999. pp. 47–104.
- Cite this Page National Research Council (US) Committee on Future Directions for Behavioral and Social Sciences Research at the National Institutes of Health; Singer BH, Ryff CD, editors. New Horizons in Health: An Integrative Approach. Washington (DC): National Academies Press (US); 2001. 1, Introduction.
- PDF version of this title (1.1M)
In this Page
Other titles in this collection.
- The National Academies Collection: Reports funded by National Institutes of Health
Related information
- PubMed Links to PubMed
Recent Activity
- Introduction - New Horizons in Health Introduction - New Horizons in Health
Your browsing activity is empty.
Activity recording is turned off.
Turn recording back on
Connect with NLM
National Library of Medicine 8600 Rockville Pike Bethesda, MD 20894
Web Policies FOIA HHS Vulnerability Disclosure
Help Accessibility Careers

New Horizon Research Center
Patient focused clinical research .
Today’s Research… Tomorrow’s Medicine
Advancing medical developments & treatments through quality research, evolving research uncovers new information about medical conditions and possible therapies., “we are proud to be a part of the program and part of the solution to the crisis affecting all of us. we have found a way to give back to the community and to the world. the staff provided a fun experience for us both.” , we pride ourselves on listening to community and being actively involved, current clinical trials.
Clinical research is the study of new pharmaceutical therapies or medical devices that are designed to improve the treatment of a specific disease. When promising new drugs or devices appear, New Horizon Research Group participates in highly specific clinical trials to evaluate these products.
Our Current Research Trials
Interested in participating.
Every day, research uncovers new information about medical conditions and possible therapies. You and many other people may benefit from your willingness to become involved.
Qualified participants will receive study drugs and related testing at no cost and may receive compensation for time and travel.
Learn more about the benefits of participation in a clinical trial on our Volunteers page.
To advance medical developments and treatments through quality research.
Our Mission
We are committed to producing accurate and valid data in an effort to facilitate FDA approval and bring new highly effective and efficient treatment options and alternatives to the market by collaborating with research partners and volunteers. We strive to provide excellent, safe, and high-quality care through strong leadership, teamwork, and advocacy to all of our participants and volunteers.
3 Convenient Locations
Medical Affiliated Research Center 303 Williams Ave. SW Suite 511 Huntsville Alabama 35801 (256) 533-6603
North Alabama Research Center 721 West Market Street Suite B Athens, AL 35611 (256) 771-9919
Cullman Research Center 909 Graham St. SW Suite D Cullman, AL 35055 (256) 735-4262
News and Announcements
How do i get involved as an investigator, a letter from our president.
Our vision is to advance medical developments and treatments through quality research
Employment Opportunities
Our locations.
Medical Affiliated Research Center Huntsville, Alabama (256) 533-6603
North Alabama Research Center Athens, Alabama (256) 771-9919
Cullman Research Center Cullman, Alabama (256) 735-4262
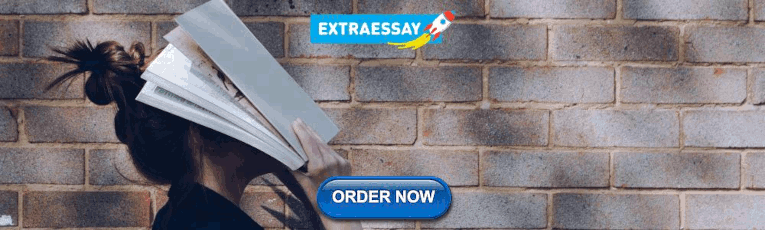
Stay Connected
© 2023 New Horizons Research Group Privacy Policy | Website Design And Development By Flourish Design Studio, Ithaca New York
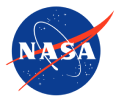
New Horizons Discoveries Keep Coming
NASA's New Horizons – the fastest-ever spacecraft at launch – left Earth in 2006 and hurtled through the void at nearly one million miles per day toward a mysterious world on the solar system’s outer edge. Three billion miles (4.8 billion km) and 9 1/2 years later, the spacecraft flew-by its target: Pluto.
First-time close-up photos of that incredible dwarf planet revealed wonders such as nitrogen glaciers flowing across the surface, mountain ranges rivaling the Rockies, possible ice volcanoes, and areas that are geologically active.
The incredible isn’t over.
New Horizons is on its way to new discoveries deeper in the Kuiper Belt – a region inhabited by ancient remnants from the dawn of the solar system. New Horizons’ next mission is to execute a close flyby of Kuiper Belt Object (KBO) 2014 MU69 on Jan. 1, 2019. At about 20 miles across (30 km), 2014 MU69 is more than 10 times larger and 1,000 times more massive than typical comets.
“MU69’s orbit indicates it is a cold classical Kuiper Belt Object, one of the most primordial objects in the solar system,” explains Alan Stern, New Horizons Principal Investigator. “Additionally, a recent telescopic observation made from Argentina leads us to believe that MU69 may be part of a binary pair, or two objects orbiting one another.”
Meanwhile, there’s more news about Pluto.
Images recently analyzed from New Horizons’ cameras revealed what appear to be small, low-lying isolated clouds – the first to be seen on the dwarf planet.
Stern says, "If there are clouds, it would mean the weather on Pluto is even more complex than we imagined."
Other data and images from the spacecraft are showing that Pluto’s brightest surface region is among the most reflective in the solar system. Why is the surface so reflective?
Stern explains that, “The atmosphere can snow, making bright surface deposits.”
Another recent New Horizons discovery is landslides on Pluto’s moon Charon. These are the first landslides seen in the Kuiper Belt.
The discoveries are far from over. The last bits of science data from the spacecraft’s July 2015 Pluto flyby reached Earth on Oct 27, 2016. Over the next few years, scientists on the New Horizons team will be scrutinizing the data. At the same time, the spacecraft will be exploring MU69, other KBOs, and the surrounding territory to solve more mysteries about the far reaches of the solar system.
New Horizons instruments have been hard at work since well before the spacecraft arrived at Pluto.
The Pluto Energetic Particle Spectrometer Science Investigation (PEPSSI) instrument has been measuring the composition and density of high energy charged particles in the Sun’s outer heliosphere. New Horizons’ Solar Wind Around Pluto (SWAP) instrument collected solar wind observations en route from Earth to Pluto and is still collecting them out in the Kuiper Belt. New Horizons also has a dust detector, built and conceived by students on the New Horizons team, which detects impacts of particles from asteroids and comets.
By 2021, New Horizons will make observations out to 50 times the distance from the Earth to the sun.
The incredible keeps coming.
For more news from the outer reaches, stay tuned to science.nasa.gov .
Related Terms
- Planetary Science
Explore More
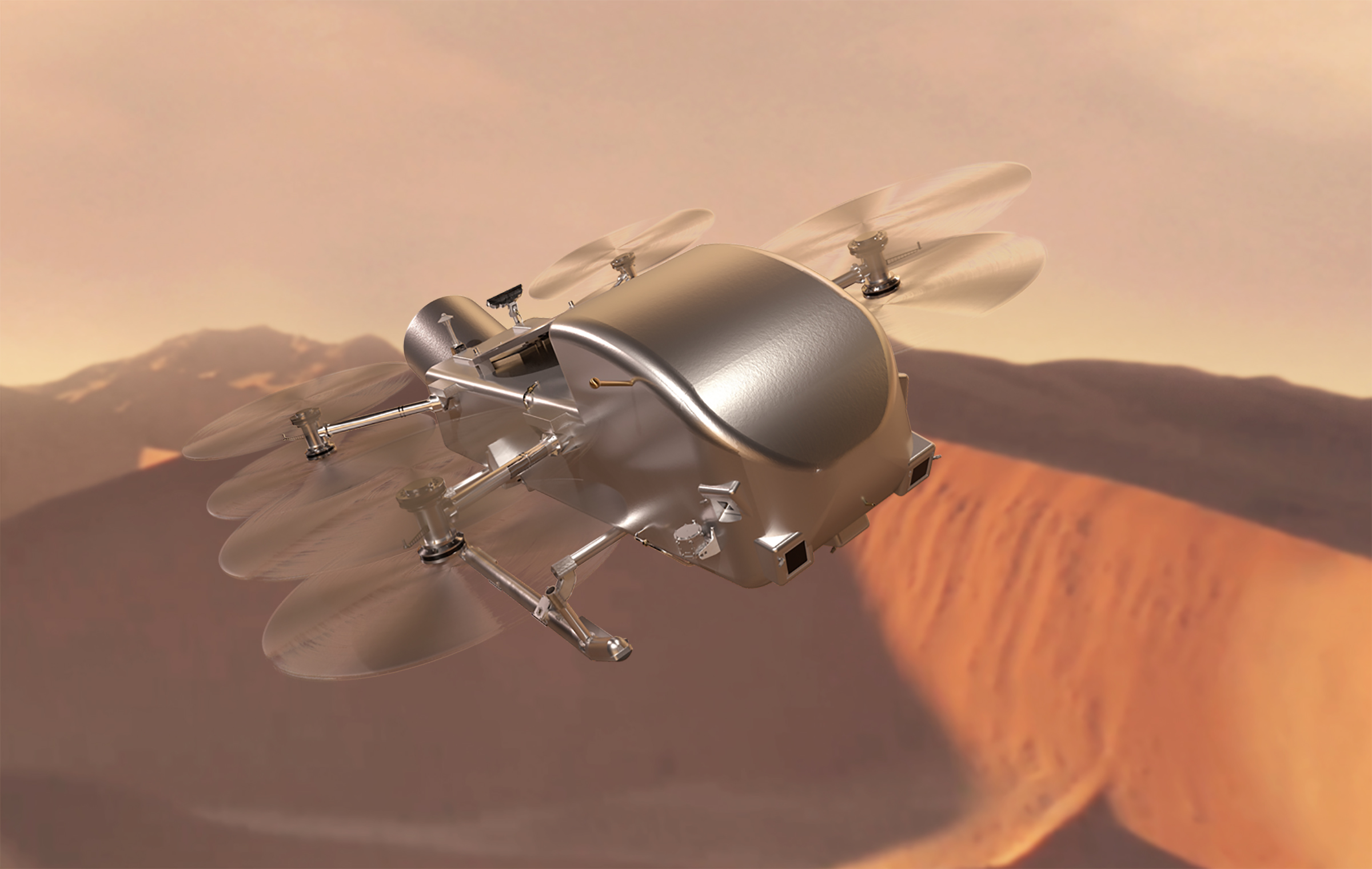
NASA’s Dragonfly Rotorcraft Mission to Saturn’s Moon Titan Confirmed
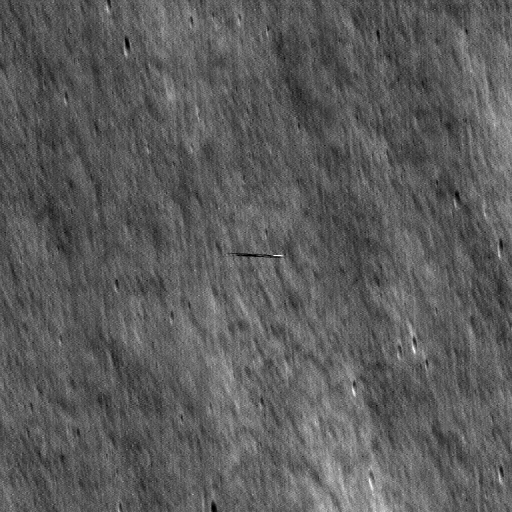
NASA’s LRO Finds Photo Op as It Zips Past SKorea’s Danuri Moon Orbiter
Editor’s Note: This feature was updated on April 9, 2024, to correct the velocity difference between spacecraft to 11,500 kilometers per hour. NASA’s LRO (Lunar Reconnaissance Orbiter), which has been circling and studying the Moon for 15 years, captured several images of Korea Aerospace Research Institute’s Danuri lunar orbiter last month. The two spacecraft, traveling […]
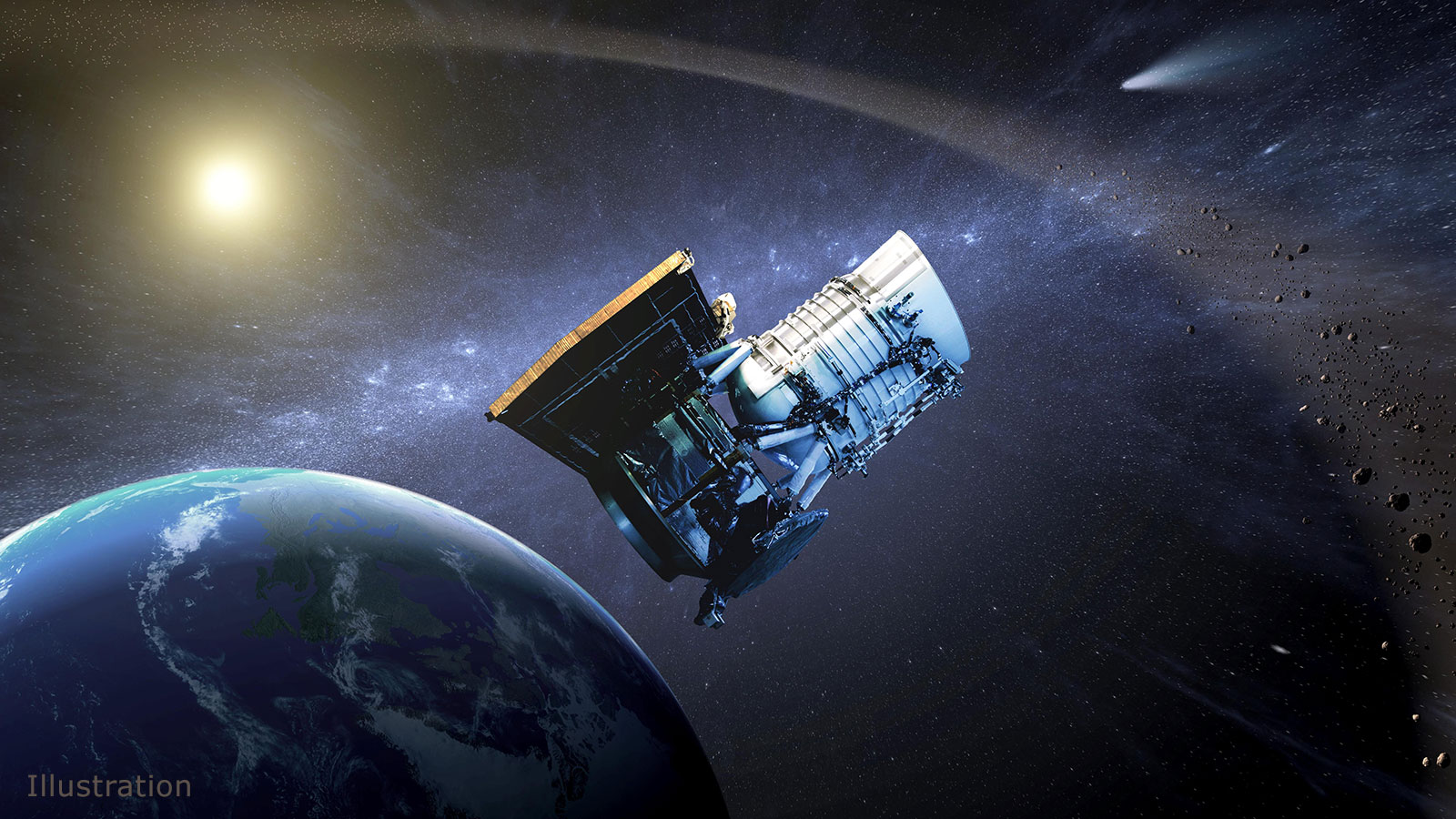
NASA’s NEOWISE Extends Legacy With Decade of Near-Earth Object Data
As the infrared space telescope continues its long-duration survey of the universe, it is creating a unique resource for future astronomers to make new discoveries. NASA’s NEOWISE mission has released its 10th year of infrared data – the latest in a unique long-duration (or “time-domain”) survey that captures how celestial objects change over long periods. […]
Discover More Topics From NASA
James Webb Space Telescope
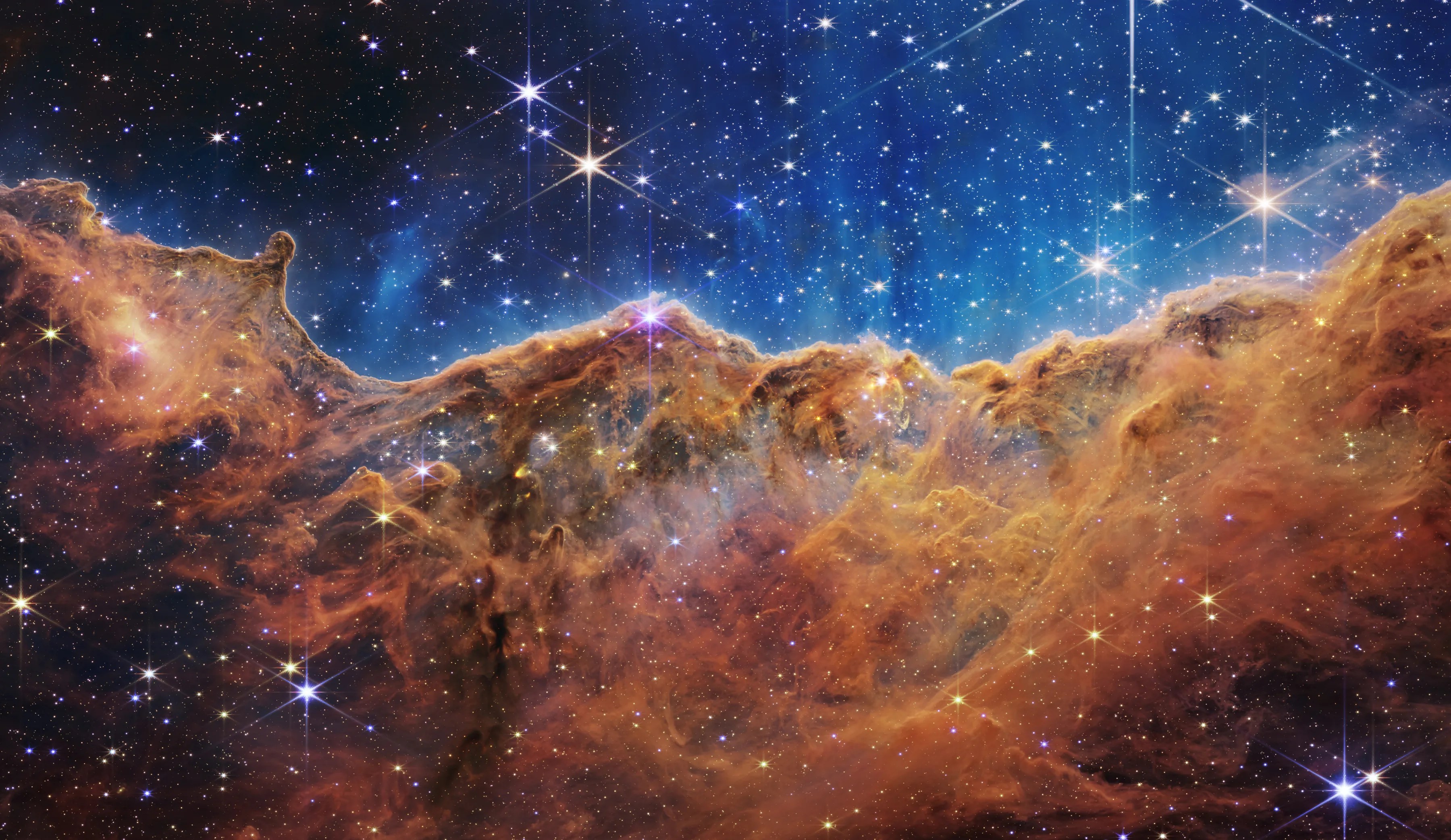
Perseverance Rover
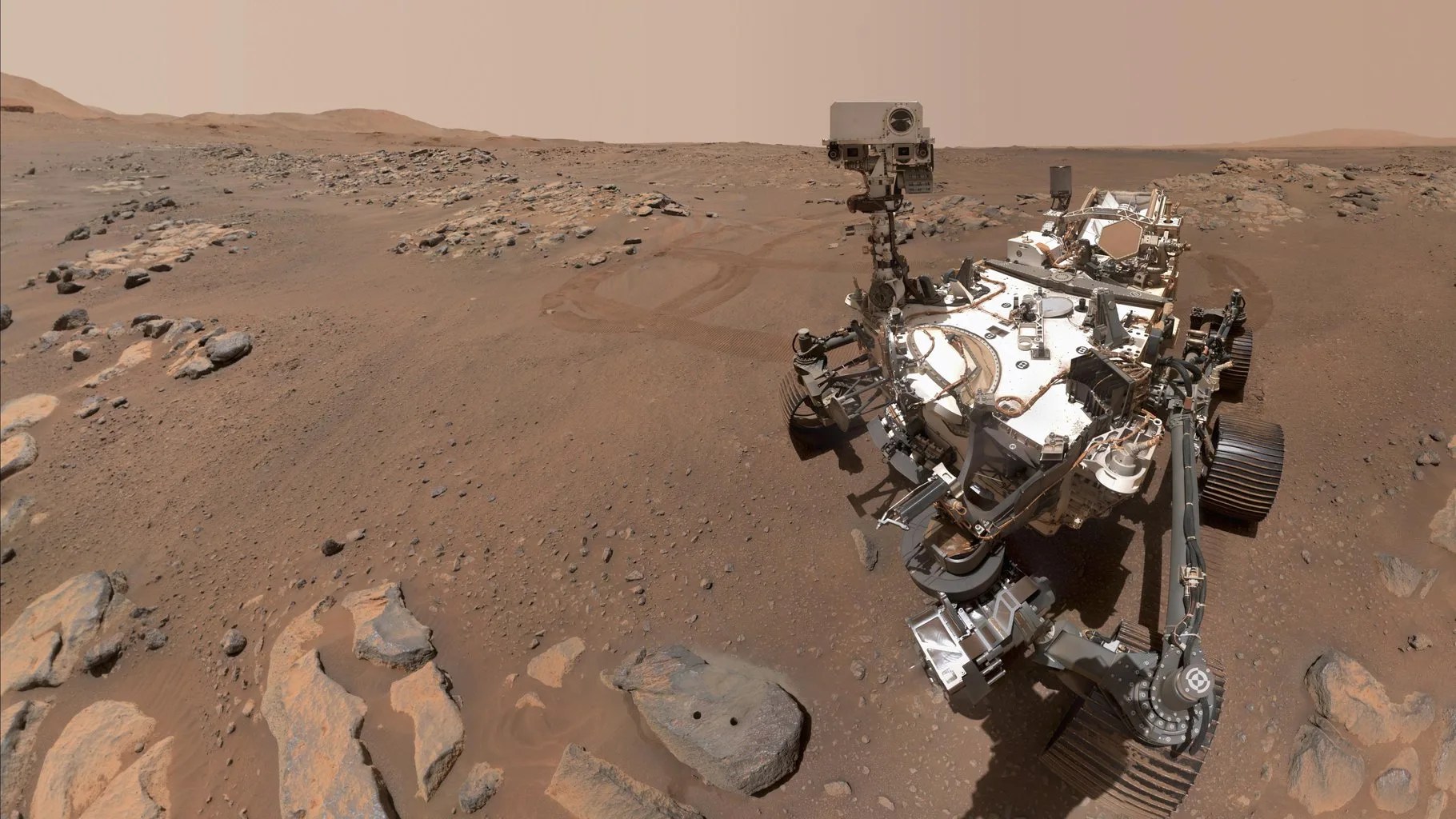
Parker Solar Probe
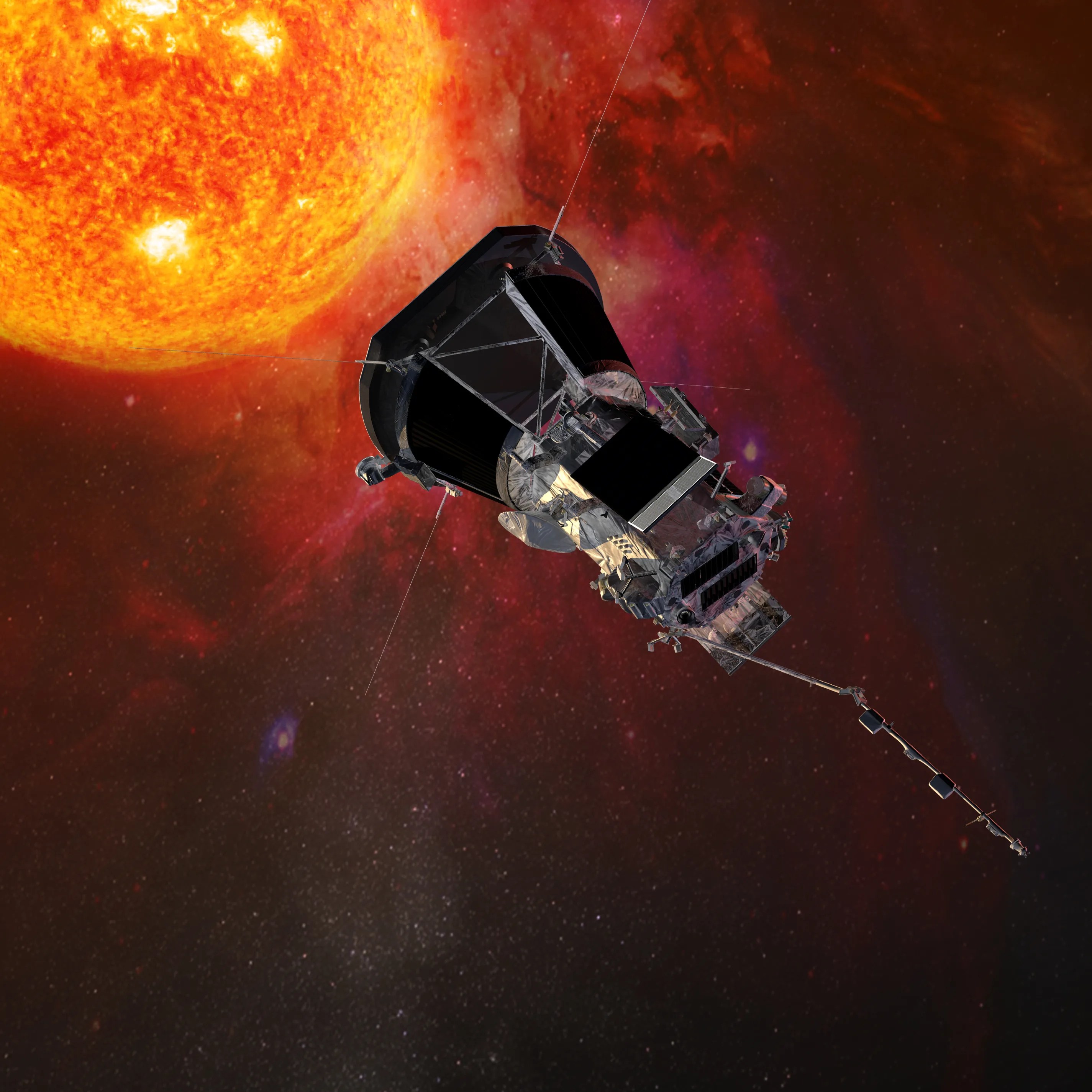
Press Release
New horizons team publishes first research paper in science, describing numerous pluto system findings.
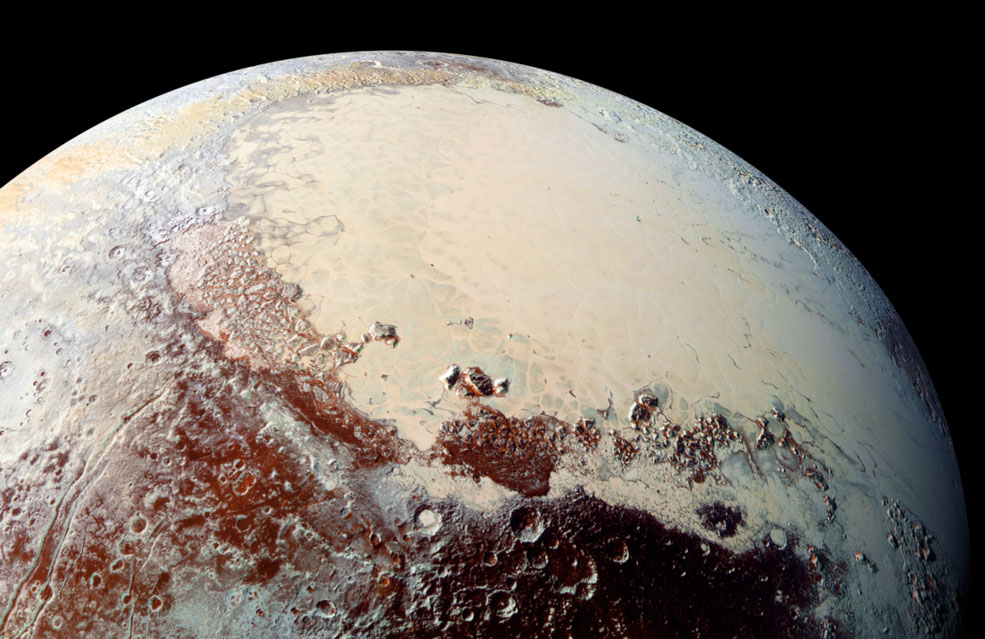
This high-resolution image captured by NASA’s New Horizons spacecraft combines blue, red and infrared images taken by the Ralph/Multispectral Visual Imaging Camera (MVIC). The bright expanse is the western lobe of the “heart,” informally called Sputnik Planum, which has been found to be rich in nitrogen, carbon monoxide and methane ices.
Credit: NASA/Johns Hopkins University Applied Physics Laboratory/Southwest Research Institute
Thu, 10/15/2015 - 09:11
From Pluto’s unusual heart-shaped region to its extended atmosphere and intriguing moons, New Horizons has revealed a degree of diversity and complexity in the Pluto system that few expected in the frigid outer reaches of the solar system.
The New Horizons team describes a wide range of findings about the Pluto system in its first science paper, released today. “The Pluto System: Initial Results from its Exploration by New Horizons,” led by mission Principal Investigator Alan Stern, appears as the cover story in the Oct. 16 issue of Science , just three months after NASA’s historic first exploration of the Pluto system in July.
“The New Horizons mission completes our initial reconnaissance of the solar system, giving humanity our first look at this fascinating world and its system of moons,” said Jim Green, director of planetary science at NASA Headquarters in Washington. “New Horizons is not only writing the textbook on the Pluto system, it’s serving to inspire current and future generations to keep exploring — to keep searching for what’s beyond the next hill.”
NASA’s New Horizons spacecraft reached a distance of 7,750 miles (12,500 kilometers) from Pluto’s surface during its July 14 closest approach, gathering so much data it will take almost another year to return to Earth. The data returned so far show a surprisingly wide variety of landforms and terrain ages on Pluto, as well as variations in color, composition and albedo (surface reflectivity). Team members also discovered evidence for a water-ice rich crust, multiple haze layers above the surface in Pluto’s atmosphere, and that Pluto is somewhat larger and a bit more ice rich than expected.
“The Pluto system surprised us in many ways, most notably teaching us that small planets can remain active billions of years after their formation,” said Stern, with the Southwest Research Institute (SwRI) in Boulder, Colorado. “We were also taught important lessons by the degree of geological complexity that both Pluto and its large moon Charon display.”
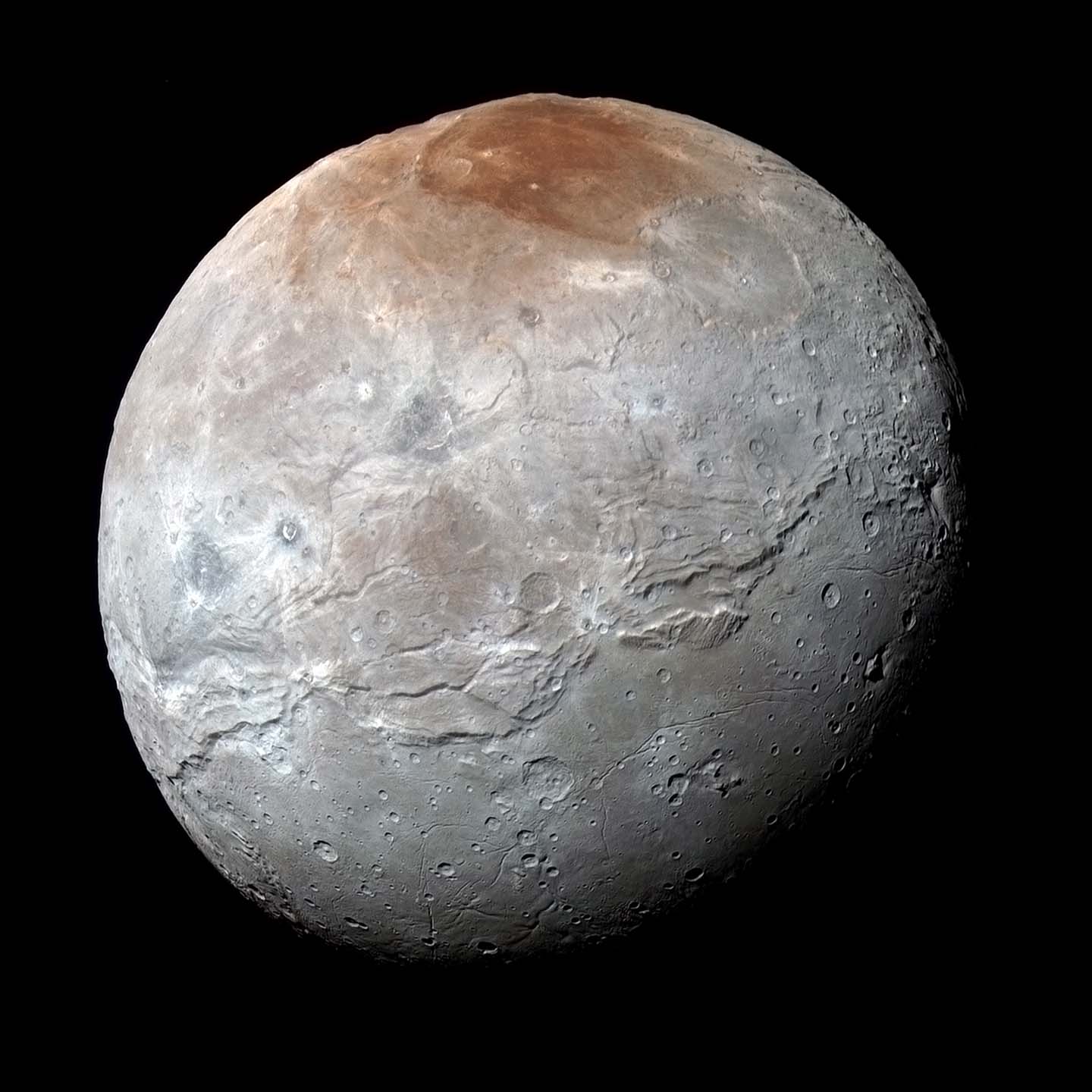
NASA’s New Horizons captured this high-resolution enhanced color view of Pluto’s largest moon, Charon, just before closest approach on July 14, 2015.
Some of the processes on Pluto appear to have occurred geologically recently, including those that involve the water-ice rich bedrock as well as the more volatile, and presumably more mobile, ices of the western lobe of Pluto’s “heart.” The diverse geology and apparent recent activity raise fundamental questions about how small planetary bodies remain active many billions of years after formation. The research suggests that other large worlds in the Kuiper belt — such as Eris, Makemake and Haumea — could also have similarly complex histories that rival those of terrestrial planets such as Mars and Earth.
The New Horizons team notes that Triton, likely a former Kuiper Belt planet captured by Neptune, was considered the best analog for Pluto prior to the July 14 flyby. The team now believes that the geologies of Triton and Pluto are more different than similar, but will know more as additional data are returned.
New Horizons is part of NASA’s New Frontiers Program, managed by the agency’s Marshall Space Flight Center in Huntsville, Alabama. The Johns Hopkins University Applied Physics Laboratory, Laurel, Maryland, designed, built and operates the New Horizons spacecraft and manages the mission for NASA’s Science Mission Directorate. SwRI leads the science mission, payload operations and encounter science planning.
RELATED AREA OF IMPACT
Media contacts.
The Applied Physics Laboratory, a not-for-profit division of The Johns Hopkins University, meets critical national challenges through the innovative application of science and technology. For more information, visit www.jhuapl.edu .
Johns Hopkins APL
Michael buckley, nasa headquarters, dwayne brown, laurie cantillo, southwest research institute, maria stothoff.
This website uses cookies to measure traffic and improve your experience. View our Privacy Policy to learn more.
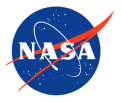
Suggested Searches
- Climate Change
- Expedition 64
- Mars perseverance
- SpaceX Crew-2
- International Space Station
- View All Topics A-Z
Humans in Space
Earth & climate, the solar system, the universe, aeronautics, learning resources, news & events.
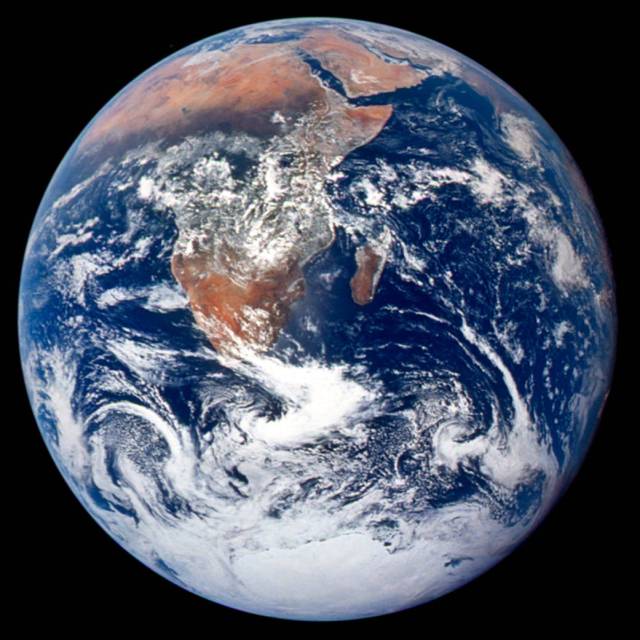
Join NASA in Celebrating Earth Day 2024 by Sharing a #GlobalSelfie
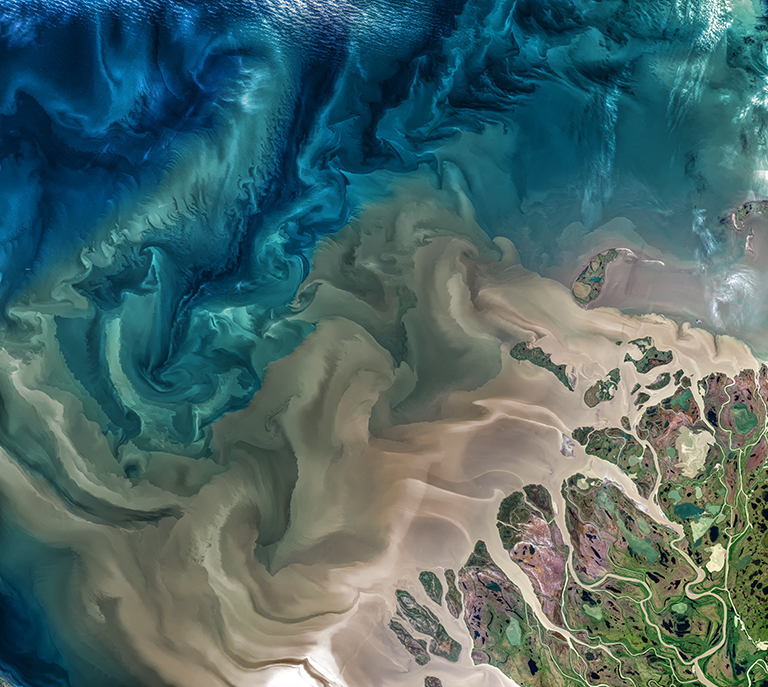
NASA Selects New Aircraft-Driven Studies of Earth and Climate Change
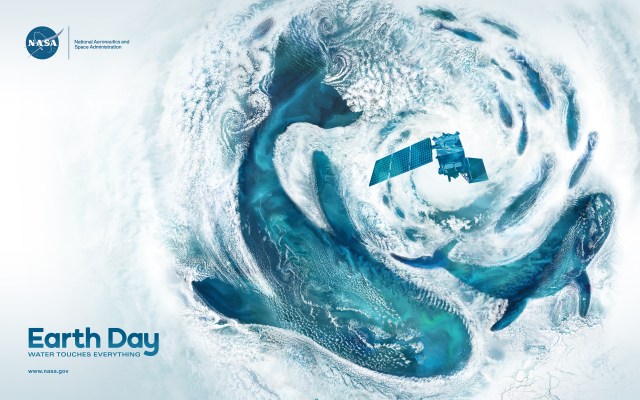
The Ocean Touches Everything: Celebrate Earth Day with NASA
- Search All NASA Missions
- A to Z List of Missions
- Upcoming Launches and Landings
- Spaceships and Rockets
- Communicating with Missions
- James Webb Space Telescope
- Hubble Space Telescope
- Why Go to Space
- Astronauts Home
- Commercial Space
- Destinations
- Living in Space
- Explore Earth Science
- Earth, Our Planet
- Earth Science in Action
- Earth Multimedia
- Earth Science Researchers
- Pluto & Dwarf Planets
- Asteroids, Comets & Meteors
- The Kuiper Belt
- The Oort Cloud
- Skywatching
- The Search for Life in the Universe
- Black Holes
- The Big Bang
- Dark Energy & Dark Matter
- Earth Science
- Planetary Science
- Astrophysics & Space Science
- The Sun & Heliophysics
- Biological & Physical Sciences
- Lunar Science
- Citizen Science
- Astromaterials
- Aeronautics Research
- Human Space Travel Research
- Science in the Air
- NASA Aircraft
- Flight Innovation
- Supersonic Flight
- Air Traffic Solutions
- Green Aviation Tech
- Drones & You
- Technology Transfer & Spinoffs
- Space Travel Technology
- Technology Living in Space
- Manufacturing and Materials
- Science Instruments
- For Kids and Students
- For Educators
- For Colleges and Universities
- For Professionals
- Science for Everyone
- Requests for Exhibits, Artifacts, or Speakers
- STEM Engagement at NASA
- NASA's Impacts
- Centers and Facilities
- Directorates
- Organizations
- People of NASA
- Internships
- Our History
- Doing Business with NASA
- Get Involved
- Aeronáutica
- Ciencias Terrestres
- Sistema Solar
- All NASA News
- Video Series on NASA+
- Newsletters
- Social Media
- Media Resources
- Upcoming Launches & Landings
- Virtual Events
- Sounds and Ringtones
- Interactives
- STEM Multimedia
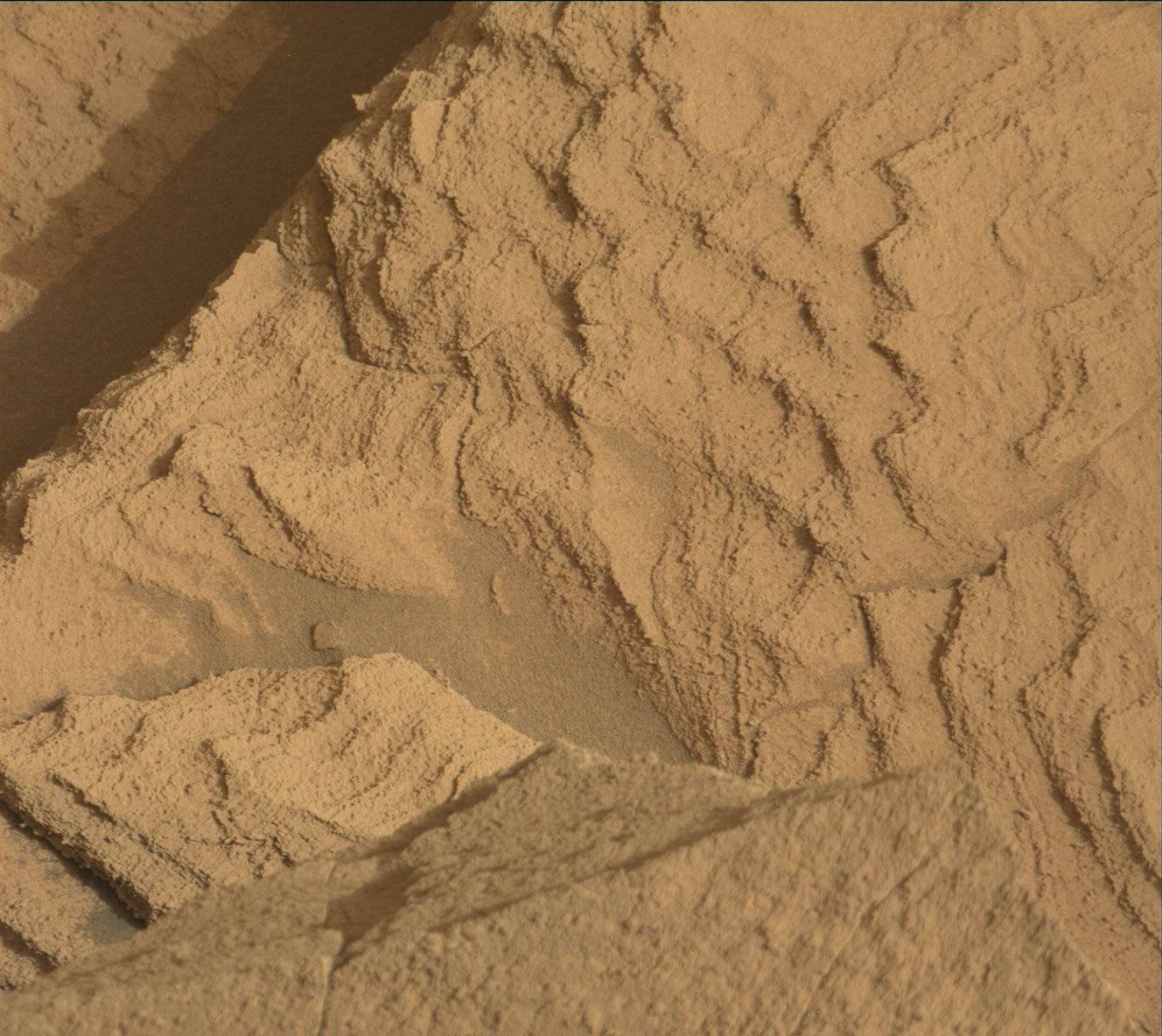
Sols 4161-4163: Double Contact Science
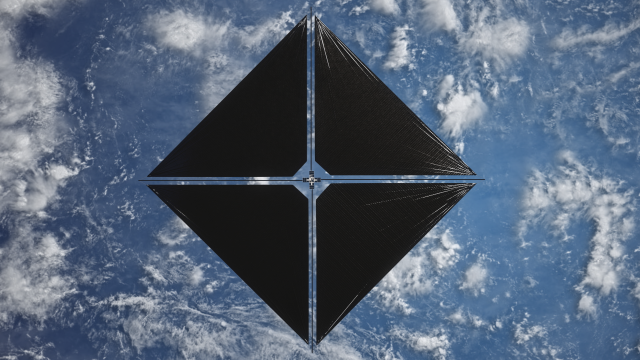
NASA Invites Media to Learn About New Tech Mission Powered by the Sun
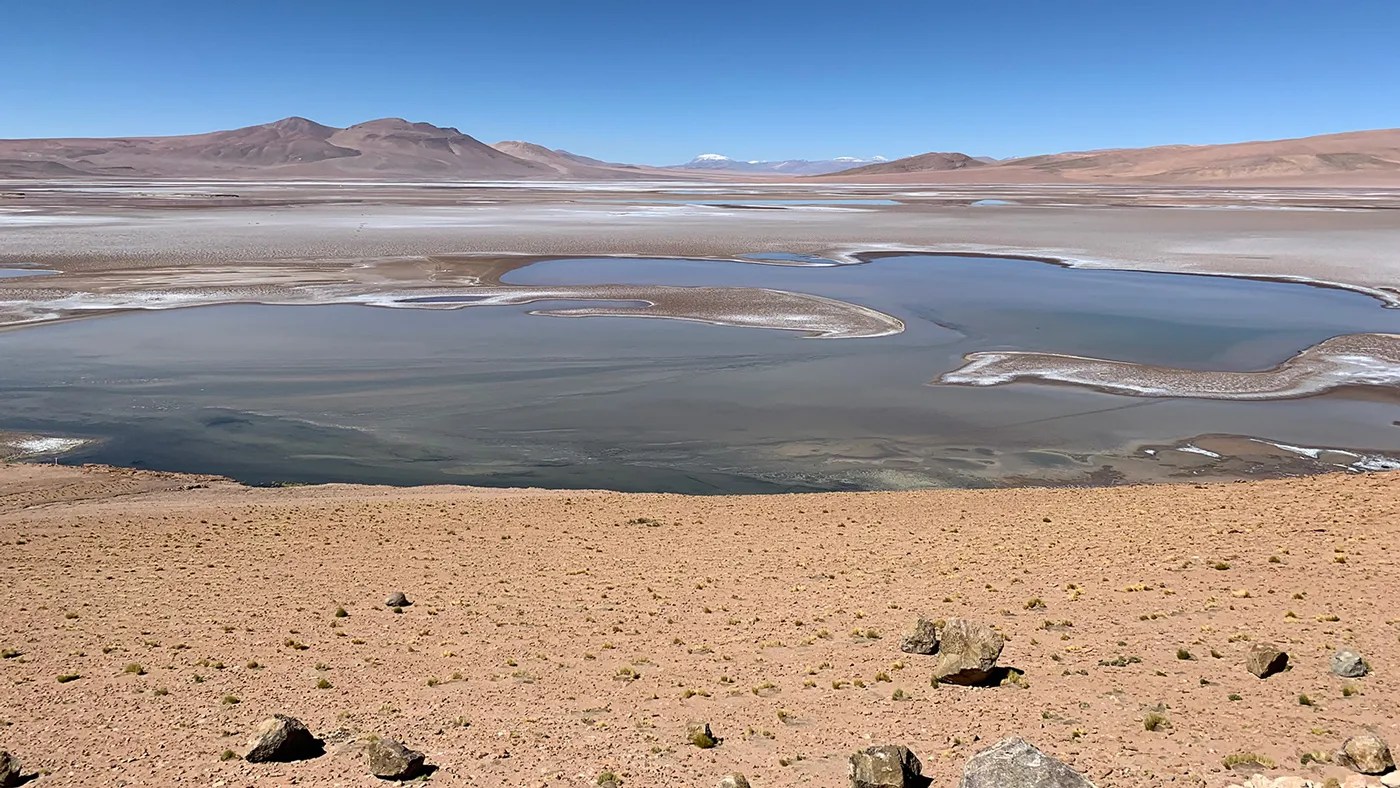
Why is Methane Seeping on Mars? NASA Scientists Have New Ideas

Work Underway on Large Cargo Landers for NASA’s Artemis Moon Missions
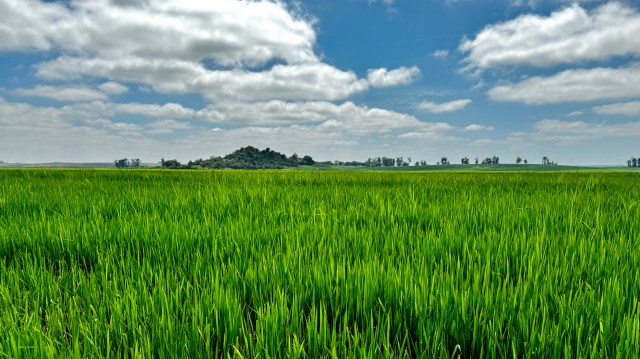
NASA Open Science Initiative Expands OpenET Across Amazon Basin
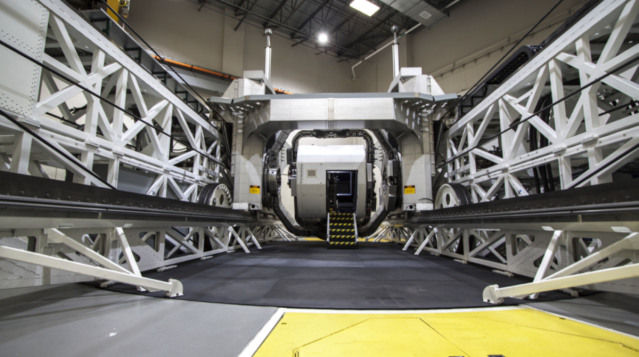
NASA Motion Sickness Study Volunteers Needed!
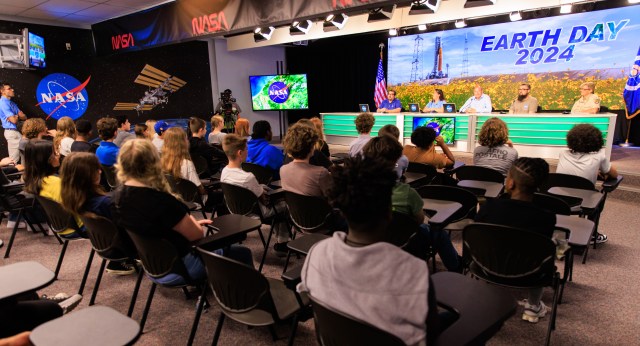
Students Celebrate Rockets, Environment at NASA’s Kennedy Space Center
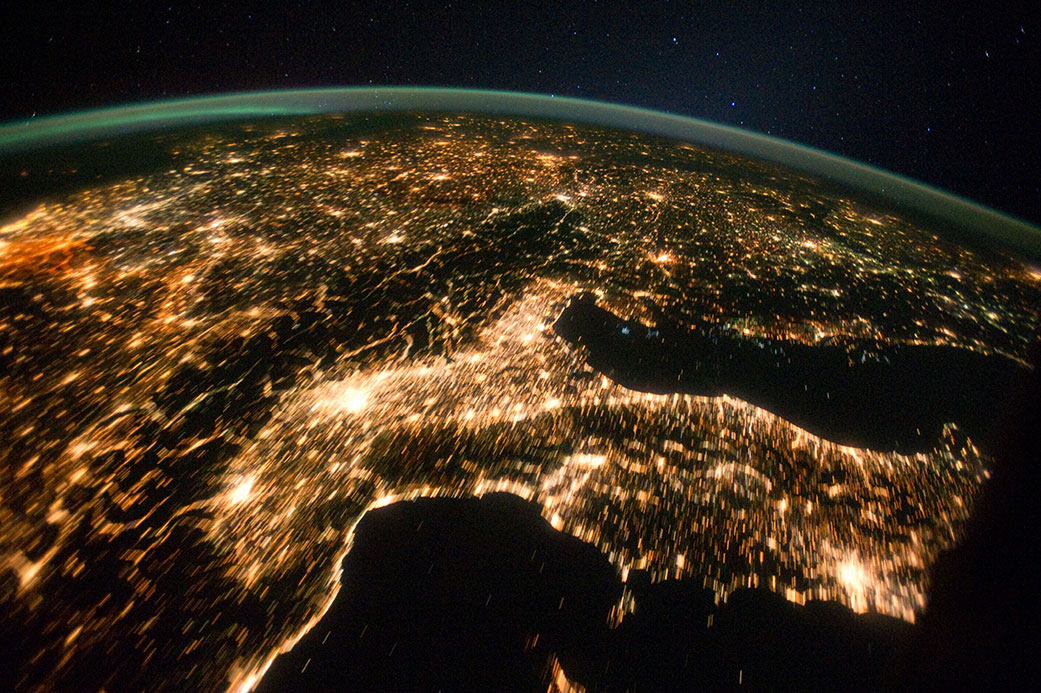
AI for Earth: How NASA’s Artificial Intelligence and Open Science Efforts Combat Climate Change
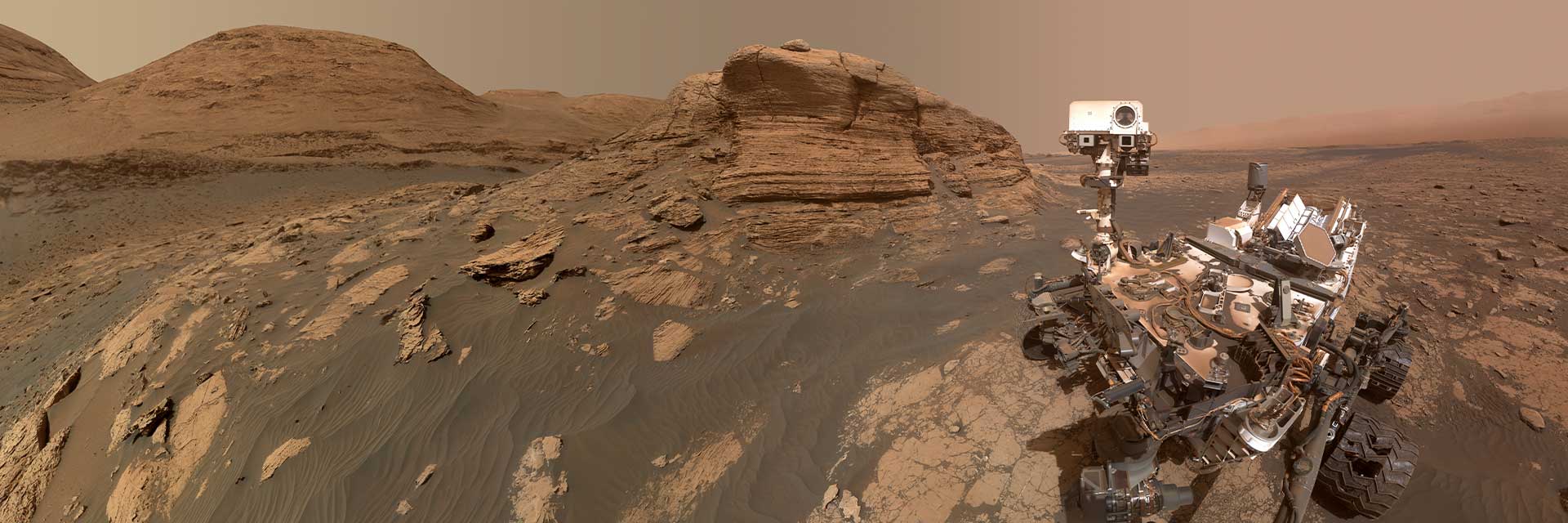
Mars Science Laboratory: Curiosity Rover
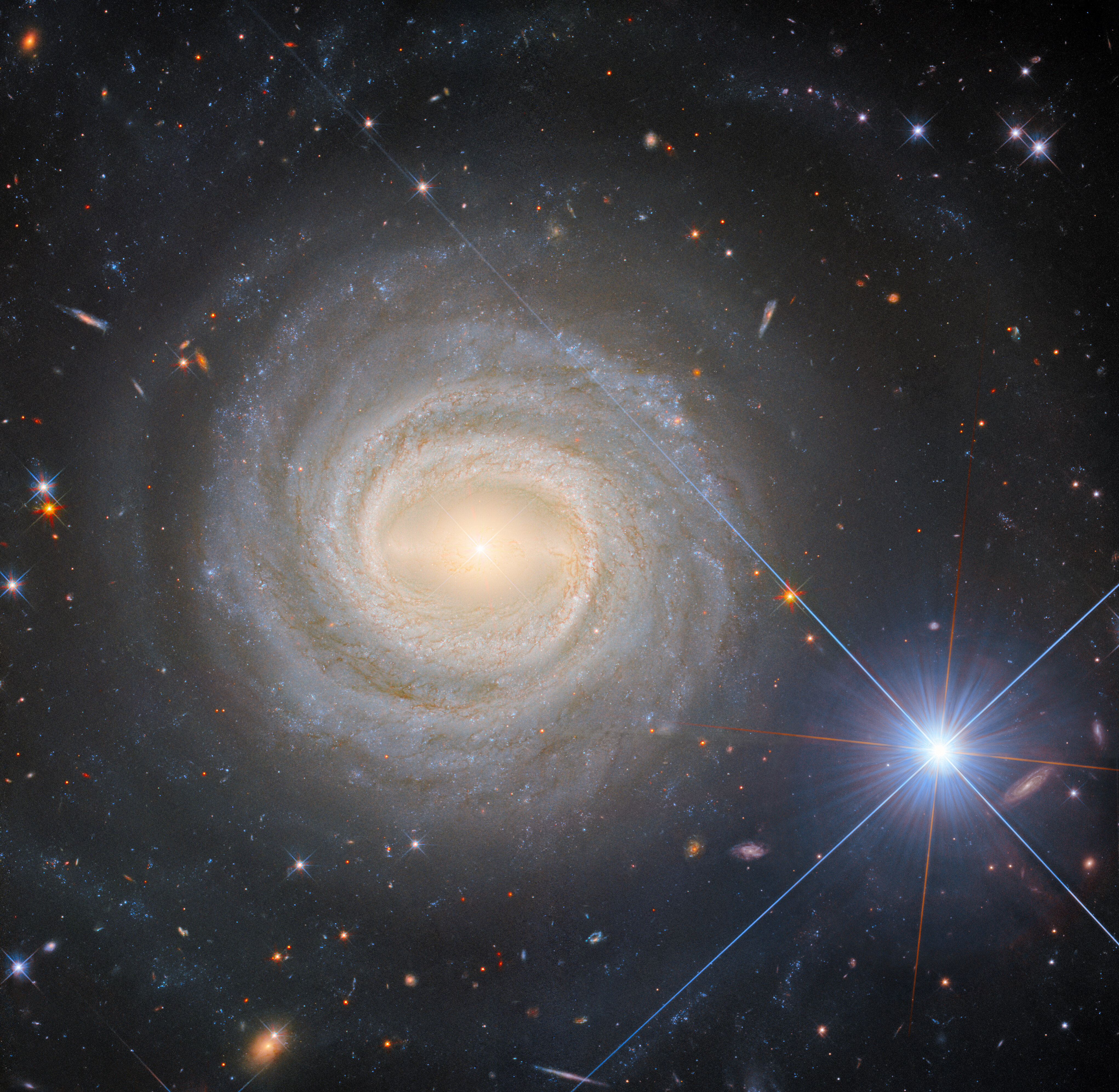
Hubble Captures a Bright Galactic and Stellar Duo
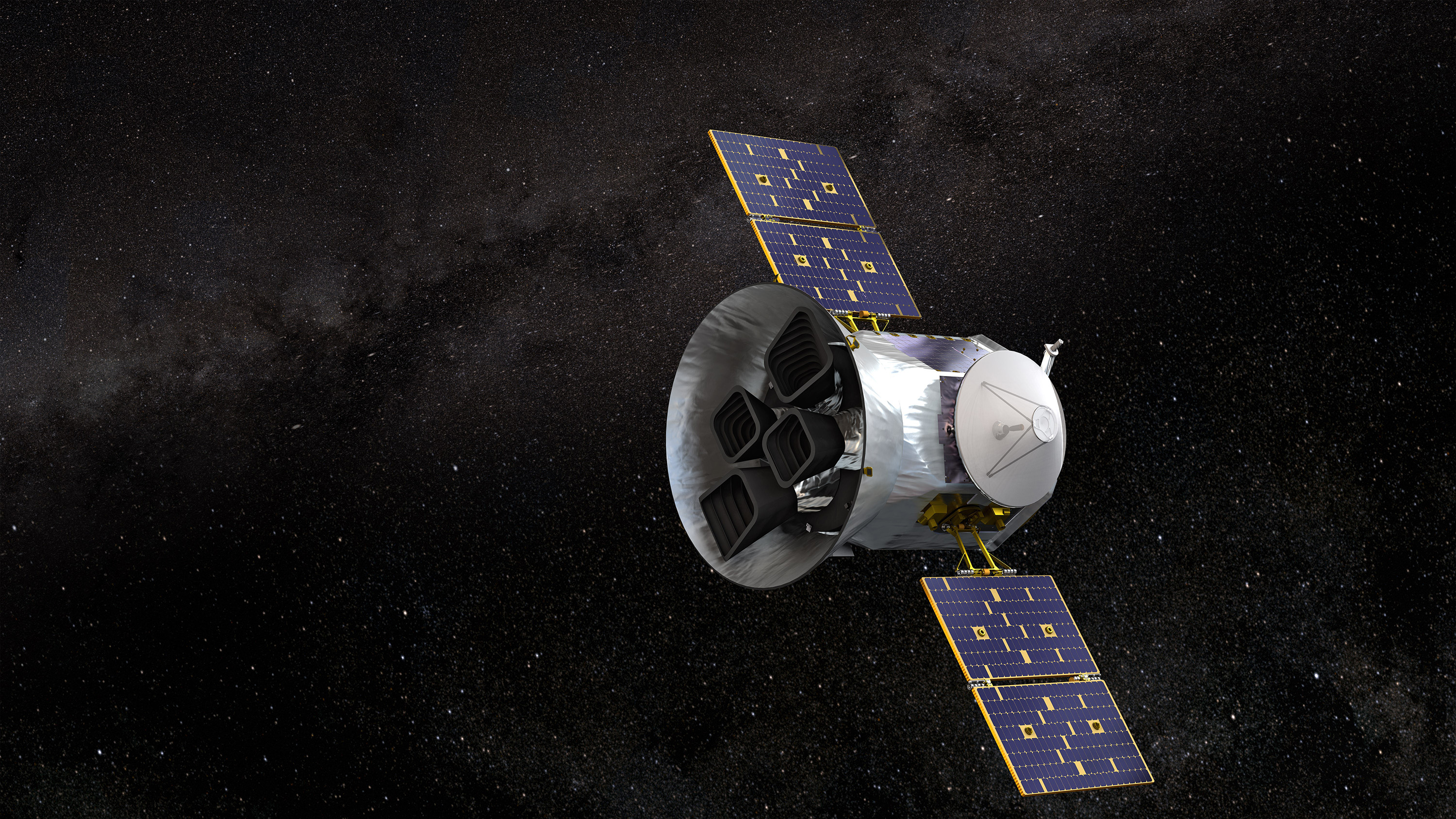
NASA’s TESS Returns to Science Operations
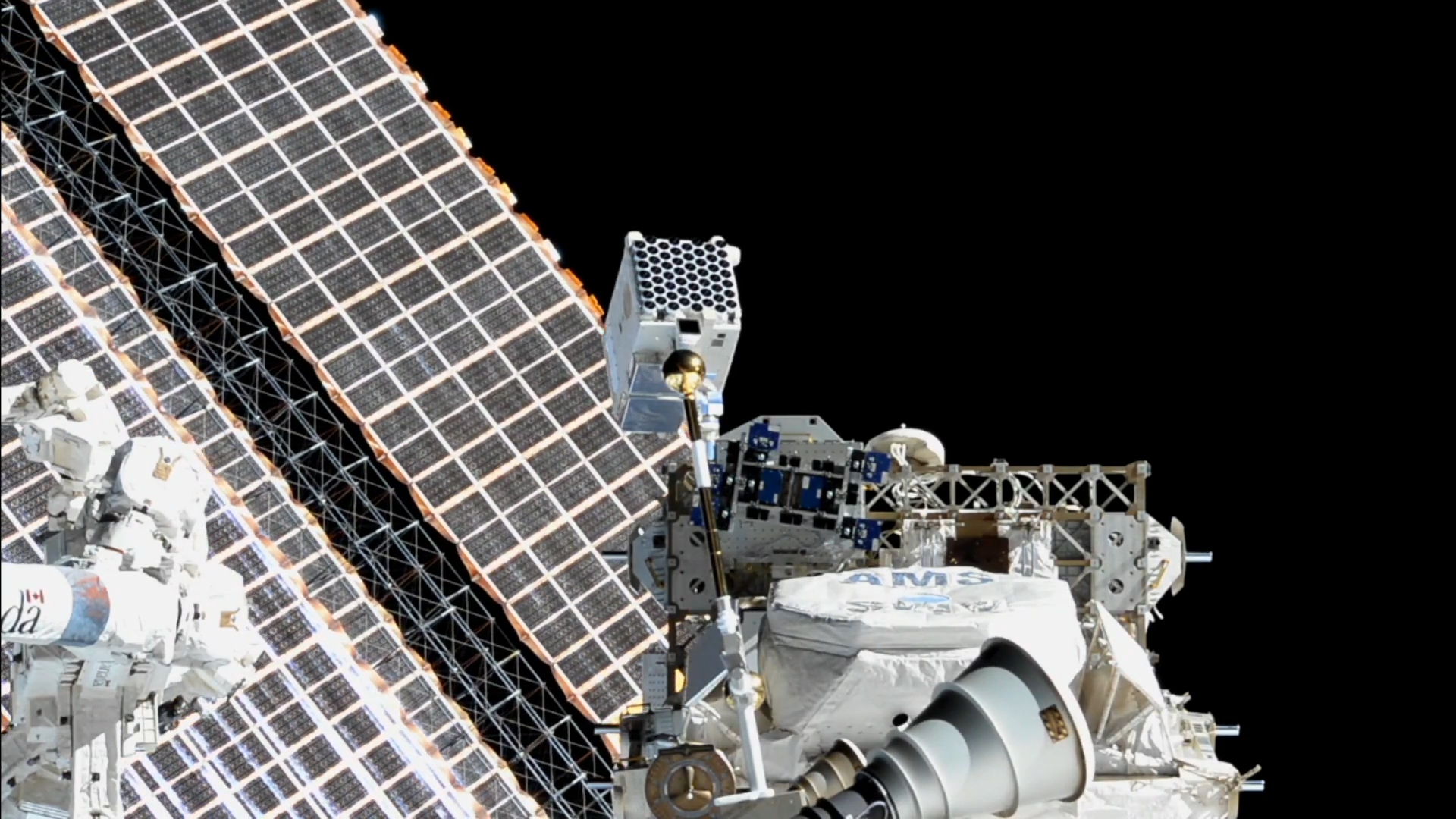
Astronauts To Patch Up NASA’s NICER Telescope
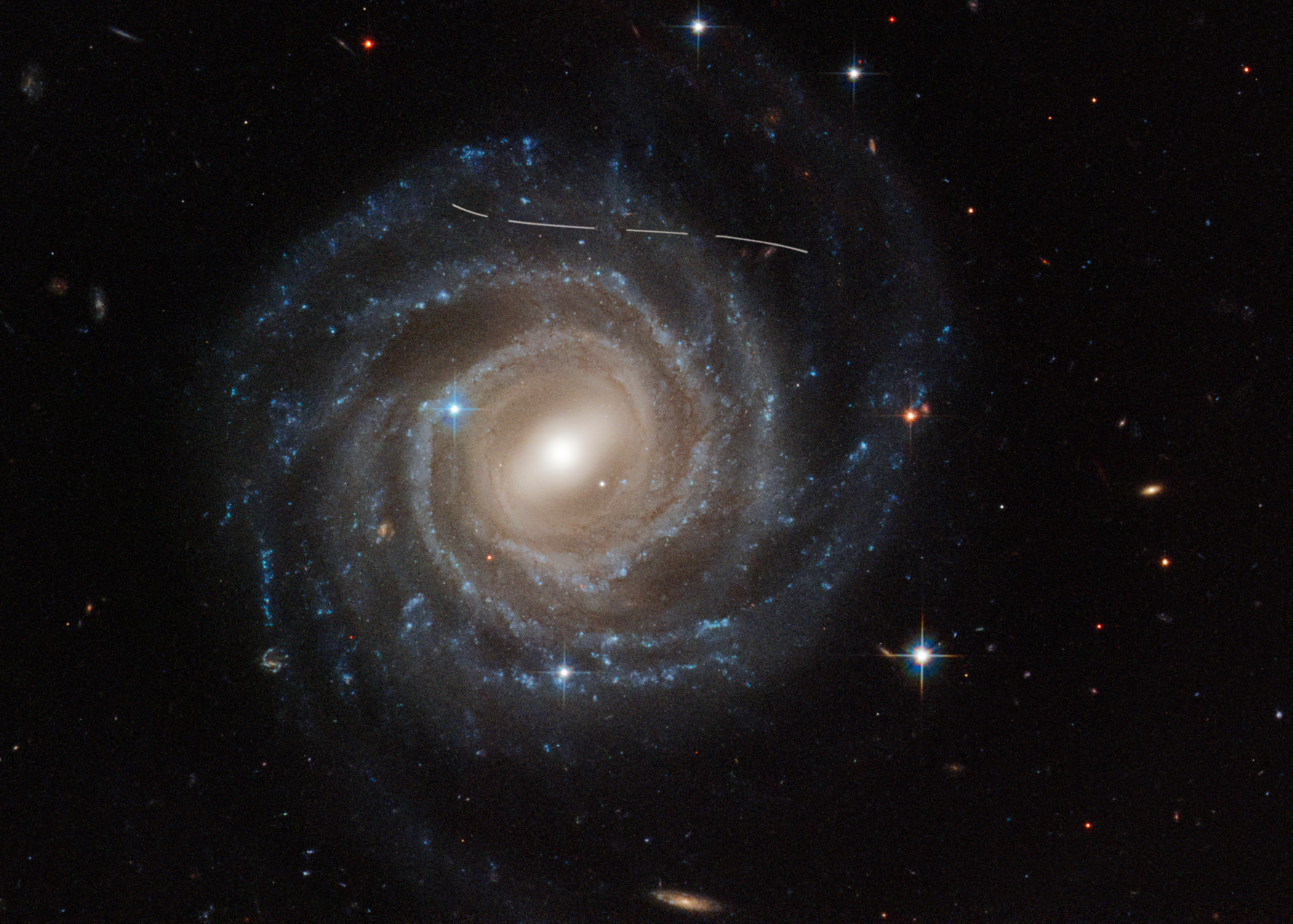
Hubble Goes Hunting for Small Main Belt Asteroids
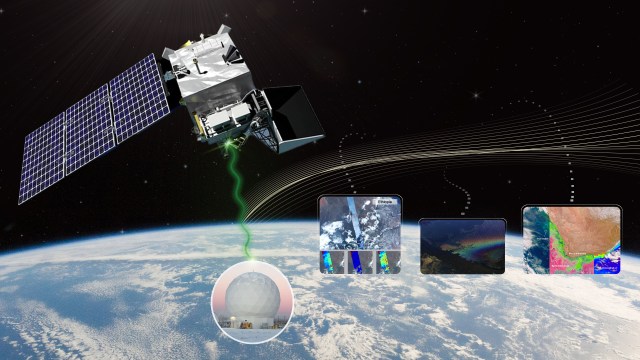
NASA’s Near Space Network Enables PACE Climate Mission to ‘Phone Home’
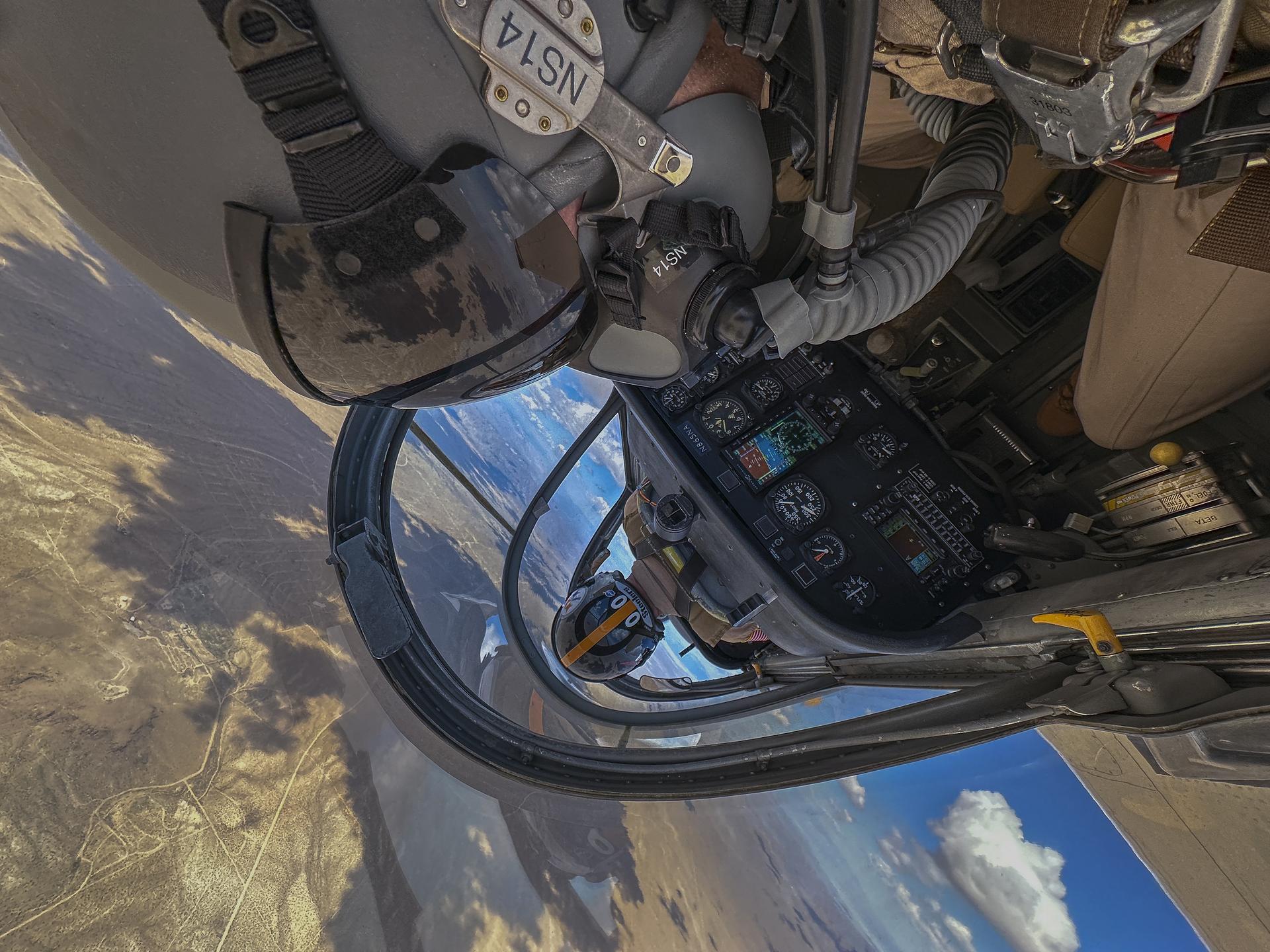
NASA Photographer Honored for Thrilling Inverted In-Flight Image
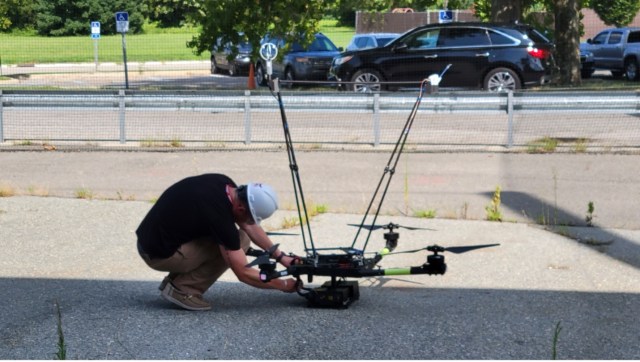
NASA Langley Team to Study Weather During Eclipse Using Uncrewed Vehicles
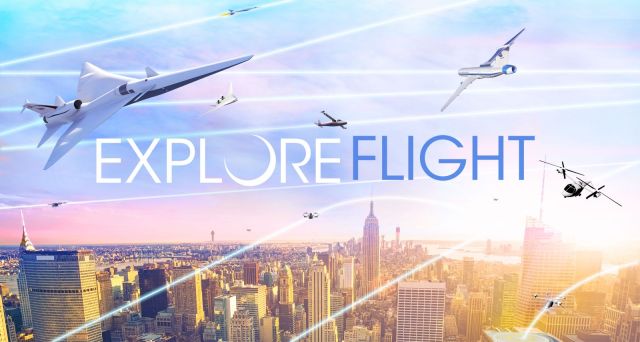
ARMD Solicitations
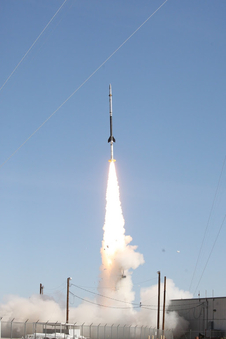
Amendment 10: B.9 Heliophysics Low-Cost Access to Space Final Text and Proposal Due Date.
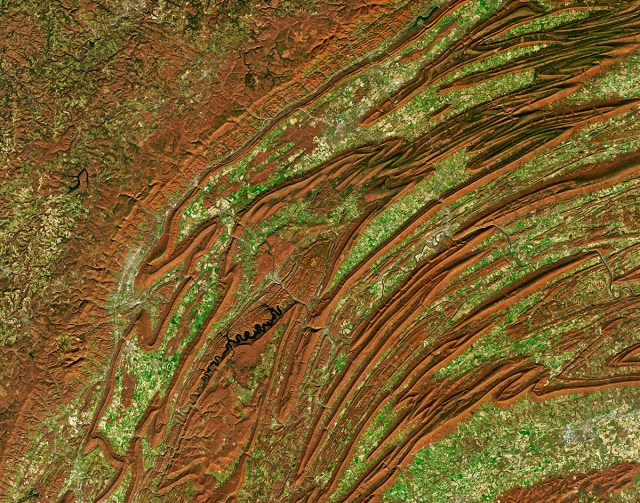
Tech Today: Taking Earth’s Pulse with NASA Satellites
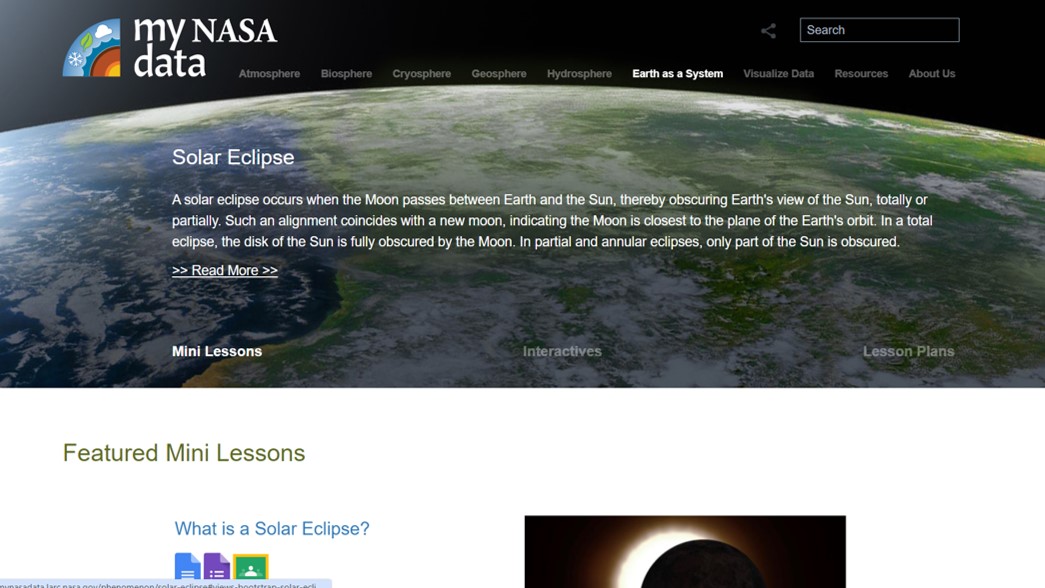
My NASA Data Milestones: Eclipsed by the Eclipse!
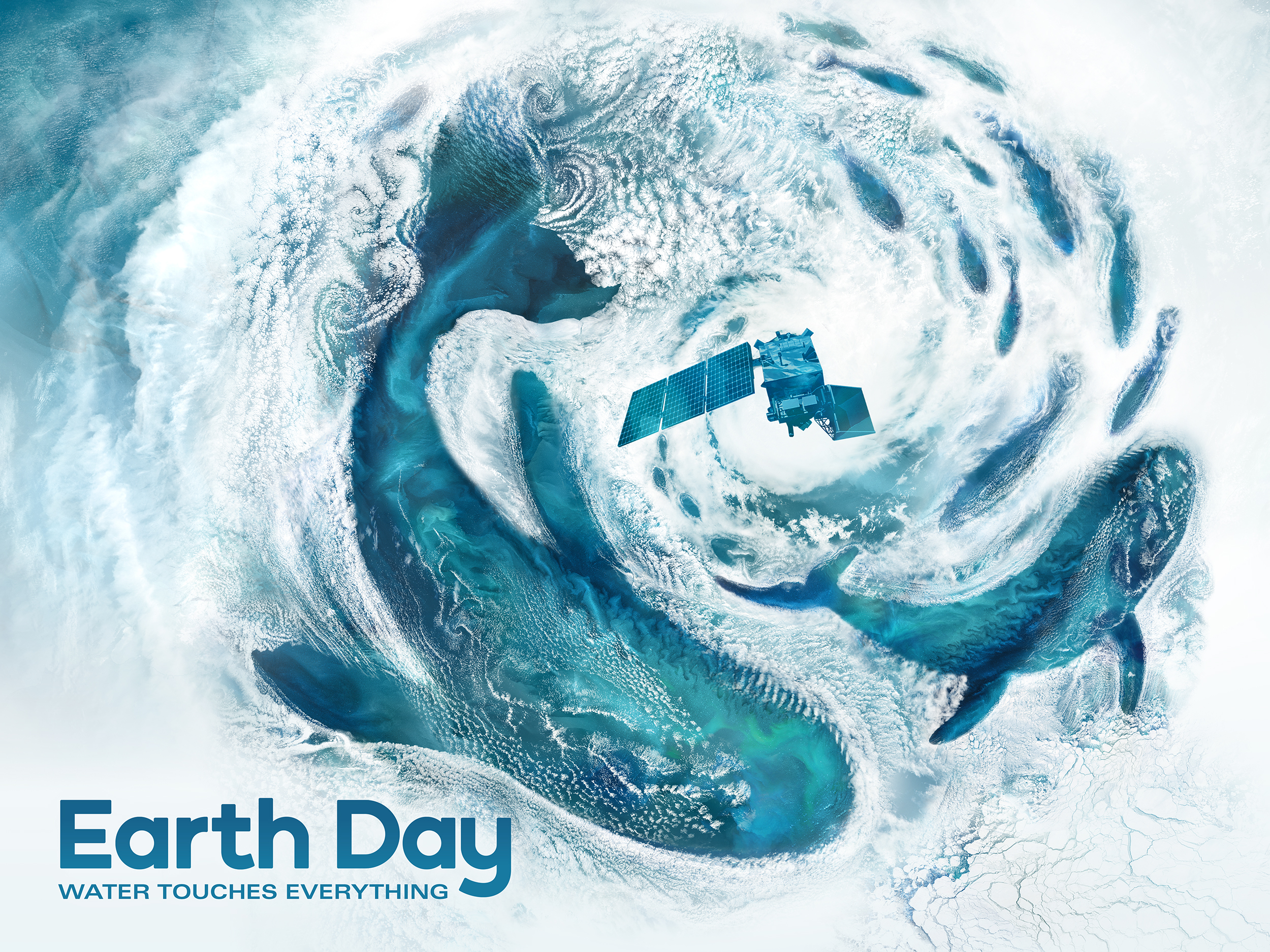
Earth Day 2024: Posters and Virtual Backgrounds
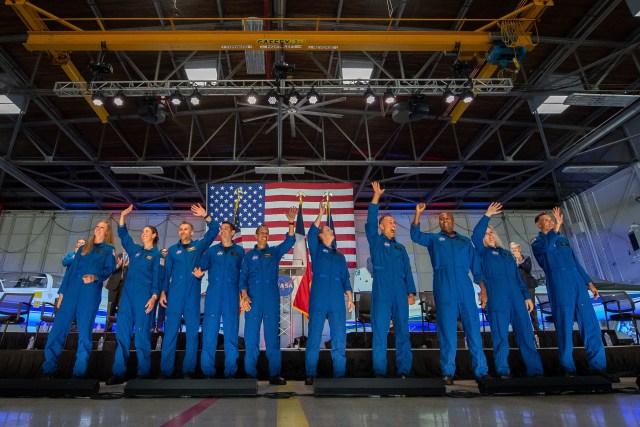
Diez maneras en que los estudiantes pueden prepararse para ser astronautas
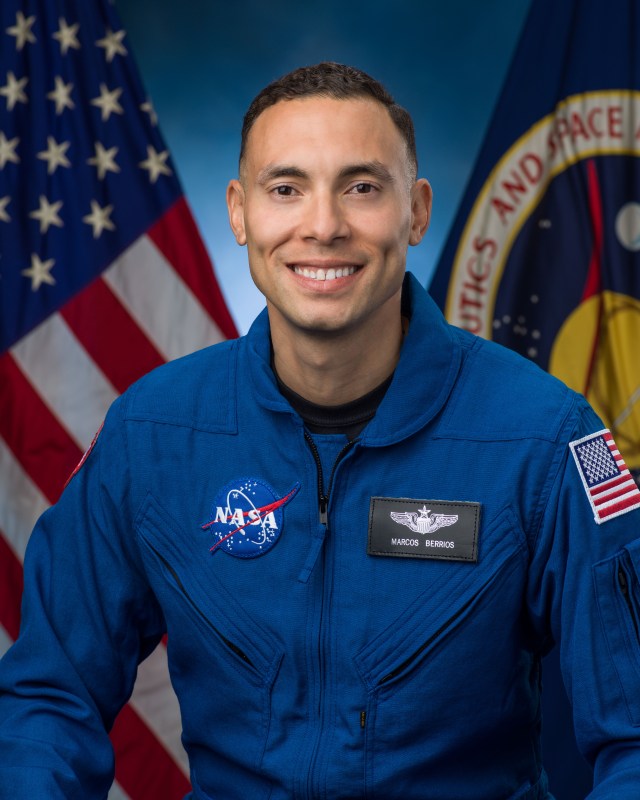
Astronauta de la NASA Marcos Berríos
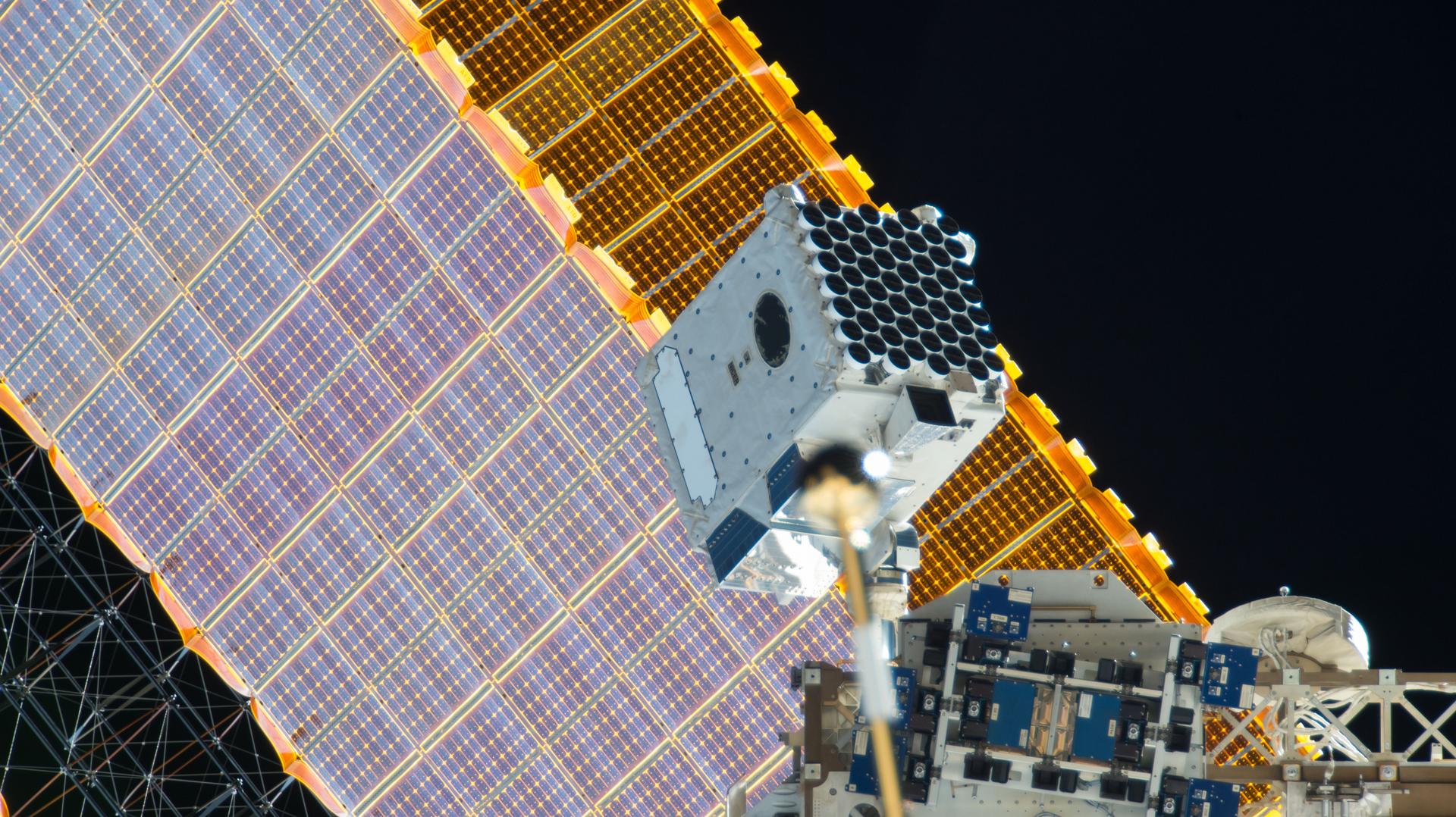
Resultados científicos revolucionarios en la estación espacial de 2023
Nasa goddard involved in new horizons from start.
William Steigerwald
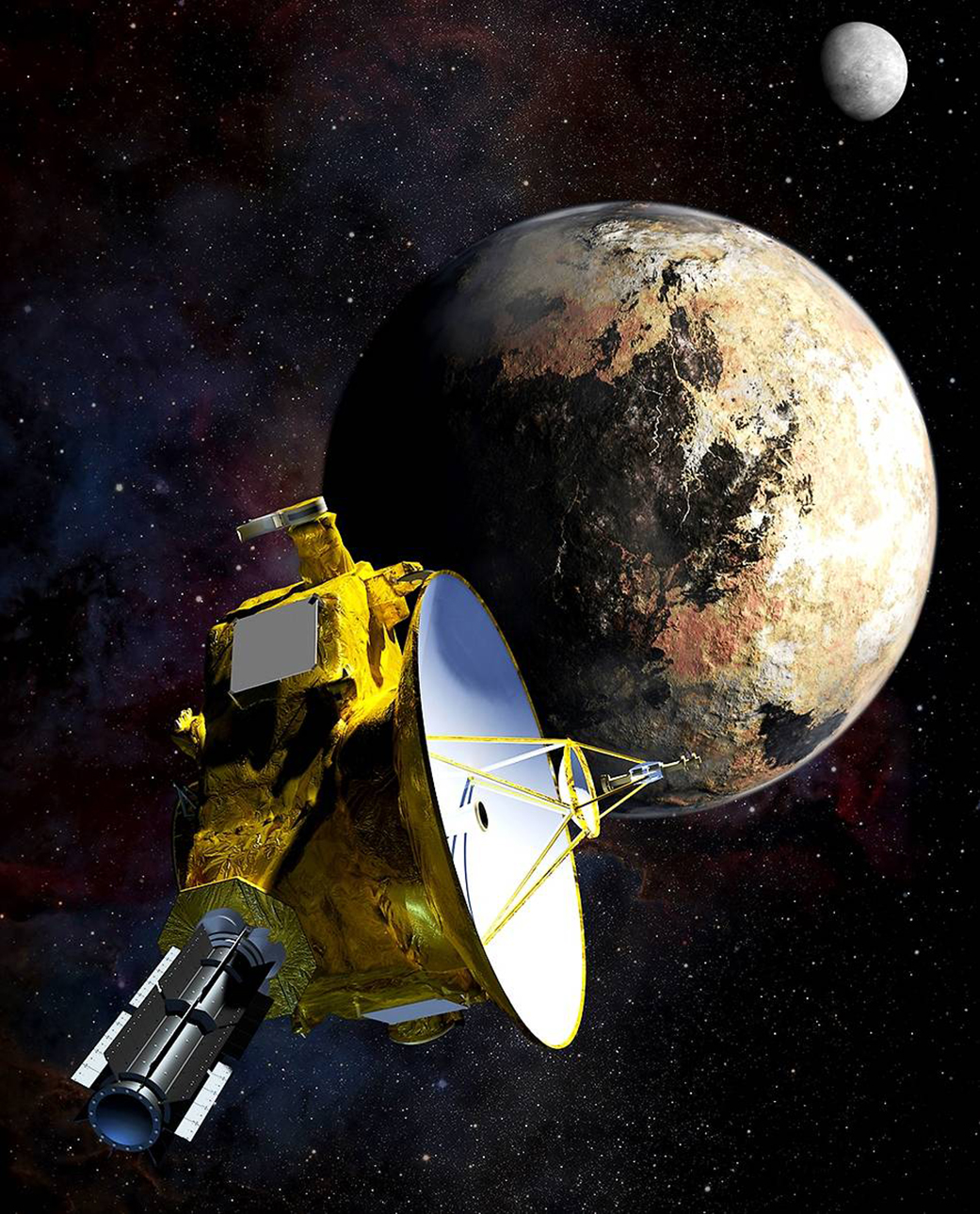
Three billion miles of space travel and 26 years of careful planning and execution ago, New Horizons Principal Investigator Alan Stern, now of the Southwest Research Institute in Boulder, Colorado, gave his first talk about a theoretical mission to Pluto at the weekly science symposium at NASA’s Goddard Space Flight Center in Greenbelt, Maryland.
“I remember thinking it was a wild idea,” said New Horizons Co-Investigator Don Jennings, of Goddard, who attended that talk in May of 1989. “But it got me to pay attention.”
Goddard scientists and engineers played a large role throughout the extended process of conceptualizing, developing and testing the New Horizons mission, which flew by the dwarf planet on Tuesday, July 14 after a nine-and-a-half-year flight.
“In a real sense this all got its start at Goddard,” Stern said.
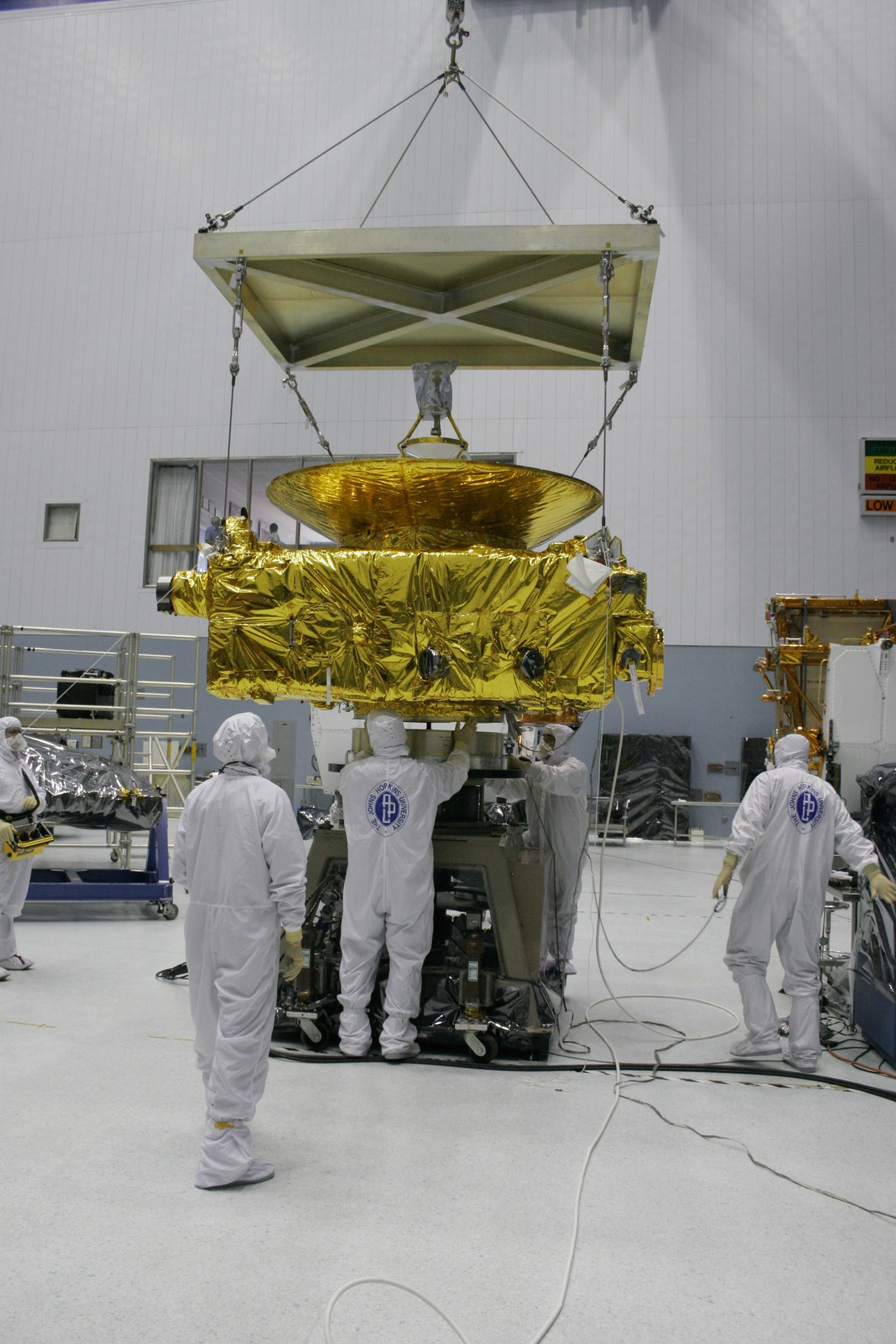
Scientists here also developed the Linear Etalon Imaging Spectral Array (LEISA) component of the Ralph instrument. Ralph/LEISA is an infrared camera designed to identify and map the composition of Pluto and its moons.
Ralph, the main eyes of New Horizons, will use two components for mapping – the Multispectral Visible Imaging Camera (Ralph/MVIC) and LEISA. MVIC will make maps in both visible and near infrared light, while LEISA will map strictly in near infrared. Near infrared light waves are slightly longer than what humans can see. The instrument took images as it approached, flew by and looked back at Pluto. The best images from this instrument will begin arriving in the early fall, according to Goddard’s Dennis Reuter, the Ralph Instrument Project Scientist
“The goal of the mission is to understand what the Pluto system is made of, how it evolved and what processes are going on now,” Reuter, who was also a co-investigator for the mission, said. “To do that you need to have good, high-resolution black-and-white and color images and infrared maps. These allow the science team to link what the surface is made of, what’s happened on the surface, and what’s happening there now. Ralph provides this capability.”
In addition to developing LEISA, Goddard performed the acoustic, spin balance and thermal vacuum testing on the spacecraft to prepare it for the rigors of spaceflight.
The National Academy of Science has kept the exploration of Pluto and other Kuiper belt objects as a top priority for NASA because their pristine nature could reveal clues about the ancient solar system. Taking high-definition color photos of the small bodies is an essential part of this goal so scientists can study them in detail.
LEISA itself has a very simple design, Reuter said. It uses an ultra-light spectrometer — roughly one square centimeter — to split up light waves and identify the composition of the materials it’s studying. Every material absorbs and reemits different wavelengths of light, which LEISA is able to recognize. Reuter and Jennings developed the technology for the spectrometer in 1992 for a NASA Advanced Technology Insertion competition, in which people were proposing instruments for Pluto missions. The other component of LEISA is a detector, which reads what the spectrometer is taking in.
Lightweight instruments were essential to the New Horizons spacecraft because it is the fastest mission NASA has ever launched out of Earth, shooting through space at roughly 36,000 miles per hour. The grand piano-sized spacecraft approached Pluto at about 31,000 miles per hour. The entire Ralph instrument is roughly the size of a hatbox.
The LEISA spectrometer flying on Ralph is made of sapphire with several hundred super-thin layers of different materials on top. These layers give the spectrometer the power to spread out the light coming in, the same way a prism does. It will look at all parts of Pluto and its moons that the sun illuminates, mapping the amounts of nitrogen, methane, carbon monoxide, frozen water and other materials, including organic compounds. It will also map surface temperatures. MVIC will look at the sun-lit parts of the bodies, but additionally map the night side of Pluto, which is illuminated by “Charon light.” Charon light is light reflected from Charon onto the dwarf planet.
“[LEISA and MVIC] are completely integral to doing the mission,” Stern said. “Ralph is all over the exploration objectives, maybe more than any other single instrument.”
As they study the surfaces of the different bodies, LEISA and MVIC complement the rest of the mission’s instruments in different ways as well. Alice, the UV spectrometer, for example, looks at the atmosphere, which has molecules coming from the surface. LORRI (Long Range Reconnaissance Imager), the high-resolution camera, will help scientists connect the geology of the bodies with the composition. The Solar Wind At Pluto (SWAP) instrument will look at solar wind interactions with the atmospheres of Charon and Pluto. The Pluto Energetic Particle Spectrometer Science Investigation (PEPSSI) will measure the escape of molecules from Pluto’s atmosphere and REX (Radio Science Experiment) will investigate the structure of the atmosphere and the temperature of the surface. The data from these instruments, when combined with the Ralph data, will provide a comprehensive picture of the Pluto system.
“It is very exciting to be part of this adventure,” Jennings said. “Of course, all of us are part of it. Everyone gets to see Pluto almost as soon as the images reach Earth. And it’s hard to believe that something I helped build is doing its job 3 billion miles away.”
For more information about New Horizons, visit:
www.nasa.gov/newhorizons
Elaine Hunt NASA’s Goddard Space Flight Center , Greenbelt, Md.
Spatial Variations of the Activity of 137 Cs and the Contents of Heavy Metals and Petroleum Products in the Polluted Soils of the City of Elektrostal
- DEGRADATION, REHABILITATION, AND CONSERVATION OF SOILS
- Open access
- Published: 15 June 2022
- Volume 55 , pages 840–848, ( 2022 )
Cite this article
You have full access to this open access article
- D. N. Lipatov 1 ,
- V. A. Varachenkov 1 ,
- D. V. Manakhov 1 ,
- M. M. Karpukhin 1 &
- S. V. Mamikhin 1
1458 Accesses
2 Citations
Explore all metrics
The levels of specific activity of 137 Cs and the contents of mobile forms (1 M ammonium acetate extraction) of heavy metals (Zn, Cu, Ni, Co, Cr, Pb) and petroleum products were studied in the upper soil horizon of urban landscapes of the city of Elektrostal under conditions of local radioactive and chemical contamination were studied. In the soils within a short radius (0–100 m) around the heavy engineering plant, the specific activity of 137 Cs and the contents of mobile forms of Pb, Cu, and Zn were increased. The lognormal distribution law of 137 Cs was found in the upper (0–10 cm) soil layer; five years after the radiation accident, the specific activity of 137 Cs varied from 6 to 4238 Bq/kg. The coefficients of variation increased with an increase in the degree of soil contamination in the following sequence: Co < Ni < petroleum products < Cr < 137 Cs < Zn < Pb < Cu ranging from 50 to 435%. Statistically significant direct correlation was found between the specific activity of 137 Cs and the contents of mobile forms of Pb, Cu, and Zn in the upper horizon of urban soils, and this fact indicated the spatial conjugacy of local spots of radioactive and polymetallic contamination in the studied area. It was shown that the specific activity of 137 Cs, as well as the content of heavy metals and petroleum products in the upper layer (0–10 cm) of the soils disturbed in the course of decontamination, earthwork and reclamation is reduced.
Similar content being viewed by others
Accumulation and migration of heavy metals in soils of the rostov region, south of russia.
Olga S. Bezuglova, Sergey N. Gorbov, … Marina N. Dubinina
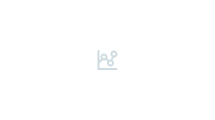
Geographical Features of Pollution of the Territory of Yakutia With Cesium-137
P. I. Sobakin, A. P. Chevychelov & Ya. R. Gerasimov
Activity Concentration of Natural Radionuclides and Total Heavy Metals Content in Soils of Urban Agglomeration
Avoid common mistakes on your manuscript.
INTRODUCTION
Contaminants migrate and accumulate in urban ecosystems under the impact of both natural and technogenic factors. The processes of technogenic migration of 137 Cs are most pronounced in radioactively contaminated territories. It was found in urboecological studies that the intensity of sedimentation of aerosol particles containing radionuclides and heavy metals is determined by the types of the surfaces of roofs, walls, roads, lawns, and parks and by their position within the urban wind field [ 12 , 26 ]. Traffic in the cities results in significant transport of dust and associated contaminants and radionuclides [ 15 , 24 ]. During decontamination measures in the areas of Chernobyl radioactive trace, not only the decrease in the level of contamination but also the possibility of secondary radioactive contamination because of the transportation of contaminated soil particles by wind or water, or anthropogenic transfer of transferring of ground were observed [ 5 , 6 ]. Rainstorm runoff and hydrological transport of dissolved and colloidal forms of 137 Cs can result in the accumulation of this radionuclide in meso- and microdepressions, where sedimentation takes place [ 10 , 16 ]. Different spatial distribution patterns of 137 Cs in soils of particular urban landscapes were found in the city of Ozersk near the nuclear fuel cycle works [ 17 ]. Natural character of 137 Cs migration in soils of Moscow forest-parks and a decrease in its specific activity in industrial areas have been revealed [ 10 ]. Determination of the mean level and parameters of spatial variations of 137 Cs in soils is one of primary tasks of radioecological monitoring of cities, including both unpolluted (background) and contaminated territories.
Emissions and discharges from numerous sources of contamination can cause the accumulation of a wide range of toxicants in urban soils: heavy metals (HMs), oil products (OPs), polycyclic aromatic hydrocarbons (PAHs), and other chemical substances. Soil contamination by several groups of toxicants is often observed in urban landscapes [ 20 , 23 ] because of the common contamination source or close pathways of the migration of different contaminants. A comprehensive analysis of contamination of urban soils by radionuclides and heavy metals has been performed in some studies [ 21 , 25 ]. The determination of possible spatial interrelationships between radioactive and chemical contaminations in urban soils is an important problem in urban ecology.
A radiation accident took place in the Elektrostal heavy engineering works (EHEW) in April 2013: a capacious source of 137 Cs entered the smelt furnace, and emission of radioactive aerosols from the aerating duct into the urban environment took place. The activity of molten source was estimated at about 1000–7000 Ci [ 14 ]. The area of contamination in the territory of the plant reached 7500 m 2 . However, radioactive aerosols affected a much larger area around the EHEW, including Krasnaya and Pervomaiskaya streets, and reached Lenin Prospect.
Geochemical evaluation of contamination of the upper soil horizon in the city of Elektrostal was carried out in 1989–1991. This survey indicated the anomalies of concentrations of wolfram, nickel, molybdenum, chromium, and other heavy metals related to accumulation of alloying constituent and impurities of non-ferrous metals in the emissions of steelmaking works [ 19 ].
The aim of our work was to determine the levels of specific activity of 137 Cs, concentrations of mobile forms of heavy metals (Zn, Cu, Ni, Co, Cr, and Pb) and oil products in the upper soil horizons in different urban landscapes of the city of Elektrostal under the conditions of local radioactive and chemical contamination.
Author information
Authors and affiliations.
Lomonosov Moscow State University, 119991, Moscow, Russia
D. N. Lipatov, V. A. Varachenkov, D. V. Manakhov, M. M. Karpukhin & S. V. Mamikhin
You can also search for this author in PubMed Google Scholar
Corresponding author
Correspondence to D. N. Lipatov .
Ethics declarations
The authors declare that they have no conflicts of interest.
Additional information
Translated by T. Chicheva
Rights and permissions
Open Access. This article is licensed under a Creative Commons Attribution 4.0 International License, which permits use, sharing, adaptation, distribution and reproduction in any medium or format, as long as you give appropriate credit to the original author(s) and the source, provide a link to the Creative Commons license, and indicate if changes were made. The images or other third party material in this article are included in the article’s Creative Commons license, unless indicated otherwise in a credit line to the material. If material is not included in the article’s Creative Commons license and your intended use is not permitted by statutory regulation or exceeds the permitted use, you will need to obtain permission directly from the copyright holder. To view a copy of this license, visit http://creativecommons.org/licenses/by/4.0/ .
Reprints and permissions
About this article
Lipatov, D.N., Varachenkov, V.A., Manakhov, D.V. et al. Spatial Variations of the Activity of 137 Cs and the Contents of Heavy Metals and Petroleum Products in the Polluted Soils of the City of Elektrostal. Eurasian Soil Sc. 55 , 840–848 (2022). https://doi.org/10.1134/S1064229322060072
Download citation
Received : 21 October 2021
Revised : 22 December 2021
Accepted : 30 December 2021
Published : 15 June 2022
Issue Date : June 2022
DOI : https://doi.org/10.1134/S1064229322060072
Share this article
Anyone you share the following link with will be able to read this content:
Sorry, a shareable link is not currently available for this article.
Provided by the Springer Nature SharedIt content-sharing initiative
- urban soils
- urban ecosystems
- radiation monitoring
- decontamination
- Urban Technosols
- Find a journal
- Publish with us
- Track your research
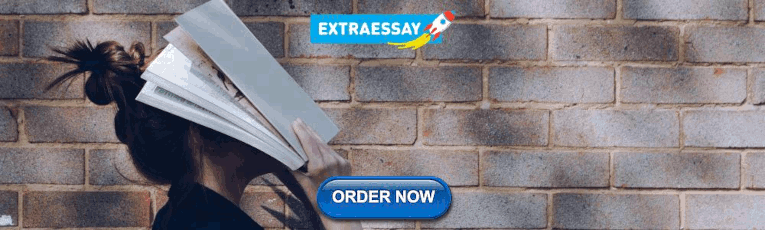
IMAGES
VIDEO
COMMENTS
FAIR data enabling new horizons for materials research. Nature 604 , 635-642 ( 2022) Cite this article. The prosperity and lifestyle of our society are very much governed by achievements in ...
New Horizons was the first spacecraft to explore Pluto and its five moons up close and, later, made the first close exploration of a Kuiper Belt Object. ... These observations were designed to gather new data on Jupiter's atmosphere, ring system, and moons (building on research from Galileo) and to test out New Horizon's instruments.
Sep 29, 2023. Article. NASA has announced an updated plan to continue New Horizons' mission of exploration of the outer solar system. Beginning in fiscal year 2025, New Horizons will focus on gathering unique heliophysics data, which can be readily obtained during an extended, low-activity mode of operations. While the science community is ...
New Horizons is an interplanetary space probe launched as a part of NASA 's New Frontiers program. [5] Engineered by the Johns Hopkins University Applied Physics Laboratory (APL) and the Southwest Research Institute (SwRI), with a team led by Alan Stern, [6] the spacecraft was launched in 2006 with the primary mission to perform a flyby study ...
Landscapes on the Edge: New Horizons for Research on Earth's Surface. Chemical, physical, biotic, and human processes constantly reshape Earth's surface from particles to continents. These processes form a complex network of interactions and feedbacks, but these interplays are not well understood, and challenging questions face science and ...
New Horizons reaches the 50 AU mark on April 18, 2021, and will join Voyagers 1 and 2 in interstellar space in the 2040s. NASA/Johns Hopkins APL/Southwest Research Institute. "That's a hauntingly beautiful image to me," said Alan Stern, New Horizons principal investigator from the Southwest Research Institute in Boulder, Colorado.
"This flyby is a historic achievement," said New Horizons Principal Investigator Alan Stern of the Southwest Research Institute in Boulder, Colorado. "Never before has any spacecraft team tracked down such a small body at such high speed so far away in the abyss of space. New Horizons has set a new bar for state-of-the-art spacecraft navigation."
And that was only the beginning. 1. Natural-color view of Pluto and its large moon Charon, compiled from images taken by NASA's New Horizons spacecraft on July 13 and 14, 2015. 2. Illustration of Sputnik Planitia at Pluto. 3. Illustration of the interior structure of Sputnik Planitia at Pluto.
New Worlds, New Horizons in Astronomy and Astrophysics recommends a balanced and executable program that will support research surrounding the most profound questions about the cosmos. The discoveries ahead will facilitate the search for habitable planets, shed light on dark energy and dark matter, and aid our understanding of the history of ...
The Johns Hopkins Applied Physics Laboratory in Laurel, Maryland, designed, built and operates the New Horizons spacecraft, and manages the mission for NASA's Science Mission Directorate. The Southwest Research Institute, based in San Antonio, leads the science team, payload operations and encounter science planning.
July 14, 2022 -- Seven years ago today, NASA's New Horizons spacecraft made history with the first up-close exploration of the Pluto system - providing breathtaking views and detailed data on Pluto and its largest moon, Charon, revealing the surfaces of these distant, mysterious worlds at the outer reaches of our solar system. These simulated flights over Pluto and Charon include some of the ...
New Horizons in Health: An Integrative Approach. Washington (DC): National Academies Press (US); 2001. New Horizons in Health: An Integrative Approach. ... psychological, and social precursors to disease. This priority is intended to broaden the time horizons that guide research on disease etiology as well as underscore agendas that may lead to ...
Founded in 2009, New Horizon Research Center has established itself as one of Florida's premier clinical research facilities.
Home Science Vol. 278, No. 5346 New Research Horizons. Back To Vol. 278, No. 5346. Full access. News. Share on. New Research Horizons. Science. 19 Dec 1997. Vol 278, Issue 5346. p. 2040. ... New predictions for this winter have been posted, from warmth in Minnesota to drought in southern Africa. Such seasonal forecasts pale before the new ...
"The New Horizons experiment provides the largest parallax baseline ever made — over 4 billion miles — and is the first demonstration of an easily observable stellar parallax," said Tod Lauer, New Horizons science team member from the National Science Foundation's National Optical-Infrared Astronomy Research Laboratory who coordinated ...
Clinical research is the study of new pharmaceutical therapies or medical devices that are designed to improve the treatment of a specific disease. When promising new drugs or devices appear, New Horizon Research Group participates in highly specific clinical trials to evaluate these products.
NASA's New Horizons - the fastest-ever spacecraft at launch - left Earth in 2006 and hurtled through the void at nearly one million miles per day toward a mysterious world on the solar system's outer edge. Three billion miles (4.8 billion km) and 9 1/2 years later, the spacecraft flew-by its target: Pluto.
New Horizons Team Publishes First Research Paper in Science, Describing Numerous Pluto System Findings; News Releases . This high-resolution image captured by NASA's New Horizons spacecraft combines blue, red and infrared images taken by the Ralph/Multispectral Visual Imaging Camera (MVIC). The bright expanse is the western lobe of the ...
of muon multiplicities (from 3 to about 100) and zenith angles (from 30º to almost a horizon) have been analyzed. Phenomenological distributions of muon bundles in particle multiplicity for several zenith angle intervals and in zenith angle for 3 values of the minimal number of muons are presented in figure 2.
Fifty years ago, five scientists from UKAEA's Culham Laboratory made a trip to the Soviet Union that was to prove a pivotal moment in the quest for fusion en...
Three billion miles of space travel and 26 years of careful planning and execution ago, New Horizons Principal Investigator Alan Stern, now of the Southwest Research Institute in Boulder, Colorado, gave his first talk about a theoretical mission to Pluto at the weekly science symposium at NASA's Goddard Space Flight Center in Greenbelt, Maryland.
Arkady A. Karyakin's 141 research works with 7,763 citations and 7,028 reads, including: Educational problems in electrochemical thermodynamics
Abstract. The levels of specific activity of 137 Cs and the contents of mobile forms (1 M ammonium acetate extraction) of heavy metals (Zn, Cu, Ni, Co, Cr, Pb) and petroleum products were studied in the upper soil horizon of urban landscapes of the city of Elektrostal under conditions of local radioactive and chemical contamination were studied. In the soils within a short radius (0-100 m ...