
Enter search terms to find related medical topics, multimedia and more.
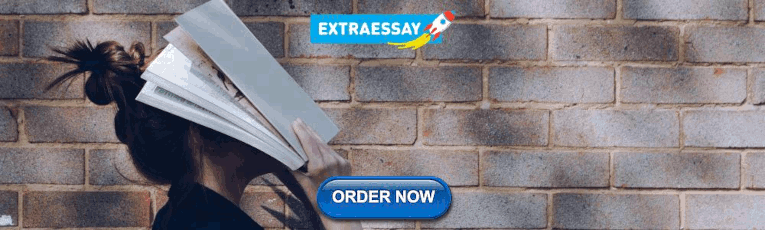
Advanced Search:
- Use “ “ for exact phrases.
- For example: “pediatric abdominal pain”
- Use – to remove results with certain keywords.
- For example: abdominal pain -pediatric
- Use OR to account for alternate keywords.
- For example: teenager OR adolescent
Metabolic Acidosis
, MD, Brookwood Baptist Health and Saint Vincent’s Ascension Health, Birmingham
- Symptoms and Signs
- 3D Models (0)

Metabolic acidosis is primary reduction in bicarbonate (HCO 3 − ), typically with compensatory reduction in carbon dioxide partial pressure (P co 2 ); pH may be markedly low or slightly subnormal. Metabolic acidoses are categorized as high or normal anion gap based on the presence or absence of unmeasured anions in serum. Causes include accumulation of ketones and lactic acid, renal failure, and drug or toxin ingestion (high anion gap) and gastrointestinal or renal HCO 3 − loss (normal anion gap). Symptoms and signs in severe cases include nausea and vomiting, lethargy, and hyperpnea. Diagnosis is clinical and with arterial blood gas (ABG) and serum electrolyte measurement. The cause is treated; IV sodium bicarbonate may be indicated when pH is very low.
(See also Acid-Base Regulation Acid-Base Regulation Metabolic processes in the human body continually produce acid and, to a lesser degree, base. Hydrogen ion (H + ) is especially reactive; it can attach to negatively charged proteins... read more and Acid-Base Disorders Acid-Base Disorders Acid-base disorders are pathologic changes in carbon dioxide partial pressure (Pco2) or serum bicarbonate (HCO3 − ) that typically produce abnormal arterial pH values. Acidemia is serum... read more .)
With metabolic acidosis, “acidosis” refers to a process that lowers blood pH below 7.35, and “metabolic” refers to the fact that it’s a problem caused by a decrease in the bicarbonate HCO3− concentration in the blood.
Normally, blood pH depends on the balance or ratio between the concentration of bases, mainly bicarbonate HCO3−, which increases the pH, and acids, mainly carbon dioxide CO2, which decrease the pH. The blood pH needs to be constantly between 7.35 and 7.45, and in addition the blood needs to remain electrically neutral, which means that the total cations, or positively charged particles, equals the total anions, or negatively charged particles.
Now, not all of the ions are easy or convenient to measure, so typically the dominant cation, sodium Na+, which is typically around 137 mEq/L and the two dominant anions, chloride Cl−, which is about 104 mEq/L, and bicarbonate HCO3−, which is around 24 mEq/L, are measured. The rest are unmeasured. So just counting up these three ions, there’s usually a difference, or “gap” between the sodium Na+ concentration and the sum of bicarbonate HCO3− and chloride Cl− concentrations in the plasma, which is 137 minus 128 (104 plus 24) or 9 mEq/L. This is known as the anion gap, and normally it ranges between 3 and 11 mEq/L. The anion gap largely represents unmeasured anions like organic acids and negatively charged plasma proteins, like albumin.
So, basically, metabolic acidosis arises either from the buildup of acid in our blood, which could be because it’s produced or ingested in increased amounts, or because the body can’t get rid of it, or from excessive bicarbonate HCO3− loss from the kidneys or gastrointestinal tract. The main problem with all of this is that they lead to a primary decrease in the concentration of bicarbonate HCO3− in the blood.
They can be broken down to two categories, based on whether the anion gap is high or normal. So, the first category of metabolic acidosis is a high anion gap metabolic acidosis. In this case, the bicarbonate HCO3− ion concentration decreases by binding of bicarbonate HCO3− ions and protons H+, which results in the formation of H2CO3 carbonic acid, which subsequently breaks down into carbon dioxide CO2 and water H2O. These protons can come from organic acids which have accumulated in the blood, but they can also come from increased production in our body. One such example is lactic acidosis, which is where decreased oxygen delivery to the tissues leads to increased anaerobic metabolism and the buildup of lactic acid. Another example is diabetic ketoacidosis, which can occurs in uncontrolled diabetes mellitus, where the lack of insulin forces cells to use fats as primary energy fuel instead of glucose. Fats are then converted to ketoacids, such as acetoacetic acid and β-hydroxybutyric acid. Another way acids can build up in our blood is due to an inability of the kidneys to throw them away, although they are produced in normal amounts. This can happen in cases of chronic renal failure, in which organic acids such as uric acid or sulfur- containing amino acids can accumulate because they aren’t excreted normally.
In other cases, organic acids don’t come from inside our bodies at all, but, instead, they are accidentally ingested. These include oxalic acid which can build up after an accidental ingestion of ethylene glycol, which is a common antifreeze, formic acid, which is a metabolite of methanol, a highly toxic alcohol, or hippuric acid, which comes from toluene, which is found in paint and glue. All of these organic acids have protons, and at a physiologic pH, these organic acids dissociate into protons H+ and corresponding organic acid anions. The protons H+ attach to bicarbonate HCO3− ions floating around, decreasing its plasma concentration and shifting the pH towards the acidic range. The key is that the plasma maintains its electroneutrality, because for each new negatively charged organic acid anions, there’s one less bicarbonate HCO3− ion, and because the organic acid anions are not part of the anion gap equation, the anion gap will be high.
In contrast, in other cases of metabolic acidosis, the decrease in bicarbonate HCO3− ions is offset by the buildup of Cl- ions which are part of the anion gap equation, so the anion gap remains normal. The most common cause is severe diarrhea, where bicarbonate- rich intestinal and pancreatic secretions rush through the gastrointestinal tract before they can be reabsorbed.
Another cause is type 2 renal tubular acidosis, which is the most common type of renal tubular acidosis, and develops because the proximal convoluted tubule, a part of the nephron, is unable to reabsorb bicarbonate HCO3−. Other types of renal tubular acidosis also result in normal anion gap metabolic acidosis, but the underlying mechanism is an inability to excrete protons H+ in the urine. The excessive loss of bicarbonate HCO3− results in a low plasma bicarbonate HCO3− concentration, which lowers the pH. In response, the kidneys start reabsorbing more chloride Cl- anions, so for each bicarbonate HCO3− ion that’s lost, there’s a new chloride Cl- anion. This is why normal anion gap metabolic acidosis is sometimes called a hyperchloremic metabolic acidosis.
Now, if there’s a decrease in the HCO3− concentration in the blood, threatening to decrease blood pH, the body has a number of important mechanisms to help keep the pH in balance. One of them is moving hydrogen ions out of the blood and into cells. To accomplish this, cells usually need to exchange the hydrogen ion for a potassium ion, using a special ion transporter located across the cell membrane. So, in order to help compensate for an acidosis, hydrogen ions enter cells and potassium ions leave the cells and enter the blood. This might help with the acidosis, but it results in hyperkalemia. In cases, though, when there’s a metabolic acidosis from excess organic acids, like lactic acid and ketoacids, protons can enter cells with the organic anion rather than having to be exchanged for potassium ions.
Another important regulatory mechanism involves the respiratory system, and begins with chemoreceptors that are located in the walls of the carotid arteries and in the wall of the aortic arch. These chemoreceptors start to fire when the pH falls, and that notifies the respiratory centers in the brainstem that they need to increase the respiratory rate and depth of breathing. As the respiratory rate and depth of each breath increase, the minute ventilation increases - that’s the volume of air that moves in and out of the lungs in a minute. The increased ventilation, helps move more carbon dioxide CO2 out of the body, reducing the PCO2 in the body, which increases the pH.
An additional mechanism, is that if metabolic acidosis is not caused by some renal problem, then several days later, the kidneys usually correct the imbalance. The kidneys excrete more hydrogen ions, while also, reabsorbing bicarbonate HCO3− so that it’s not lost in the urine.
All right, as a quick recap, metabolic acidosis caused by a decreased bicarbonate HCO3− concentration in the blood. It can be classified into high anion gap cases, which are caused by the accumulation of organic acids, either due to their increased production in the body, decreased excretion or exogenous ingestion, and normal anion gap cases, which are caused directly by a loss of bicarbonate HCO3−, as in diarrhea or type 2 renal tubular acidosis.
Metabolic Acidosis (https://www.youtube.com/watch?v=vf99lYkJRnE&list=PLY33uf2n4e6PT53f0Z5LmFHo7Vb0ljn5b&index=5&t=23s) by Osmosis (https://open.osmosis.org/) is licensed under CC-BY-SA 4.0 (https://creativecommons.org/licenses/by-sa/4.0/).
Etiology of Metabolic Acidosis
Metabolic acidosis is acid accumulation due to
Increased acid production or acid ingestion
Decreased acid excretion
Gastrointestinal or renal HCO 3 − loss
Acidemia (arterial pH < 7.35) results when acid load overwhelms respiratory compensation. Causes are classified by their effect on the anion gap Calculation of the anion gap Acid-base disorders are pathologic changes in carbon dioxide partial pressure (Pco2) or serum bicarbonate (HCO3 − ) that typically produce abnormal arterial pH values. Acidemia is serum... read more (see table ).

High anion gap acidosis
The most common causes of a high anion gap metabolic acidosis are
Ketoacidosis
Lactic acidosis
Renal failure
Toxic ingestions
Ketoacidosis is a common complication of type 1 diabetes mellitus (see diabetic ketoacidosis Diabetic Ketoacidosis (DKA) Diabetic ketoacidosis (DKA) is an acute metabolic complication of diabetes characterized by hyperglycemia, hyperketonemia, and metabolic acidosis. Hyperglycemia causes an osmotic diuresis with... read more ), but it also occurs with chronic alcohol use disorder (see alcoholic ketoacidosis Alcoholic Ketoacidosis Alcoholic ketoacidosis is a metabolic complication of alcohol use and starvation characterized by hyperketonemia and anion gap metabolic acidosis without significant hyperglycemia. Alcoholic... read more ), undernutrition Overview of Undernutrition Undernutrition is a form of malnutrition. (Malnutrition also includes overnutrition.) Undernutrition can result from inadequate ingestion of nutrients, malabsorption, impaired metabolism, loss... read more , and, to a lesser degree, fasting. In these conditions, the body converts from glucose metabolism to free fatty acid (FFA) metabolism; FFAs are converted by the liver into ketoacids, acetoacetic acid, and beta-hydroxybutyrate (all unmeasured anions). Ketoacidosis is also a rare manifestation of congenital isovaleric acidemia Isovaleric Acidemia Valine, leucine, and isoleucine are branched-chain amino acids; deficiency of enzymes involved in their metabolism leads to accumulation of organic acids with severe metabolic acidosis. There... read more or congenital methylmalonic acidemia Methylmalonic Acidemia Valine, leucine, and isoleucine are branched-chain amino acids; deficiency of enzymes involved in their metabolism leads to accumulation of organic acids with severe metabolic acidosis. There... read more , which are rare disorders that involve the abnormal metabolism of amino acids.
Lactic acidosis Lactic Acidosis Lactic acidosis is a high anion gap metabolic acidosis due to elevated blood lactate. Lactic acidosis results from overproduction of lactate, decreased metabolism of lactate, or both. (See also... read more is the most common cause of metabolic acidosis in hospitalized patients. Lactate accumulation results from a combination of excess formation and decreased metabolism of lactate. Excess lactate production occurs during states of anaerobic metabolism. The most serious form occurs during the various types of shock. Decreased metabolism generally occurs with hepatocellular dysfunction resulting from decreased liver perfusion or as a part of generalized shock. Diseases and medications that impair mitochondrial function can cause lactic acidosis.
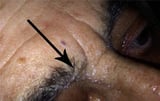
Toxins may have acidic metabolites or trigger lactic acidosis.
Rhabdomyolysis Rhabdomyolysis Rhabdomyolysis is a clinical syndrome involving the breakdown of skeletal muscle tissue. Symptoms and signs include muscle weakness, myalgias, and reddish-brown urine, although this triad is... read more is a rare cause of metabolic acidosis thought to be due to release of protons and anions directly from muscle.
Normal anion gap acidosis
The most common causes of normal anion gap acidosis are
Gastrointestinal (GI) or renal HCO 3 − loss
Impaired renal acid excretion
Normal anion gap metabolic acidosis is also called hyperchloremic acidosis because the kidneys reabsorb chloride (Cl − ) instead of reabsorbing HCO 3 − .
Many GI secretions are rich in HCO 3 − (eg, biliary, pancreatic, and intestinal fluids); loss due to diarrhea, tube drainage, or fistulas can cause acidosis. In ureterosigmoidostomy (insertion of ureters into a section of sigmoid colon after obstruction or cystectomy), the colon secretes and loses HCO 3 − in exchange for urinary chloride (Cl − ) and absorbs urinary ammonium, which dissociates into ammonia (NH 3 + ) and hydrogen ion (H + ).
Ion-exchange resin uncommonly causes HCO 3 − loss by binding HCO 3 − .
The renal tubular acidoses Renal Tubular Acidosis Renal tubular acidosis (RTA) is acidosis and electrolyte disturbances due to impaired renal hydrogen ion excretion (type 1), impaired bicarbonate resorption (type 2), or abnormal aldosterone... read more impair either H + secretion (types 1 and 4) or HCO 3 − absorption (type 2). Impaired acid excretion and a normal anion gap also occur in early renal failure, tubulointerstitial renal disease, and when carbonic anhydrase inhibitors (eg, acetazolamide ) are taken.
Symptoms and Signs of Metabolic Acidosis
Symptoms and signs (see table ) are primarily those of the cause.
Mild acidemia is itself asymptomatic. More severe acidemia (pH < 7.10) may cause nausea, vomiting, and malaise. Symptoms may occur at higher pH if acidosis develops rapidly.
The most characteristic sign is hyperpnea (long, deep breaths at a normal rate), reflecting a compensatory increase in alveolar ventilation; this hyperpnea is not accompanied by a feeling of dyspnea.
Pearls & Pitfalls
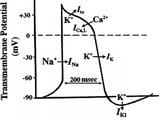
Diagnosis of Metabolic Acidosis
Arterial blood gas (ABG) and serum electrolyte measurement
Anion gap and delta gap calculated
Winters formula for calculating compensatory changes
Evaluation for cause
Recognition of metabolic acidosis and appropriate respiratory compensation are discussed in Diagnosis of Acid-Base Disorders Diagnosis Acid-base disorders are pathologic changes in carbon dioxide partial pressure (Pco2) or serum bicarbonate (HCO3 − ) that typically produce abnormal arterial pH values. Acidemia is serum... read more . Determining the cause of metabolic acidosis begins with the anion gap.
The cause of an elevated anion gap may be clinically obvious (eg, hypovolemic shock, missed hemodialysis), but if not, blood testing should include
BUN (blood urea nitrogen)
Possible toxins
Salicylate levels can be measured in most laboratories, but methanol and ethylene glycol frequently cannot; their presence may be suggested by presence of an osmolar gap.
Calculated serum osmolarity (2 [sodium] + [glucose]/18 + BUN/2.8 + blood alcohol/5, based on conventional units) is subtracted from measured osmolarity. A difference > 10 implies the presence of an osmotically active substance, which, in the case of a high anion gap acidosis, is methanol or ethylene glycol. Although ingestion of ethanol may cause an osmolar gap and a mild acidosis, it should never be considered the sole cause of a significant metabolic acidosis.
If the serum anion gap is normal, urinary electrolytes are measured and the urinary anion gap is calculated as [sodium] + [potassium] – [chloride]. A normal urinary anion gap in a person without acidosis has a value near zero. If the urinary anion gap becomes negative, there are excess unmeasured positively charged ions in the urine, typically ammonium (NH4+), which is normally secreted by the kidneys in response to volume depletion. If the urinary anion gap in a patient with non-anion gap metabolic acidosis is -30 to -50 mEq/L (-30 to -50 mmol/L), the kidneys are responding to extra-renal volume losses typically from the GI tract. An elevation in urinary anion gap in such patients (a positive calculated result) is due to the loss of unmeasured negatively charged ions in the urine and suggests renal HCO 3 − loss, which most commonly occurs in renal tubular acidosis ( evaluation of renal tubular acidosis Diagnosis Renal tubular acidosis (RTA) is acidosis and electrolyte disturbances due to impaired renal hydrogen ion excretion (type 1), impaired bicarbonate resorption (type 2), or abnormal aldosterone... read more is discussed elsewhere).
In addition, when metabolic acidosis is present, a delta gap Calculation of the anion gap Acid-base disorders are pathologic changes in carbon dioxide partial pressure (Pco2) or serum bicarbonate (HCO3 − ) that typically produce abnormal arterial pH values. Acidemia is serum... read more is calculated to identify concomitant metabolic alkalosis Metabolic Alkalosis Metabolic alkalosis is primary increase in bicarbonate (HCO3 − ) with or without compensatory increase in carbon dioxide partial pressure (Pco2); pH may be high or nearly normal. Common... read more , and Winters formula is applied to determine whether respiratory compensation is appropriate or reflects a second acid-base disorder.
Treatment of Metabolic Acidosis
Cause treated
Sodium bicarbonate (NaHCO 3 ) primarily for severe acidemia—give with caution
Treatment is directed at the cause. Hemodialysis Hemodialysis In hemodialysis, a patient’s blood is pumped into a dialyzer containing 2 fluid compartments configured as bundles of hollow fiber capillary tubes or as parallel, sandwiched sheets of semipermeable... read more is required for renal failure and sometimes for ethylene glycol, methanol, or salicylate poisoning.
Treatment of acidemia with sodium bicarbonate (NaHCO 3 ) is clearly indicated only in certain circumstances and is probably deleterious in others. When metabolic acidosis results from loss of HCO 3 − or accumulation of inorganic acids (ie, normal anion gap acidosis), bicarbonate therapy is generally safe and appropriate. However, when acidosis results from organic acid accumulation (ie, high anion gap acidosis), bicarbonate therapy is controversial; it does not clearly decrease mortality in these conditions, and there are several possible risks.
With treatment of the underlying condition, lactate and ketoacids are metabolized back to HCO 3 − ; exogenous HCO 3 − loading may therefore cause an “overshoot” metabolic alkalosis. In any condition, sodium bicarbonate may also cause sodium and volume overload, hypokalemia, and, by inhibiting respiratory drive, hypercapnia. Furthermore, because HCO 3 − does not diffuse across cell membranes, intracellular acidosis is not corrected and may paradoxically worsen because some of the added HCO 3 − is converted to carbon dioxide (CO 2 ), which does cross into the cell and is hydrolyzed to H + and HCO 3 − . Clearance of additional CO 2 also requires adequate minute ventilation. Patients with compromised respiratory status due to underlying pulmonary disease may not be able meet the increased minute ventilation. Patients requiring mechanical ventilatory support Ventilatory Failure Ventilatory failure is a rise in PaCO2 (hypercapnia) that occurs when the respiratory load can no longer be supported by the strength or activity of the system. The most common causes are severe... read more will need appropriate ventilator settings to account for increased minute ventilation.
Despite these and other controversies, most experts recommend giving bicarbonate IV for severe metabolic acidosis (pH < 7.0) primarily because of concerns about worsening cardiovascular instability at lower pH values.
Treatment requires 2 calculations (same for both conventional and SI units). The first is the level to which HCO 3 − must be raised, calculated by the Kassirer-Bleich equation, using a target value for [H + ] of 79 nEq/L (79 nmol/L), which corresponds to a pH of 7.10:
79 = 24 × P co 2 /HCO 3 −
Desired HCO 3 − = 0.30 × P co 2
The amount of sodium bicarbonate needed to achieve that level is
NaHCO 3 required (mEq/mmol) = (desired [HCO 3 − ] − observed [HCO 3 − ]) × 0.4 × body weight (kg)
For example, a 70-kg man has severe metabolic acidosis with a pH of 6.92, P CO 2 of 40 mmHg, and HCO 3 − of 8 mEq/L (8 mmol/L). The target bicarbonate level needed to achieve a pH of 7.10 is 0.30 × 40 = 12 mEq/L (12 mmol/L). This level is 4 mEq/L (4 mmol/L) more than his current bicarbonate level of 8. To increase bicarbonate by 4, multiply 4 by 0.4 times 70 (the body weight), giving a result of 112 mEq (112 mmol) of HCO 3 − . This amount of sodium bicarbonate is given over several hours. Blood pH and HCO 3 − levels can be checked 30 minutes to 1 hour after administration, which allows for equilibration with extravascular HCO 3 − . There is no consensus regarding the concentration of bicarbonate solution to use, but it is important to realize that the typical 50-mL ampule of NaHCO 3 contain 50 mEq of sodium bicarbonate and can worsen volume overload and also cause hyperosmolality especially if multiple ampules are given over a short period time. Iso-osmotic formulations such as 75 mEq NaHCO 3 mixed with 1 L of 0.45% saline and 150 mEq NaHCO 3 mixed with 1 L of sterile water are also used.
Alternatives to sodium bicarbonate include
Lactate, either in the form of lactated Ringer's solution or sodium lactate (metabolized mEq for mEq to bicarbonate when liver function is normal)
Sodium acetate (metabolized mEq for mEq to bicarbonate when liver function is normal)
Tromethamine , an amino alcohol that buffers both metabolic (H + ) and respiratory (carbonic acid [H 2 CO 3 ]) acid
Carbicarb, an equimolar mixture of sodium bicarbonate and carbonate (the latter consumes CO 2 and generates HCO 3 − )
Dichloroacetate, which enhances oxidation of lactate
These alternatives do not offer a proven benefit over sodium bicarbonate alone and can cause complications of their own.
Potassium (K + ) depletion, common in metabolic acidosis, should be identified through frequent serum K + monitoring and treated as needed with oral or parenteral potassium chloride .
Metabolic acidosis can be caused by acid accumulation due to increased acid production or acid ingestion; decreased acid excretion; or gastrointestinal or renal bicarbonate (HCO 3 − ) loss.
Metabolic acidoses are categorized based on whether the anion gap is high or normal.
High anion gap acidoses are most often due to ketoacidosis, lactic acidosis, chronic kidney disease, or certain toxic ingestions.
Normal anion gap acidoses are most often due to gastrointestinal or renal HCO 3 − loss.
Calculate delta gap to identify concomitant metabolic alkalosis, and apply Winters formula to see whether respiratory compensation is appropriate or reflects a 2nd acid-base disorder.
Treat the cause.
Intravenous sodium bicarbonate (NaHCO 3 ) is indicated when acidosis is due to a change in HCO 3 − level (normal anion gap acidosis).
Intravenous sodium bicarbonate is controversial in high anion gap acidosis (but may be considered when pH < 7.00, with a target pH of ≥ 7.10).
Drugs Mentioned In This Article

Was This Page Helpful?

Test your knowledge
Brought to you by Merck & Co, Inc., Rahway, NJ, USA (known as MSD outside the US and Canada) — dedicated to using leading-edge science to save and improve lives around the world. Learn more about the Merck Manuals and our commitment to Global Medical Knowledge.
- Permissions
- Cookie Settings
- Terms of use
- Veterinary Manual

- IN THIS TOPIC
Learn how UpToDate can help you.
Select the option that best describes you
- Medical Professional
- Resident, Fellow, or Student
- Hospital or Institution
- Group Practice
- Patient or Caregiver
- Find in topic
RELATED TOPICS
INTRODUCTION
Renal excretion of acid involves the combination of hydrogen ions with urinary titratable acids, particularly phosphate (HPO 4 2- + H + → H 2 PO 4 - ), and ammonia to form ammonium (NH 3 + H + → NH 4 + ) [ 1 ]. The latter is the primary adaptive response since ammonia production from the metabolism of glutamine can be appropriately increased in response to an acid load [ 2 ].
Acid-base balance is usually assessed in terms of the bicarbonate-carbon dioxide buffer system:
Dissolved CO 2 + H 2 O ↔ H 2 CO 3 ↔ HCO 3 - + H +
The ratio between these reactants can be expressed by the Henderson-Hasselbalch equation. By convention, the pKa of 6.10 is used when the denominator is the concentration of dissolved CO 2 , and this is proportional to the pCO 2 (the actual concentration of the acid H 2 CO 3 is very low):
AKF spotlights
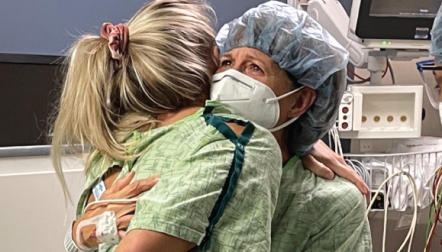
Learn about kidney donation and transplants
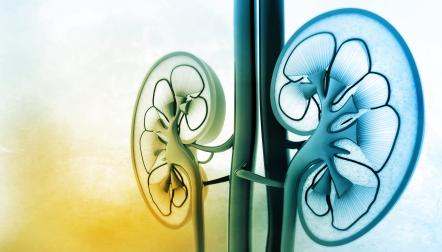
Learn how to get tested for kidney disease
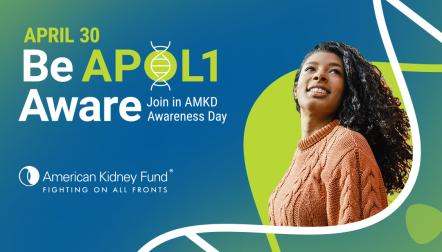
Learn about APOL1-mediated kidney disease
Metabolic acidosis – symptoms, complications & treatment.
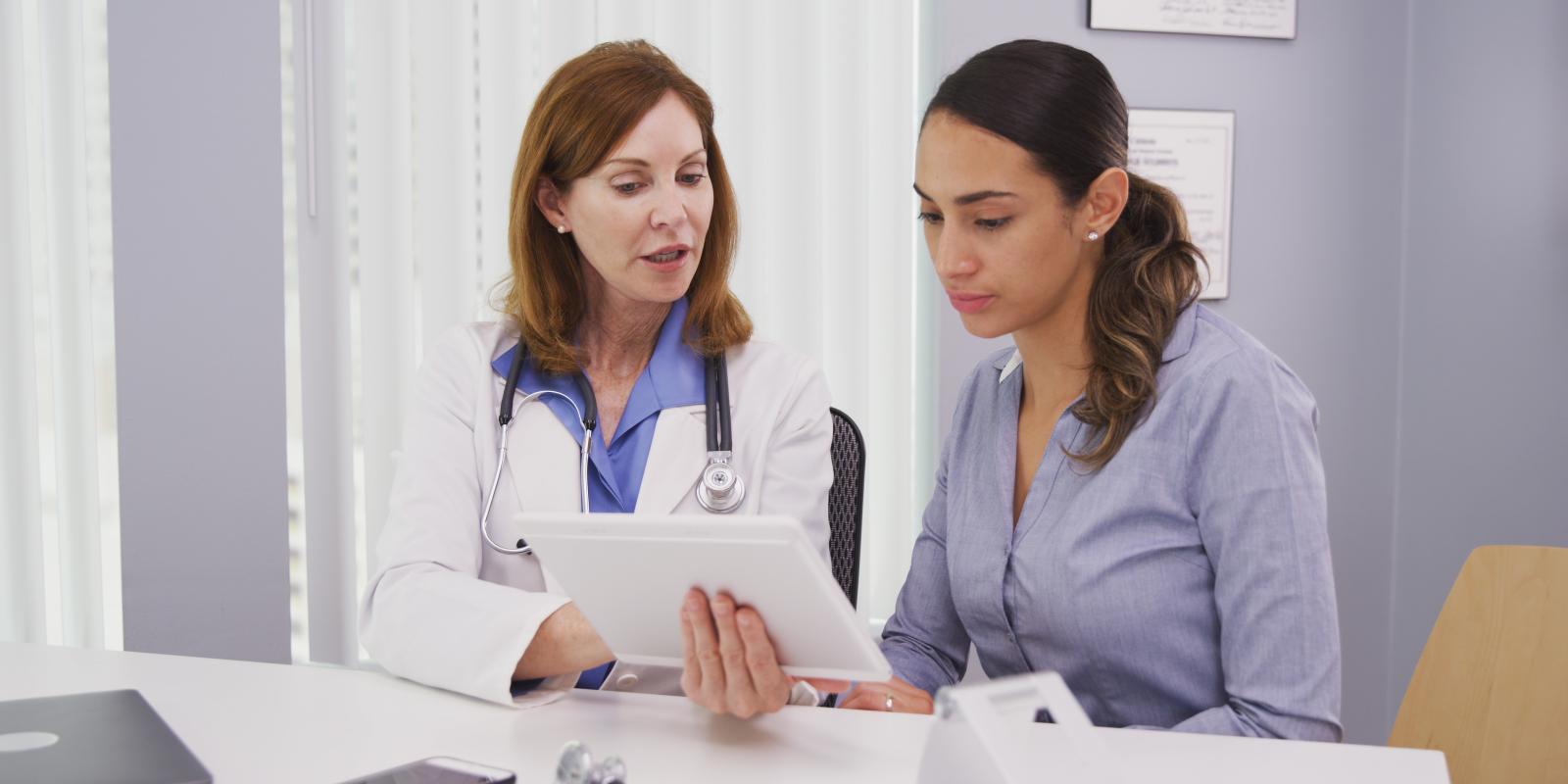
- {wysiwyg_placeholder}
Metabolic acidosis is a buildup of acid in your body. Your kidneys help keep the right balance of acids in your body. Your body can have too much acid for two main reasons:
- your kidneys are not balancing or getting rid of enough acid, or
- your body is making too much acid.
Metabolic acidosis is common in people with kidney disease because their kidneys are not filtering their blood well enough. It can also happen in people with diabetes or kidney failure.
Doctors will do blood and urine tests to find out if someone has metabolic acidosis.
What are the symptoms of metabolic acidosis?
Many people do not have symptoms, but some people may have:
- Fast heartbeat
- Feeling sick to your stomach
- Long and deep breaths
- Not wanting to eat
- Feeling tired
- Feeling weak
What problems can happen from metabolic acidosis?
Here are some health problems that can happen if metabolic acidosis is not treated:
- Your kidney disease can get worse.
- Bone loss (osteoporosis), which can lead to a higher chance of fractures in important bones like your hips or backbone.
- Muscle loss because of less protein in your body.
- Endocrine disorders, which mean that glands that produce hormones in your body are not working like they should.
- Slowed growth in children.
- Inflammation (swelling, redness, and pain).
- Amyloid accumulation, which is a build-up of protein in your body that can hurt your joints, organs and brain.
- Increased chance of death.
How is metabolic acidosis treated?
There are no FDA-approved therapies for long-term treatment of metabolic acidosis. However, some studies show the treatments below may help keep blood acid levels balanced.
Alkali therapy
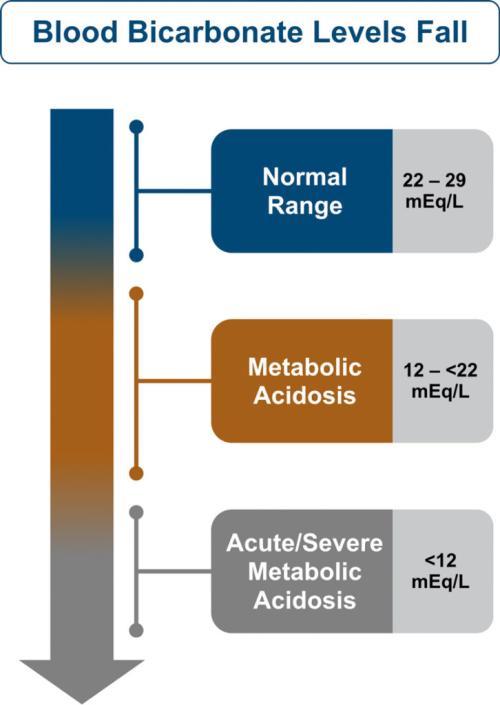
It is recommended to keep blood bicarbonate at levels above 22 mEq/L. The normal range is 22–29 mEq/L. People with metabolic acidosis have a blood bicarbonate level between 12–22 mEq/L, while people with severe metabolic acidosis fall below 12 mEq/L. There are medicines that can add more bicarbonate to your body to balance out the acid. One way to increase bicarbonate levels is through alkali therapy, which could include taking sodium bicarbonate. Your doctor could also suggest taking another similar supplement, such as calcium citrate, calcium carbonate, or calcium acetate.
Taking sodium bicarbonate introduces sodium (salt) into your body. The problem with this is that most people with later-stage chronic kidney disease have health problems that require them to limit sodium. This includes high blood pressure, heart disease, heart failure, or swelling. In some individuals, using sodium-based supplements could make health problems worse. They may have to take a different type of medication to help instead.
Sometimes there are side effects to sodium bicarbonate, including belching, bloating, flatulence, stomach pain, loss of appetite, feeling sick, and throwing up. You should not take sodium bicarbonate, or any other type of medicine to treat acid in your blood, without your doctor's approval.
Changes in what you eat
For people with metabolic acidosis, making changes in what they eat may help. For example, eating plant-based protein instead of animal-based protein may keep acid levels lower. Always talk to your doctor before you make any changes to your diet. Your doctor may also recommend that you see a registered dietitian who can help create a specific nutritional plan just for you.
Sign up for news, updates and information on events from AKF.

Enter search terms to find related medical topics, multimedia and more.
Advanced Search:
- Use “ “ for exact phrases.
- For example: “pediatric abdominal pain”
- Use – to remove results with certain keywords.
- For example: abdominal pain -pediatric
- Use OR to account for alternate keywords.
- For example: teenager OR adolescent
With metabolic acidosis, “acidosis” refers to a process that lowers blood pH below 7.35, and “metabolic” refers to the fact that it’s a problem caused by a decrease in the bicarbonate HCO3− concentration in the blood.
Normally, blood pH depends on the balance or ratio between the concentration of bases, mainly bicarbonate HCO3−, which increases the pH, and acids, mainly carbon dioxide CO2, which decrease the pH. The blood pH needs to be constantly between 7.35 and 7.45, and in addition the blood needs to remain electrically neutral, which means that the total cations, or positively charged particles, equals the total anions, or negatively charged particles.
Now, not all of the ions are easy or convenient to measure, so typically the dominant cation, sodium Na+, which is typically around 137 mEq/L and the two dominant anions, chloride Cl−, which is about 104 mEq/L, and bicarbonate HCO3−, which is around 24 mEq/L, are measured. The rest are unmeasured. So just counting up these three ions, there’s usually a difference, or “gap” between the sodium Na+ concentration and the sum of bicarbonate HCO3− and chloride Cl− concentrations in the plasma, which is 137 minus 128 (104 plus 24) or 9 mEq/L. This is known as the anion gap, and normally it ranges between 3 and 11 mEq/L. The anion gap largely represents unmeasured anions like organic acids and negatively charged plasma proteins, like albumin.
So, basically, metabolic acidosis arises either from the buildup of acid in our blood, which could be because it’s produced or ingested in increased amounts, or because the body can’t get rid of it, or from excessive bicarbonate HCO3− loss from the kidneys or gastrointestinal tract. The main problem with all of this is that they lead to a primary decrease in the concentration of bicarbonate HCO3− in the blood.
They can be broken down to two categories, based on whether the anion gap is high or normal. So, the first category of metabolic acidosis is a high anion gap metabolic acidosis. In this case, the bicarbonate HCO3− ion concentration decreases by binding of bicarbonate HCO3− ions and protons H+, which results in the formation of H2CO3 carbonic acid, which subsequently breaks down into carbon dioxide CO2 and water H2O. These protons can come from organic acids which have accumulated in the blood, but they can also come from increased production in our body. One such example is lactic acidosis, which is where decreased oxygen delivery to the tissues leads to increased anaerobic metabolism and the buildup of lactic acid. Another example is diabetic ketoacidosis, which can occurs in uncontrolled diabetes mellitus, where the lack of insulin forces cells to use fats as primary energy fuel instead of glucose. Fats are then converted to ketoacids, such as acetoacetic acid and β-hydroxybutyric acid. Another way acids can build up in our blood is due to an inability of the kidneys to throw them away, although they are produced in normal amounts. This can happen in cases of chronic renal failure, in which organic acids such as uric acid or sulfur- containing amino acids can accumulate because they aren’t excreted normally.
In other cases, organic acids don’t come from inside our bodies at all, but, instead, they are accidentally ingested. These include oxalic acid which can build up after an accidental ingestion of ethylene glycol, which is a common antifreeze, formic acid, which is a metabolite of methanol, a highly toxic alcohol, or hippuric acid, which comes from toluene, which is found in paint and glue. All of these organic acids have protons, and at a physiologic pH, these organic acids dissociate into protons H+ and corresponding organic acid anions. The protons H+ attach to bicarbonate HCO3− ions floating around, decreasing its plasma concentration and shifting the pH towards the acidic range. The key is that the plasma maintains its electroneutrality, because for each new negatively charged organic acid anions, there’s one less bicarbonate HCO3− ion, and because the organic acid anions are not part of the anion gap equation, the anion gap will be high.
In contrast, in other cases of metabolic acidosis, the decrease in bicarbonate HCO3− ions is offset by the buildup of Cl- ions which are part of the anion gap equation, so the anion gap remains normal. The most common cause is severe diarrhea, where bicarbonate- rich intestinal and pancreatic secretions rush through the gastrointestinal tract before they can be reabsorbed.
Another cause is type 2 renal tubular acidosis, which is the most common type of renal tubular acidosis, and develops because the proximal convoluted tubule, a part of the nephron, is unable to reabsorb bicarbonate HCO3−. Other types of renal tubular acidosis also result in normal anion gap metabolic acidosis, but the underlying mechanism is an inability to excrete protons H+ in the urine. The excessive loss of bicarbonate HCO3− results in a low plasma bicarbonate HCO3− concentration, which lowers the pH. In response, the kidneys start reabsorbing more chloride Cl- anions, so for each bicarbonate HCO3− ion that’s lost, there’s a new chloride Cl- anion. This is why normal anion gap metabolic acidosis is sometimes called a hyperchloremic metabolic acidosis.
Now, if there’s a decrease in the HCO3− concentration in the blood, threatening to decrease blood pH, the body has a number of important mechanisms to help keep the pH in balance. One of them is moving hydrogen ions out of the blood and into cells. To accomplish this, cells usually need to exchange the hydrogen ion for a potassium ion, using a special ion transporter located across the cell membrane. So, in order to help compensate for an acidosis, hydrogen ions enter cells and potassium ions leave the cells and enter the blood. This might help with the acidosis, but it results in hyperkalemia. In cases, though, when there’s a metabolic acidosis from excess organic acids, like lactic acid and ketoacids, protons can enter cells with the organic anion rather than having to be exchanged for potassium ions.
Another important regulatory mechanism involves the respiratory system, and begins with chemoreceptors that are located in the walls of the carotid arteries and in the wall of the aortic arch. These chemoreceptors start to fire when the pH falls, and that notifies the respiratory centers in the brainstem that they need to increase the respiratory rate and depth of breathing. As the respiratory rate and depth of each breath increase, the minute ventilation increases - that’s the volume of air that moves in and out of the lungs in a minute. The increased ventilation, helps move more carbon dioxide CO2 out of the body, reducing the PCO2 in the body, which increases the pH.
An additional mechanism, is that if metabolic acidosis is not caused by some renal problem, then several days later, the kidneys usually correct the imbalance. The kidneys excrete more hydrogen ions, while also, reabsorbing bicarbonate HCO3− so that it’s not lost in the urine.
All right, as a quick recap, metabolic acidosis caused by a decreased bicarbonate HCO3− concentration in the blood. It can be classified into high anion gap cases, which are caused by the accumulation of organic acids, either due to their increased production in the body, decreased excretion or exogenous ingestion, and normal anion gap cases, which are caused directly by a loss of bicarbonate HCO3−, as in diarrhea or type 2 renal tubular acidosis.
Metabolic Acidosis (https://www.youtube.com/watch?v=vf99lYkJRnE&list=PLY33uf2n4e6PT53f0Z5LmFHo7Vb0ljn5b&index=5&t=23s) by Osmosis (https://open.osmosis.org/) is licensed under CC-BY-SA 4.0 (https://creativecommons.org/licenses/by-sa/4.0/).
- IN THIS TOPIC
You are here
Metabolic acidosis, what is metabolic acidosis.
The buildup of acid in the body due to kidney disease or kidney failure is called metabolic acidosis . When your body fluids contain too much acid, it means that your body is either not getting rid of enough acid, is making too much acid, or cannot balance the acid in your body.
What causes metabolic acidosis?
Healthy kidneys have many jobs. One of these jobs is to keep the right balance of acids in the body. The kidneys do this by removing acid from the body through urine. Metabolic acidosis is caused by a build-up of too many acids in the blood. This happens when your kidneys are unable to remove enough acid from your blood.
What are the signs and symptoms?
Not everyone will have signs or symptoms. However, you may experience:
- Long and deep breaths
- Fast heartbeat
- Headache and/or confusion
- Feeling very tired
- Vomiting and/or feeling sick to your stomach (nausea)
- Loss of appetite
If you experience any of these, it is important to let your healthcare provider know immediately.
What are the complications of metabolic acidosis if I have kidney disease or kidney failure?
- Increased bone loss (osteoporosis): Metabolic acidosis can lead to a loss of bone in your body. This can lead to a higher chance of fractures in important bones like your hips or backbone.
- Progression of kidney disease: Metabolic acidosis can make your kidney disease worse. Exactly how this happens is not clear. As acid builds up, kidney function lowers; and as kidney function lowers, acid builds up. This can lead to the progression of kidney disease.
- Muscle loss: Albumin is an important protein in your body that helps build and keep muscles healthy. Metabolic acidosis lowers the amount of albumin created in your body, and leads to muscle loss, or what is called “muscle wasting.”
- Endocrine disorders: Metabolic acidosis interferes with your body’s ability to maintain normal functions of your endocrine system (the collection of glands that produce hormones). This can cause your body to build a resistance to insulin (the hormone in your body that helps keep your blood sugar level from getting too high or too low). If left untreated for too long or not corrected in time, it can lead to diabetes.
How is it treated?
Bicarbonate : We all need bicarbonate (a form of carbon dioxide) in our blood. Low bicarbonate levels in the blood are a sign of metabolic acidosis. It is an alkali (also known as base), the opposite of acid, and can balance acid. It keeps our blood from becoming too acidic. Healthy kidneys help keep your bicarbonate levels in balance. Low bicarbonate levels (less than 22 mmol/l) can also cause your kidney disease to get worse. A small group of studies have shown that treatment with sodium bicarbonate or sodium citrate pills can help keep kidney disease from getting worse. However, you should not take sodium bicarbonate or sodium citrate pills unless your healthcare provider recommends it.
Diet : Increasing fruit and vegetable intake may decrease acid load in the body. This is because fruits and vegetables produce alkali, whereas foods such as meats, eggs, cheese, and cereal grains cause the body to make acid. Your kidney dietitian can show you how to safely increase the right type and amounts of fruits and vegetables in your diet based on your stage of kidney disease.
Save this content:
Share this content:
Is this content helpful?
Back to top:
Advertisement
The Evaluation and Treatment of Metabolic Acidosis
- Pediatric Nephrology (B Dixon and E Nehus, Section Editors)
- Published: 20 April 2020
- Volume 6 , pages 29–37, ( 2020 )
Cite this article
- Paul Thomlinson DO 1 ,
- Melinda Carpenter MS 2 , 3 &
- Cynthia D’Alessandri-Silva MD 1 , 2
459 Accesses
Explore all metrics
Purpose of review
Metabolic acidosis has a wide array of etiologies making the initial evaluation imperative in order to identify the proper treatment pathway. The clinical sequela of metabolic acidosis can often be vague and nonspecific. This underscores the importance of being able to comfortably recognize and evaluate acid-base disturbances.
Recent findings
The diagnostic modalities used to evaluate metabolic acidosis have existed for quite some time, though the validity and usefulness of particular metrics have shown superior to others. Similarly, as our understanding of disease processes progresses, the conditions that warrant and respond to the use of base therapy are rare.
Metabolic acidosis has potentially grave consequences to patient outcomes. As a result, timely diagnosis and recognition of treatment options is vital.
This is a preview of subscription content, log in via an institution to check access.
Access this article
Price excludes VAT (USA) Tax calculation will be finalised during checkout.
Instant access to the full article PDF.
Rent this article via DeepDyve
Institutional subscriptions
Similar content being viewed by others
Core Concepts and Treatment of Metabolic Acidosis
Acidosis: Diagnosis and Treatment
Pathophysiologic Approach to Metabolic Acidosis
References and recommended reading, papers of particular interest, published recently, have been highlighted as: • of importance •• of major importance.
Kraut JA, Madias NE. Metabolic acidosis: pathophysiology, diagnosis and management. Nat Rev Nephrol. 2010;6(5):274–85.
Article CAS Google Scholar
Hamm LL, Nakhoul N, Hering-Smith KS. Acid-base homeostasis. Clin J Am Soc Nephrol. 2015;10(12):2232–42.
Mustaqeem R, Arif A. Renal tubular acidosis: StatPearls Publishing; 2019.
Weiner ID, Verlander JW, Wingo CS. Renal acidification mechanisms. In: Mount D, Sayegh M, Singh A, editors. Core concepts in the disorders of fluid, electrolytes and acid-base balance. Boston: Springer; 2013.
Google Scholar
Mora Carpio AL, Mora JI. Ventilator management. [Updated 2019 Jun 2]. In: StatPearls. Treasure Island (FL): StatPearls Publishing; 2019.
•• Jung B, Martinez M, Claessens YE, Darmon M, Klouche K, Lautrette A, et al. Diagnosis and management of metabolic acidosis: guidelines from a French expert panel. Ann Intensive Care. 2019;9(1). This article discusses consensus guidelines in the approach, evaluation, and treatment of metabolic acidosis based on the most recent research to date.
Adeva-Andany MM, Carneiro-Freire N, Donapetry-García C, Rañal-Muíño E, López-Pereiro Y. The importance of the ionic product for water to understand the physiology of the acid-base balance in humans. Biomed Res Int. 2014;2014:695281. https://doi.org/10.1155/2014/695281 .
Article PubMed PubMed Central Google Scholar
Feldman M, Soni N, Dickson B. Influence of hypoalbuminemia or hyperalbuminemia on the serum anion gap. J Lab Clin Med. 2005;146:317–20.
Battle D, Ba Aqell SH, Marquez A. The urine anion gap in context. Clin J Am Soc Nephrol. 2018;13(2):195–7.
Article Google Scholar
Kraut JA, Madias NE. Consequences and therapy of the metabolic acidosis of chronic kidney disease. Pediatr Nephrol. 2011;26(1):19–28. https://doi.org/10.1007/s00467-010-1564-4 .
Article PubMed Google Scholar
Adeva-Andany MM, Fernández-Fernández C, Mouriño-Bayolo D, Castro-Quintela E, Domínguez-Montero A. Sodium bicarbonate therapy in patients with metabolic acidosis. Sci World J. 2014;2014(1).
Hindman BJ. Sodium bicarbonate in the treatment of subtypes of acute lactic acidosis: physiologic considerations. Anesthesiology. 1990;72(6):1064–76.
• Ghauri SK, Javaeed A, Mustafa KJ, Podlasek A, Khan AS. Bicarbonate therapy for critically ill patients with metabolic acidosis: a systematic review. Cureus. 2019;11(3):e4297. Published 2019 Mar 22. https://doi.org/10.7759/cureus.4297 . Systematic review of 128 relevant articles investigating the use of intravenous sodium bicarbonate in metabolic acidosis most often due to lactic acidosis. The systematic review provides extensive reasoning and support for the recommendation not to use sodium bicarbonate in cases of poor perfusion/septic shock with a consequent lactic acidosis.
Sabatina S, Kurtzman NA. Bicarbonate therapy in severe metabolic acidosis. J Am Soc Nephrol. 2009;20:692–5.
Goldsmith DJA, Forni LG, Hilton PJ. Bicarbonate therapy and intracellular acidosis. Clin Sci. 1997;93(6):593–8.
Ritter JM, Doktor HS, Benjamin N. Paradoxial effect of bicarbonate on cytoplasmic pH. Lancet. 1990;335(8700):1243–6.
Hoste EA, Colpaert K, Vanholder RC, Lameire NH, De Waele JJ, Blot SI, et al. Sodium bicarbonate versus THAM in ICU patients with mild metabolic acidosis. J Nephrol. 2005;18(3):303–7.
CAS PubMed Google Scholar
Singer RB, Deering RC, Clark JK. The acute effects in man of a rapid intravenous infusion of hypertonic sodium bicarbonate solution. II. Changes in respiration and output of carbon dioxide. J Clin Invest. 1956;35(2):245–53.
Nahas GG, Sutin KM, Fermon C, Streat S, Wiklund L, Wahlander S, et al. Guidelines for the treatment of acidaemia with THAM. Drugs. 1998;55:191–224.
Lu C, Leibner E, Wright B. The use of tris-hydroxymethyl aminomethane in the emergency department. Clin Exp Emerg Med. 2016;3(4):264–5. Published 2016 Dec 30. https://doi.org/10.15441/ceem.16.165 .
Starke A, Corsenca A, Kohler T, Knubben J, Kraenzlin M, Uebelhart D, et al. Correction of metabolic acidosis with potassium citrate in renal transplant patients and its effect on bone quality. Clin J Am Soc Nephrol. 2012;7(9):1461–72. https://doi.org/10.2215/CJN.01100112 .
Article CAS PubMed PubMed Central Google Scholar
Mirrakhimov AE, Ayach T, Barbaryan A, Talari G, Chadha R, Gray A. The role of sodium bicarbonate in the management of some toxic ingestions. Int J Nephrol. 2017;2017:7831358.
Download references
Author information
Authors and affiliations.
Department of Pediatrics, University of Connecticut Health Center, Farmington, CT, USA
Paul Thomlinson DO & Cynthia D’Alessandri-Silva MD
Division of Nephrology, Connecticut Children’s Medical Center, 282 Washington Street, Hartford, CT, 06106, USA
Melinda Carpenter MS & Cynthia D’Alessandri-Silva MD
Department of Research, Connecticut Children’s Medical Center, Hartford, CT, USA
Melinda Carpenter MS
You can also search for this author in PubMed Google Scholar
Corresponding author
Correspondence to Cynthia D’Alessandri-Silva MD .
Ethics declarations
Conflict of interest.
Dr. Melinda Carpenter declares no conflict of interest.
Dr. Cynthia D’Alessandri-Silva declares funds received from Advicenne Medical.
Dr. Thomlinson has nothing to disclose at this time
Human and Animal Rights and Informed Consent
This article does not contain any studies with human or animal subjects performed by any of the authors.
Additional information
Publisher’s note.
Springer Nature remains neutral with regard to jurisdictional claims in published maps and institutional affiliations.
This article is part of the Topical Collection on Pediatric Nephrology
Rights and permissions
Reprints and permissions
About this article
Thomlinson, P., Carpenter, M. & D’Alessandri-Silva, C. The Evaluation and Treatment of Metabolic Acidosis. Curr Treat Options Peds 6 , 29–37 (2020). https://doi.org/10.1007/s40746-020-00192-6
Download citation
Published : 20 April 2020
Issue Date : June 2020
DOI : https://doi.org/10.1007/s40746-020-00192-6
Share this article
Anyone you share the following link with will be able to read this content:
Sorry, a shareable link is not currently available for this article.
Provided by the Springer Nature SharedIt content-sharing initiative
- Metabolic acidosis
- Find a journal
- Publish with us
- Track your research
Your cart is empty
Have an account?
Log in to check out faster.
Estimated total
FREE US SHIPPING ON ALL FLASHCARDS
ABG Interpretation, part 6: Metabolic Acidosis
In this article, we'll teach you all about metabolic acidosis, including: the difference between uncompensated, partially-compensated, and fully compensated, and the lab values you can expect to see with each of those; what causes metabolic acidosis; and its symptoms and treatment.
The Arterial Blood Gas video article series follows along with our Arterial Blood Gas Flashcards , which are intended to help RN and PN nursing students study for nursing school exams, including the ATI, HESI, and NCLEX. You can also check out our ABG cheatsheet and practice questions .
Lab Values & ABG Interpretation - Nursing Flashcards
4.914893617 / 5.0
(94) 94 total reviews
Metabolic acidosis
You can identify metabolic acidosis on an ABG using two steps:
- Check the pH. A pH under 7.35 is acidic, and indicative of acidosis.*
- Determine which system, respiratory or metabolic, is to blame for the acidosis. The value for bicarbonate (HCO₃) represents the metabolic system. So if HCO₃ is under 22 mEq/L, which means it's also acidic, this means the metabolic system is to blame for the acidosis.
If pH is under 7.35* and HCO₃ is under 22 mEq/L, it's metabolic acidosis.
*Is the pH technically normal but on the acidic side? It may still be metabolic acidosis—fully compensated!
Metabolic acidosis with respiratory compensation
Once you have identified metabolic acidosis, you need to determine if the respiratory system is compensating or not. The respiratory system can be represented by partial pressure of carbon dioxide (PaCO₂), so we can look to PaCO₂ to determine how much, if at all, the respiratory system is compensating.
If PaCO₂ is in the normal range (35 - 45 mmHg), the respiratory system is not trying to compensate for the acidosis.
If the PaCO₂ level is basic (under 35 mmHg), it means the respiratory system is trying to compensate for metabolic acidosis. But how do you know the difference between partially and fully compensated metabolic acidosis?
When pH is "normal"
If PaCO₂ is basic (under 35 mmHg), some amount of respiratory compensation is happening for the metabolic acidosis. The respiratory system's goal with compensation is to get the pH to the normal range.
If the pH is not in the normal range, the respiratory system has not completed its job all the way, so the metabolic acidosis is only partially compensated .
If the pH has been knocked back into the normal range, the respiratory system has succeeded and the metabolic acidosis is fully compensated .
In step 1, we evaluated the pH to determine if acidosis was occurring, we stated that a pH under 7.35 indicates acidosis. That is a strong rule of thumb, except in the cases of fully compensated acidosis, where the pH will be normal but on the acidic side.
Uncompensated metabolic acidosis
Uncompensated metabolic acidosis occurs when metabolic acidosis is present, with pH acidic under 7.35 and HCO₃ acidic (under 22 mEq/L); but the respiratory system does not act to correct it, marked by PaCO₂ in the normal range (35 - 45 mmHg).
Partially compensated metabolic acidosis
Partially compensated metabolic acidosis occurs when metabolic acidosis is present, with pH acidic (under 7.35) and HCO₃ acidic (under 22 mEq/L); and the respiratory system acts to correct it, marked by an PaCO₂ level that's basic (under 35 mmHg).
The respiratory system's goal with compensation is to get the pH to the normal range. In the case of partially compensated metabolic acidosis, the respiratory system has only partially succeeded in correcting the acidosis, because the pH is still acidic and outside the normal range.
Fully compensated metabolic acidosis
Fully compensated metabolic acidosis occurs when metabolic acidosis is present, with pH normal but closer to acidic (7.35 - 7.39) and HCO₃ acidic (under 22 mEq/L); and the respiratory system acts to correct it, marked by a PaCO₂ level that's basic (under 35 mmHg).
Again, the respiratory system's goal with compensation is to get the pH to the normal range. In the case of fully compensated metabolic acidosis, the respiratory system has succeeded in its goal of correcting the acidosis, because the pH was pushed back into the normal range.
If you're following closely, you might be thinking... pH in the normal range? I thought we can tell if it's acidosis or alkalosis in the first place by the pH being outside the normal range?
That's the trick with fully compensated metabolic acidosis. The pH may be technically within the normal range. But the way to determine this is still metabolic acidosis is that the pH is on the acidic side (7.35 - 7.39) and the other two blood gases, HCO₃ and PaCO₂ are outside the normal range.
Common causes of metabolic acidosis include diabetic ketoacidosis (DKA), kidney failure, starvation, or anything that causes hypermetabolism.
Another cause of metabolic acidosis is diarrhea, which can lead to a loss of bicarbonate (HCO₃ under 22 mEq/L).
Other causes of metabolic acidosis include dehydration, pancreatitis, or liver failure.
Diabetic Ketoacidosis (DKA)
The key cause of metabolic acidosis is diabetic ketoacidosis (DKA) . This is a life-threatening complication for diabetic patients (usually with Type 1 diabetes).
Insulin carries glucose into the body's cells where it can be used as energy. If a diabetic patient is not producing enough insulin or any insulin, the glucose can't get from their bloodstream into their cells to be used for energy. The cells want energy, so they start breaking down protein and fat to get it. The breakdown of protein and fat causes an accumulation of acid in the body, including ketones, which causes metabolic acidosis.
For the key facts you need to remember on DKA, check out our Medical-Surgical Nursing lesson on Diabetes Complications . This is one of the many important topics covered in our Medical-Surgical Nursing Flashcards .
Kidney failure and starvation can lead to metabolic acidosis for the same reason as DKA. If cells can't get glucose in, they begin to break down muscle and fat, which causes acid buildup.
Hypermetabolism
Hypermetabolism is elevated metabolic activity wherein the body's basal metabolic rate is increased. If the body is burning through its glucose quickly, it may turn to protein and fat for energy, causing acid buildup. Hypermetabolism can be caused by a fever or infection, seizures, or heavy exercising.
Medications
Some medications that interfere with the reabsorption of HCO₃ can cause metabolic acidosis as a side effect. One such medication is the anti seizure medication topiramate . For your exams, however, the focus will be on the more common causes outlined above.
Signs and symptoms
Symptoms of metabolic acidosis include hypotension, tachycardia , weak pulses, GI upset (nausea, vomiting), and dysrhythmias.
Dysrhythmias and metabolic acidosis
Dysrhythmias (abnormal heart rhythms) can be a symptom of metabolic acidosis. Dysrhythmias can be caused by electrolyte imbalances. Electrolytes are minerals that have electric charge, and the heart relies on them to conduct its electrical impulses to keep itself running. Potassium is an important electrolyte for the heart.
During metabolic acidosis, the potassium levels in the body usually increase. So, metabolic acidosis leads to hyperkalemia (too much potassium ), and hyperkalemia can cause life-threatening dysrhythmias.
For the key facts you need to remember on the different types of dysrhythmias, check out our EKG Interpretation Series . This video series follows along with our EKG Flashcards for Nursing Students .
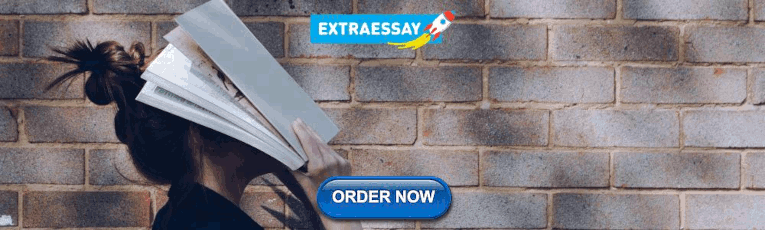
Kussmaul's respirations
Other signs and symptoms of metabolic acidosis can include Kussmaul's respirations. This is a key symptom of DKA in a diabetic patient. Kussmaul's respirations are distinctively deep, rapid breaths. This is respiratory compensation in action—the respiratory system working overtime to try to balance out metabolic acidosis by removing carbon dioxide (lowering PaCO₂).
Other symptoms of DKA are a fruity breath odor (that's the glucose!) and warm, flushed skin.
One treatment option for metabolic acidosis is to provide the patient with sodium bicarbonate , an alkalinizing agent. This is in an effort to lower the acid levels by creating acid-base balance.
A patient with metabolic acidosis may need IV fluids and insulin , if they have DKA. Giving the patient insulin helps their body get glucose into the cells, so the cells stop breaking down protein and fat for energy, which lowers the acid in the bloodstream.
Sodium bicarbonate and insulin are some of the important medications covered in our Pharmacology Flashcards for Nursing Students .
If a patient has renal failure (kidney failure), then hemodialysis is another option.
Comparisons
Metabolic vs. respiratory acidosis.
The difference between metabolic and respiratory acidosis stems from which body system causes the acidosis. Metabolic acidosis is when the HCO₃ of the metabolic system is acidic and causes the body's pH to become acidic. Respiratory acidosis happens when the PaCO₂ of the respiratory system is acidic and causes the body's pH to become acidic.
Learn more about respiratory acidosis .
Metabolic acidosis vs. alkalosis
The difference between metabolic acidosis and alkalosis is how acidic or alkaline (basic) the blood is. Metabolic acidosis happens when the HCO₃ of the metabolic system is acidic and causes the body's pH to become acidic. Metabolic alkalosis happens when the HCO₃ levels are basic and cause the body's pH to become basic.
Learn more about metabolic alkalosis .
Full Transcript: ABG Interpretation, part 6: Metabolic Acidosis
In this video, we will talk about metabolic acidosis. So we'll talk about what lab values you will see with uncompensated metabolic acidosis, partially compensated metabolic acidosis, and fully compensated metabolic acidosis. We will also cover the causes of metabolic acidosis, what symptoms you may see in your patient, and treatment options for metabolic acidosis.
Alright. So when we look at the pH, if it is under 7.35, then we know we have acidosis present, and we just have to figure out who's to blame.
When we look at PaCO2 here, if it's in the normal range between 35 and 45, then we can't blame that acidosis on the respiratory system. When we go over here to HCO3, which is bicarb which represents the metabolic system, we can see it's low, it's under 22, which means that we have metabolic acidosis and that it is uncompensated because this respiratory system's not doing anything to try to save the day. It's not trying to fix the situation, it's just chilling out in its normal range, so we have uncompensated metabolic acidosis.
Then if we go to the second row here, again, if the pH is under 7.35, we have acidosis.
Who's to blame? If we look at PaCO2, it is under 35 which means it's basic. So we definitely know that the respiratory system is not to blame for the acidosis.
However, with HCO3, we see that it is low under 22. So the metabolic system is to blame for the acidosis. And the respiratory system is trying to fix the situation, right? It's becoming more basic to help counteract this acidosis.
So we have partially compensated metabolic acidosis. The metabolic system is to blame for the acidosis. Respiratory system's trying to save the day but hasn't done so completely because that pH is still out of range.
Then if we go down to this last row, if we have a pH that is within the normal range, so it's between 7.35 and 7.45, but it's a little on the low side like if it's 7.35 or 7.36, then you can guess there may be some compensation that is occurring.
And sure enough, if we look here at PaCO2, we'll see that it's basic. It is under 35.
And if we look at HCO3, it is acidic. It is under 22.
So we can deduce that we had metabolic acidosis that was fully compensated by the respiratory system because our pH is in normal range. So again the metabolic system caused the acidosis - they're to blame - and the respiratory system came in and fixed the situation, got that pH in the normal range.
Alright. Let's talk about some of the causes of metabolic acidosis. One big cause is something called diabetic ketoacidosis, or DKA. This is a life-threatening complication for, typically, Type 1 diabetic patients that we see fairly regularly in the hospital. So with DKA, these Type 1 diabetic patients, they don't produce enough insulin or any insulin, and so the glucose can't get from the patient's bloodstream into their cells. They can't get in there because it needs insulin to get in there. So these cells feel like they're starving to death, right, so they start breaking down protein and fat to try to get energy. And with that breakdown of protein and fat, we get an accumulation of acid in the body, including ketones, and that's what causes the metabolic acidosis.
Other causes of metabolic acidosis include kidney failure, so that's another really common cause, as well as starvation, for kind of the same reasons as DKA, right? If your cells are starving, they can't get glucose in. They start breaking down that muscle and fat, the protein and fat, which is how we have that accumulation of acid.
Other causes include diarrhea. So one way to remember that diarrhea causes metabolic acidosis is that you lose bicarb out the butt. Another tip that someone gave me is that with diarrhea, you have diarrhea out the acidosis. So if either of those little tricks help you, that's great.
Other causes include dehydration, pancreatitis, liver failure, and then anything that causes hypermetabolism. So this could be something such as a fever, seizures, or heavy exercising.
And then in terms of symptoms, symptoms of metabolic acidosis include hypotension, tachycardia, weak pulses, GI upset such as nausea and vomiting, dysrhythmias because we often have hyperkalemia or too high of our potassium levels when it comes to metabolic acidosis. It happens almost all the time. So when you think of metabolic acidosis, I want you to think of hyperkalemia. And when you think of hyperkalemia, I want you to know that there is a high risk of life-threatening dysrhythmias when a patient's potassium levels are out of whack.
Other signs and symptoms can include Kussmaul's respirations. So you would see this with a diabetic patient who has DKA. They have this deep rapid breathing due to respiratory compensation for that metabolic acidosis. So just like we were talking before, the metabolic system is to blame for the acidosis, and the respiratory system often tries to compensate. And it will do that by increasing the depth and the rate of breathing, so you have this really deep, rapid breathing that you see with Kussmaul respirations.
Other things include a fruity odor or breath, so that's definitely a sign of DKA and of metabolic acidosis, and then warm, flushed skin.
In terms of treatment, we can provide the patient with sodium bicarbonate. So again, we're trying to eliminate the acid, trying to create acid-base balance. So we can give them bicarbonate.
We can also give them IV fluids as well as insulin for DKA. So by giving them insulin, we can get that glucose into the cells so that the cells will stop trying to break down that fat and that protein.
And then, if a patient has renal failure, like kidney failure, then hemodialysis is another option.
Okay. So that is it with metabolic acidosis. I spent a little more time on the causes, signs and symptoms, and treatment, just because it's really important for you to know as a nursing student and as a nurse. In my next video, we'll go over metabolic alkalosis, all of that same information. And we're on the home stretch, so stick with me. We're almost there.
Leave a comment
Please note, comments need to be approved before they are published.
Videos by Subject
- ABG Interpretation
- Clinical Nursing Skills
- Dosage Calculation
- EKG Interpretation
- Fundamentals
- Health Assessment
- Medical-Surgical
- Nutrition Essentials
- Pediatric Nursing
- Pharmacology
- Pharmacology Basics
- Psychiatric Mental Health
Tips & More
- Ask a Nurse
- Nursing Tips
- Resources for Nursing Students
- Why Flashcards Work
Exam Information
Subscribe to our emails.
- Choosing a selection results in a full page refresh.
- Opens in a new window.
Please select your country / currency.
Free economy shipping on The Survival Kit OR The Comprehensive Collection !
Select "USD" to purchase digital products including Level Up RN Membership.
- - Google Chrome
Intended for healthcare professionals
- Access provided by Google Indexer
- My email alerts
- BMA member login
- Username * Password * Forgot your log in details? Need to activate BMA Member Log In Log in via OpenAthens Log in via your institution

Search form
- Advanced search
- Search responses
- Search blogs
- Unexplained metabolic...
Unexplained metabolic acidosis
- Related content
- Peer review
- James McCaffrey , histopathology registrar ,
- Kieren Allinson , consultant neuropathologist
- Cambridge University Hospitals NHS Foundation Trust, Cambridge, UK
- Correspondence to: J McCaffrey jm2199{at}cam.ac.uk
A man in his 20s was found to be unresponsive after experiencing headache and vomiting for two days. His medical history included depression, paracetamol (acetaminophen) overdose on several occasions, and an episode of unexplained encephalopathy five months earlier. He experienced two generalised tonic-clonic seizures during initial assessment in the emergency department. Clinical examination revealed a Glasgow coma scale score of 3/15, with global hypotonia, areflexia, and size 6 pupils that were unresponsive to light.
A systematic ABCDE approach was taken, with a focus on securing the airway because of the patient’s decreased level of consciousness. 1
Arterial blood gas test results showed a metabolic acidosis with raised levels of lactate. Table 1 summarises the patient’s laboratory test results on presentation to the emergency department.
Laboratory test results on presentation to emergency department
- View inline
The patient was intubated and transferred to the intensive care unit.
1 What laboratory investigations are required for patients with metabolic acidosis?
2 What do the laboratory test results in table 1 show?
3 What are the differential diagnoses in this case?
When patients present with metabolic acidosis, 1 2 3 perform investigations for the following urgently:
Lactate: a high lactate level in critically unwell patients is a marker of poor prognosis 4
Serum electrolytes and creatinine: to assess kidney function and to calculate the anion gap
Serum osmolality: to calculate the osmolal gap
Serum ethanol: to exclude alcoholic ketoacidosis
Serum glucose: to investigate the possibility of diabetic ketoacidosis
Serum calcium: to exclude hypocalcaemia, which is an uncommon finding in patients with ethylene glycol poisoning. 2 3 5
Evaluation of the anion gap (calculated by subtracting serum concentrations of bicarbonate and chloride anions from concentrations of sodium and potassium cations) helps to delineate the possible causes of metabolic acidosis. Metabolic acidosis with a normal anion gap (eg, with diarrhoea) results from loss of bicarbonate with retention of chloride. 2 3
Evaluation of the osmolal gap (calculated by subtracting the calculated serum osmolality from the measured serum osmolality) helps to identify the presence of exogenous osmotically active substances such as ethylene glycol and methanol. 2 3
Consider cerebrospinal fluid analysis if infectious meningitis is suspected and urine toxicology screen if there is reduced consciousness of unknown aetiology.
A raised serum anion gap, increased serum osmolal gap, severe metabolic acidosis, reduced bicarbonate level, and high lactate level. 6
Raised anion gaps most commonly result from increases in “unmeasured anions” (ie, anions except for sodium and potassium), which reduce bicarbonate concentrations.
Increased osmolal gaps occur in the presence of abnormal, unmeasured osmotically active molecules, which can be exogenous (eg, methanol, ethylene glycol) or endogenous (eg, hypertriglyceridaemia). In ethylene glycol poisoning this gap might decrease with time as the toxin becomes metabolised. 2 The presence of ethylene glycol metabolites can also affect the measurement of lactic acid. 7
The normal ethanol concentration ruled out alcoholic ketoacidosis.
The normal salicylate concentration excluded aspirin ingestion as a cause of the high anion gap metabolic acidosis. The normal venous blood glucose concentration suggested that diabetic ketoacidosis was unlikely.
Infectious meningitis can cause headache with lactic acidosis and raised white cell count.
Recurrent encephalopathy with lactic acidosis could be caused by a mitochondrial disorder—differentiating tests are serum creatinine kinase, cerebrospinal fluid lactate measurements, and skeletal muscle biopsy.
The patient’s history of depression and paracetamol overdose increased the possibility of an ingested toxic substance.
In patients with altered mental state and a high anion gap metabolic acidosis, consider the mnemonic CAT MUDPILES (Cyanide/Carbon monoxide, Alcoholic ketoacidosis Toluene, Methanol, Uraemia, Diabetic ketoacidosis, Paraldehyde, Isoniazid/Iron, Lactic acidosis, Ethylene glycol, Salicylates).
The following investigations can also help to elicit the cause of a high anion gap metabolic acidosis: blood glucose, ketones, lactate, methanol (if available), salicylate concentrations, and blood ethylene glycol concentration (if available), and urinalysis to assess for the presence of calcium oxalate crystals (which are present in about half of patients with ethylene glycol poisoning). 1 2
Patient outcome
Treatment for presumed infectious meningitis was started. Serum creatinine kinase and cerebrospinal fluid lactate measurements were obtained ( table 2 ) and the plan was to perform a skeletal muscle biopsy.
Laboratory test results on admission to intensive care unit (day 0) and day 2 after admission. Relevant venous blood test results on presentation to the emergency department (day 0) are included for comparison
On day 2 of admission, magnetic resonance imaging showed evidence of tonsillar herniation and cerebral global hypoxic changes, and the patient developed acute kidney injury ( table 2 ). The patient died on day 3.
Glycolic acid and oxalic acid were found on post mortem urinalysis, and calcium oxalate crystals were identified on histology of the leptomeninges. The coroner considered ethylene glycol overdose to be the most likely cause of death.
The high cerebrospinal fluid protein level might have been related to breakdown of the blood-brain barrier after a period of seizure activity before death, with cerebral hypoxic changes and tonsillar herniation. 8 9 Noticeably high protein cerebrospinal fluid levels have been documented in patients with ethylene glycol poisoning. 10
Learning points
For critically unwell patients with no clear cause, seek early intensivist advice.
Check venous blood for electrolytes, ethanol, glucose, calcium concentration, and osmolality 1 when patients present with metabolic acidosis
Consider the mnemonic CAT MUDPILES for acutely unwell patients with a high anion gap metabolic acidosis
Undertake urinalysis for calcium oxalate crystals if ethylene glycol poisoning is suspected.
Competing interests: The BMJ has judged that there are no disqualifying financial ties to commercial companies. The authors declare the following other interests: none.
Further details of The BMJ policy on financial interests are here: https://www.bmj.com/about-bmj/resources-authors/forms-policies-and-checklists/declaration-competing-interests .
Patient consent not required: Patient deceased and material anonymised.
Provenance and peer review: Not commissioned; externally peer reviewed.
- ↵ Methanol and ethylene glycol poisoning: Management - UpToDate. https://www.uptodate.com/contents/methanol-and-ethylene-glycol-poisoning-management .
- Scalley RD ,
- Ferguson DR ,
- Piccaro JC ,
- Seheult J ,
- Fitzpatrick G ,
- Hodgman M ,
- Marraffa JM ,
- Bejjanki H ,
- Saathoff N ,
- Hannich M ,
- von Podewils F
- Venkatesh B ,
- Ziegenfuss M
- Baldwin F ,
Thank you for visiting nature.com. You are using a browser version with limited support for CSS. To obtain the best experience, we recommend you use a more up to date browser (or turn off compatibility mode in Internet Explorer). In the meantime, to ensure continued support, we are displaying the site without styles and JavaScript.
- View all journals
- My Account Login
- Explore content
- About the journal
- Publish with us
- Sign up for alerts
- Open access
- Published: 06 April 2024
Missed postoperative metabolic acidosis associated with sodium-glucose transporter 2 inhibitors in cardiac surgery patients: a retrospective analysis
- Hyeon A Kim ORCID: orcid.org/0000-0002-0648-948X 1 ,
- Joo Yeon Kim ORCID: orcid.org/0000-0002-5661-1068 1 ,
- Young Hwan Kim ORCID: orcid.org/0000-0003-4633-3102 2 ,
- Young Tak Lee ORCID: orcid.org/0000-0001-7421-2178 2 &
- Pyo Won Park ORCID: orcid.org/0000-0002-0892-4107 2
Scientific Reports volume 14 , Article number: 8087 ( 2024 ) Cite this article
Metrics details
- Risk factors
The increasing use of sodium glucose transporter 2 inhibitors (SGLT2i) for treating cardiovascular (CV) diseases and type 2 diabetes (T2D) is accompanied by a rise in euglycemic diabetic ketoacidosis occurrences in cardiac surgery patients. Patients undergoing cardiac surgery, due to their pre-existing CV disease which often requires SGLT2i prescriptions, face an increased risk of postoperative metabolic acidosis (MA) or ketoacidosis (KA) associated with SGLT2i, compounded by fasting and surgical stress. The primary aim of this study is to quantify the incidence of SGLT2i-related postoperative MA or KA and to identify related risk factors. We analyzed data retrospectively of 823 cardiac surgery patients, including 46 treated with SGLT2i from November 2019 to October 2022. Among 46 final cohorts treated preoperatively with SGLT2i, 29 (63%) developed postoperative metabolic complications. Of these 46 patients, stratified into two categories based on postoperative laboratory findings, risk factor analysis were conducted and compared. Analysis indicated a prescription duration over one week significantly elevated the risk of complications (Unadjusted OR, 11.7; p = 0.032*; Adjusted OR, 31.58; p = 0.014*). A subgroup analysis showed that a cardiopulmonary bypass duration of 60 min or less significantly raises the risk of SGLT2i-related postoperative MA in patients with a sufficient prescription duration. We omitted the term "diabetes" in describing complications related to SGLT2i, as these issues are not exclusive to T2D patients. Awareness of SGLT2i-related postoperative MA or KA can help clinicians distinguish between non-life-threatening conditions and severe causes, thereby preventing unnecessary tests and ensuring best practice.
Introduction
The U.S. Food and Drug Administration (FDA) granted approval for dapagliflozin, a sodium-glucose transporter 2 inhibitor (SGLT2i), in 2011 for the treatment of type 2 diabetes (T2D). Subsequent research has extensively shown the efficacy of these inhibitors in treating a broader spectrum of cardiovascular (CV) diseases. Two landmark trials—DAPA-HF (Dapagliflozin in Patients with Heart Failure and Reduced Ejection Fraction) 1 and EMPEROR-Reduced (Cardiovascular and Renal Outcomes with Empagliflozin in Heart Failure) 2 —have explored the effectiveness of SGLT2i in treating heart failure with reduced ejection fraction (HFrEF), irrespective of the presence of T2D. Further studies like EMPEROR-preserved (Empagliflozin in Heart Failure with a Preserved Ejection Fraction) 3 and DELIVER (Dapagliflozin in Heart Failure with Mildly Reduced or Preserved Ejection Fraction) 4 have broadened their applicability to HF patients with preserved or mildly reduced EF (HFpEF or HFmrEF) irrespective of T2D status. In alignment with these findings, the 2021 European Society of Cardiology guidelines for the treatment of acute and chronic HF recommended dapagliflozin or empagliflozin for patients with HFrEF to reduce the risk of hospitalization for HF (HHF) and death (Class IA) 5 . Furthermore, the 2022 ACC/AHA/HFSA Guideline for the Management of HF 6 advocated for the use of SGLT2i in patients with symptomatic chronic HFrEF to reduce HHF and CV mortality, irrespective of the presence of T2D. More recently, empagliflozin now has FDA approval for reducing HHF or CV death among patients with HFpEF, defined as left ventricular EF being greater than 40%, with or without diabetes 7 . With the comprehensive management of cardio-renal-metabolism being emphasized, the utilization of SGLT2i is expected to increase over the years, not only in patients with T2D but also in those with CV disease or diabetic kidney disease.
Regarding diabetic ketoacidosis (DKA) related to SGLT2i, these medications have been associated with an elevated risk of DKA 8 , 9 . In 2015, the FDA issued a warning about the potential for these drugs to cause ketoacidosis, which generated considerable attention 10 . Numerous studies have documented instances of 'euglycemic' DKA (EDKA), generally characterized by normoglycemia with a plasma glucose level of less than 200 mg/dL, in T2D patients treated SGLT2i 11 , 12 , 13 , 14 .
In the perioperative setting, diagnosing SGLT2i-related EDKA becomes more complicated due to the specific postoperative status of patients. Several studies have already highlighted the issue of unrecognized diagnosis of SGLT2i-related EDKA in this context. Thiruvenkatarajan. et al. reported a 89% prevalence of postoperative SGLT2i-related EDKA in systematic review 15 , while Blau. et al. found a 71% incidence in their research using FDA data 16 . Among cardiac surgery patients, Murugesan, K.B. observed a 70.8% incidence of SGLT2i-related ketoacidosis (KA) 17 . Given these findings, it is imperative to differentiate SGLT2i-induced KA in cardiac surgery patients for the following reasons:
Due to shared patient population with HF, cardiac surgery patients show a higher incidence of SGLT2i usage than other surgical patients.
While the surgical stress is a known risk factor for SGLT2i-induced EDKA, cardiac surgery is unique in the implementation of cardiopulmonary bypass (CPB), setting it apart from other surgical procedures.
The laboratory presentations of SGLT2i-induced KA may mimic those of other severe postoperative complications in cardiac surgery patients, leading to potential diagnostic delays and additional expenditures.
Moreover, the clinical application of SGLT2i expands in CV patients, six cases of postoperative SGLT2i-related KA following cardiac surgery were identified in our center. Given the subtle presentation of SGLT2i-related KA, we hypothesized that it might be underdiagnosed, prompting a retrospective analysis of cardiac surgery patients who were preoperatively administered SGLT2i. Due to the retrospective nature of the study, confirmation of ketone body presence could not be conducted for all patients except the six identified; hence, their condition was termed as SGLT2i-related metabolic acidosis (MA).
The primary aim of this study is to quantify the incidence of postoperative SGLT2i related KA or MA and to identify related risk factors. Such insights could assist clinicians in differentiating SGLT2i-associated postoperative KA or MA, which is typically non-life-threatening 18 , from cardiac or other severe causes of MA, thus preventing unnecessary tests and prolonged intensive care unit (ICU) stays. Furthermore, this study assessed the impact of CPB, which induces a reduction in plasma protein concentration during bypass, on SGLT2i-related KA or MA 19 .
Materials and methods
This study is a retrospective analysis of 823 patients who underwent cardiac surgery at Incheon Sejong Hospital from November 2019 to October 2022. Of the initial cohort, 55 were preoperatively prescribed SGLT2i. Exclusion criteria were set for conditions likely to induce postoperative acidosis 20 , including low cardiac output, postoperative bleeding, respiratory acidosis, elevated lactate levels (> 3 mmol/L), low-dose epinephrine (Type B lactic acidosis), intra-abdominal catastrophes, sepsis, renal failure, and acute hepatic dysfunction. An additional patient prescribed an SGLT2i other than Empagliflozin or Dapagliflozin was excluded for study consistency. Subsequently, the refined cohort consisted of 46 patients, stratified into two categories based on postoperative laboratory findings: the MA group (pH < 7.35 and HCO3- ≤ 22 mEq/L, n = 27) and the non-MA group (pH ≥ 7.35 or HCO3- > 22 mEq/L, n = 19). [Fig. 1 ] In both groups, we collected data on preoperative baseline characteristics, intraoperative factors, and immediate postoperative factors to identify risk factors associated with SGLT2i-related KA or MA.
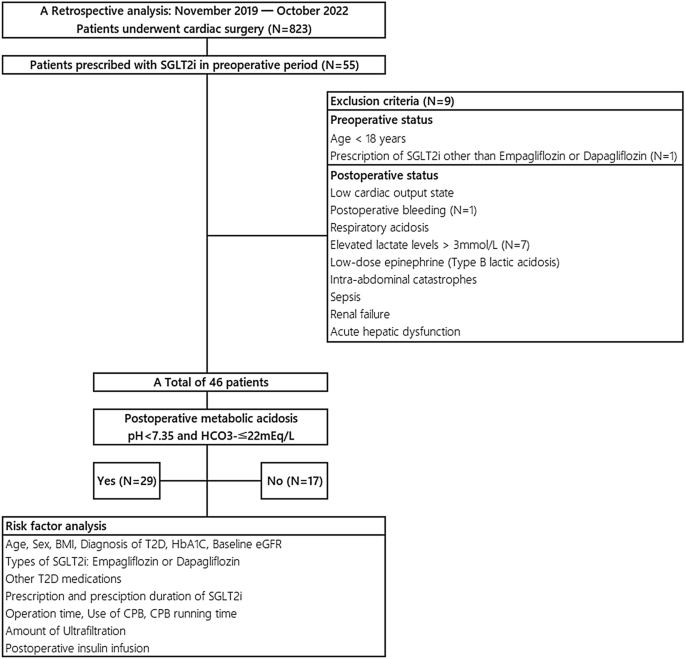
Patient selection, exclusion criteria and risk factor analysis. From November 2019 to October 2022, we conducted a retrospective analysis on 823 cardiac surgery patients at our center, 46 of whom were preoperatively treated with SGLT2i, specifically dapagliflozin or empagliflozin. Based on postoperative arterial blood gas analysis, patients were categorized into two groups: those with postoperative metabolic acidosis and those without. SGLT2i sodium-glucose transporter 2 inhibitors, BMI body mass index, T2D type 2 diabetes, HbA1c Hemoglobin A1C, eGFR estimated glomerular filtration rate, CPB cardiopulmonary bypass.
To clarify, the postoperative period generally refers to the time starting immediately after surgery, typically beginning with the patient's admission to the ICU. At our institution, preoperative glycemic control in T2D patients is managed with a glucose-insulin mix or glucose-insulin-potassium (GIK) solution, following endocrinologist consultation. Postoperatively, we follow ICU protocol to maintain blood glucose between 110 and 150 mg/dL using insulin infusion 20 . Both preoperative and postoperative insulin infusions are administered as mentioned earlier.
Statistical analysis
The IBM SPSS software, version 21.0, was utilized for data analysis. Continuous variables were expressed as mean ± standard deviation (SD) or as median (25th and 75th percentiles), while categorical variables were presented as frequency counts and percentages (n%). Independent t-tests and χ 2 tests were employed, as appropriate, to compare variables such as types of SGLT2i, arterial blood gas analyses (ABGA), duration of mechanical ventilator (MV) support, and length of ICU stay between the postoperative MA group and the non-MA group. The Mann–Whitney U test was used to compare the number of inotropes administered between the two groups. Univariate logistic regression analysis was conducted to identify potential associated risk factors in preoperative, intraoperative and postoperative period. The covariables for the multivariable logistic regression model were selected based on clinical judgement and a comprehensive review of existing literature. The variables evaluated in this model include Body Mass Index (BMI), the status of preoperative insulin usage, duration of SGLT2i prescription and cessation, operating time, CPB duration, and postoperative insulin utilization. Also, CPB running time was divided into before and after 60 min to indicate a short CPB running time. While there is no basis for specifically choosing 60 min, but generally, one shot of cardioplegia lasts between 60 to 90 min, depending on the type of cardioplegia used. We set 60 min as the criterion for short CPB time, which is also related to the reduction of plasma protein concentration and the duration or amount of ultrafiltration. The impact CPB duration on postoperative MA or KA associated with SGLT2i was examined in a subgroup analysis of patients with sufficient prescription duration. The odds ratio (OR) or hazard ratio (HR) are presented with a 95% confidence interval(CI) for inferential statistics, and a two-sided P-value of less than 0.05 was statistically significant.
Ethics approval and consent to participate
Given the retrospective design of this study and the fact that there was no harm to participants, the Institutional Review Board of Incheon Sejong Hospital approved a waiver for the requirement of informed consent. (IRB approval no. 2022-09-001). All personal and clinical data, including laboratory results and patient outcomes, were obtained from the patients' medical records. All the methods were carried out in accordance with relevant guidelines and regulations.
Demographic and postoperative profile of the study cohort
In a retrospective study of 46 patients undergoing cardiac surgeries, 26 (56.5%) were prescribed dapagliflozin, and 29 (63%) developed postoperative SGLT2i-related MA. Clinical and demographic characteristics of the study population are shown in [Table 1 ]. The average of age was 62.2 ± 11.14 year and BMI was 24.78 ± 3.3 kg/ \({m}^{2}\) . Of the 46 patients, 33 (71.3%) were men, and 42 (91.3%) had a past medical history of T2D. Blood glucose levels in both groups were investigated, and no statistical difference was found (117.7 ± 61.2 vs. 191.4 ± 35.3; p = 0.373). Furthermore, no statistically significant difference was noted between the two groups regarding the duration of MV support or the length of ICU stay. When evaluating the quantity of inotropes administered, instances of postoperative MA associated with SGLT2i exhibited a greater proportion of patients with a high inotropic demand (more than 2 agents); however, this variance was not statistically significant. (5 (17.2%) vs. 0 (0%); p = 0.140).
Clinical variables and incidence of SGLT2i -related postoperative MA
The potential risk factors, preoperative, intraoperative and postoperative variables, associated with SGLT2i-related postoperative MA were examined in univariate and multivariable regression analyses [Table 2 ]. We included factors with a p-value of less than 0.2 in the univariate analysis as significant variables in the multivariable logistic regression test. Additionally, we incorporated the presence of insulin-dependent T2D, a previously known precipitating factor for DKA, and the cessation time of more than 72 h for SGLT2i, as specified in the guidelines for the prevention of EDKA, into the analysis.
Preoperative factors
In the unadjusted analysis, several factors—including age, sex, BMI, premedical history of T2D, hemoglobin A1C (HbA1c) levels, preoperative glucose-insulin mixed infusion, use of other T2D medications, duration of SGLT2i cessation, and baseline estimated glomerular filtration rate (eGFR)—did not exhibit statistically significant differences between the postoperative MA group and the non-MA group. However, a prescription duration of SGLT2i of more than one week was associated with SGLT2i-related postoperative MA (OR, 11.7; 95% CI 1.65–237; p = 0.032*). In the multivariable analysis, this association remained significant (adjusted OR, 31.58; 95% CI 1.97–505.23; p = 0.014*).
Intraoperative factors
Regarding intraoperative factors, we assessed the types of operations conducted, the duration of these operations, the utilization of CPB, the duration of CPB running time, instances where CPB exceeded 60 min (referred as CPB 60), and the volume of ultrafiltration. In the univariate analysis, no statistically significant differences in intraoperative factors between the two groups were observed. In the adjusted model, which examined potential risk factors associated with postoperative MA or KA related to SGLT2i—including operation time and CPB 60—no statistical significance was found.
Postoperative factors
In both the univariate and multivariable models, the immediate postoperative administration of insulin infusion did not show any statistically significant differences between the group with postoperative MA related to SGLT2i and the non-MA group. (Unadjusted OR: 0.44; 95% CI 0.12–1.49; p = 0.197; Adjusted OR: 0.40; 95% CI 0.07–2.22; p = 0.298).
Subgroup analysis: impact of CPB running time on SGLT2i-related postoperative MA in patients with sufficient prescription duration
A subgroup analysis was conducted to assess the impact of CPB duration in patients with a sufficient SGLT2i prescription duration over 7 days (N = 40). Table 3 shows that a CPB duration ≤ 60 min is associated with a significantly higher risk of SGLT2i-related postoperative MA (HR 2.94; 95% CI 0.50–5.38, p = 0.018*).
For individuals with T2D, the prevalence of DKA or EDKA following the introduction of SGLT2i remains uncertain. In predominantly non-operative settings, in clinical trials involving SGLT2i for T2D treatment, the incidence of DKA ranged from 0.2 to 0.8 cases per 1000 patient-years 21 , 22 . The DKA incidence in T2D patients on SGLT2i might not be higher than in the wider diabetic population; however, clinical trials may not fully reflect real-world incidences. In a meta-analysis of 36 trials that evaluated T2D drug therapies, SGLT2i were the only class of drugs associated with an increased risk of DKA compared to other therapies 23 . A large-scale study from Canada and the United Kingdom, involving over 350,000 patients and 500 DKA cases, noted an increased risk with SGLT2i (dapagliflozin, empagliflozin, canagliflozin) compared to dipeptidyl peptidase-4 inhibitors, with respective incidence rates of 2.03 and 0.75 per 1,000 patient-years, and heightened DKA risks associated with dapagliflozin (HR: 1.86) and empagliflozin (HR: 2.52) 24 .
As known, several factors are recognized to precipitate DKA in the context of SGLT2i use. Patient characteristics such as insulin deficiency, commonly seen in latent autoimmune diabetes in adults, type 1 diabetes, or some individuals with long-standing T2D, have been noted 25 . Metabolic stressors like surgical interventions, vigorous exercise, myocardial infarctions, strokes, severe infections, and prolonged fasting have also been identified as significant contributors 25 , 26 . Other risk variables that could potentially predispose an individual to KA include pancreatic insulin deficiency, reductions in prescribed insulin dosage, caloric restriction, alcohol misuse and acute febrile illness.
Regarding perioperative patients with SGLT2i-related KA or MA, Thiruvenkatarajan et al. reported a prevalence of 89% for postoperative SGLT2i-related EDKA in their systematic review 15 . Blau. et al. using FDA data, found a comparable incidence rate of 71% (29 out of 51) 16 and Murugesan, K.B. observed a 70.8% (17 out of 24) for SGLT2i-associated EDKA in cardiac surgery patients 17 . Each study has different criteria, making comparison somewhat nonsensical; however, our study identified a 63% incidence (29 out of 46) of SGLT2i-related KA or MA, which aligns with previous studies and shows no significant statistical difference, as evidenced by p-values of 0.598 and 0.278, respectively.
Patients undergoing cardiac surgery represent a unique demographic at an intersection of heightened risk for postoperative MA or KA associated with SGLT2i, primarily because they have pre-existing CV disease, which often necessitates the prescription of SGLT2i. Moreover, these patients typically undergo fasting and experience the surgical stress. Despite these compounded risk factors, the risk-to-benefit ratio overwhelmingly supports the continued use of SGLT2i for managing CV disease or T2D, and current guidelines uphold this stance.
With the rising use of SGLT2i for treating CV disease, we observed six patients who developed postoperative KA associated with these medications. Table 4 details these patients with confirmed ketone bodies who suffered from SGLT2i-related postoperative KA. Notably, there was no consistent pattern regarding the hours of onset of ketoacidosis among these patients. For our first patient (case #1) was involved in extended diagnostic process such as bedside transthoracic echocardiography and cardiac angiography and resulting in a 24-h diagnostic delay. This initial experience facilitated more straightforward diagnoses in subsequent cases, highlighting the importance of considering SGLT2i related EDKA in the care of post-cardiac surgery patients to prevent diagnostic delays. Interestingly, one patient (case #6) without a prior medical history of T2D also exhibited postoperative KA. This implies that these incidents may not be limited only to patients with diabetes, but might also include those using SGLT2i. Therefore, we referred to this condition not as SGLT2i related EDKA, but as postoperative MA or KA related to SGLT2i, omitting the term 'diabetic’. A mechanism associated with SGLT2 inhibition appears to induce an early transition to fat metabolism, leading to an accumulation of ketones and resulting in KA or MA 27 .
We conducted a retrospective review to assess the incidence and risk factors of postoperative MA or KA related to SGLT2i in 46 cardiac surgery patients; 63% (29 patients) developed postoperative MA associated with SGLT2i [Fig. 1 ]. Although the threshold for MA might differ across clinical laboratories, we characterized SGLT2i-related postoperative MA based on the criteria of a pH < 7.35 and an HCO3- ≤ 22 mEq/L 28 . While our chosen threshold could be relatively liberal, we believe it is crucial to initially rule out benign but potentially concerning laboratory anomalies in post-cardiac surgery patients.
Cessation duration, according to the prescribing information 29 , 30 , dictates that SGLT2i should be discontinued 72 h prior to surgery.. In the univariate analysis, a cessation duration of more than 72 h in the preoperative period did not result in a significant decrease in the occurrence of SGLT2i-related postoperative MA or KA (unadjusted OR, 0.58; 95% CI 0.16–2.20; p = 0.42) [Table 2 ]. This lack of significance might suggest that the advised cessation period is shorter than actually needed.
A significant proportion of the cohort, 12 out of 46 (26%), were prescribed SGLT2i at the time of their CV diagnosis, within 1 month before their cardiac surgery. The univariate analysis indicated that a prescription duration exceeding 7 days significantly increased the incidence of SGLT2i-related postoperative MA (unadjusted OR, 11.7; 95% CI 1.65–237; p = 0.032*). This significance persisted in the multivariable analysis (adjusted OR, 31.58; 95% CI 1.97–505.23; p = 0.014*) [Table 2 ]. Our assumption is that the shift to fat metabolism induced by SGLT2 inhibition takes at least a few days, and patients who were prescribed for less than 7 days actually took SGLT2i for 3–5 days, considering the preoperative cessation period.
Furthermore, a subgroup analysis was performed to assess the impact of CPB running time on the occurrence of SGLT2i-related postoperative MA or KA. [Table 3 ] While ultrafiltration is employed to concentrate blood during CPB operations, reductions in both plasma colloid osmotic pressure and total protein concentration were noted following CPB use 19 . Following a decrease in protein levels, including drug-binding proteins, we hypothesized that the utilization of CPB might reduce the incidence of postoperative MA or KA associated with SGLT2i. Among patients prescribed SGLT2i for a sufficient duration (> 7 days, N = 40), those undergoing cardiac surgery with a short CPB running time (≤ 60 min) exhibited a higher risk of postoperative SGLT2i-related MA(OR, 2.94; 95% CI 0.50–5.38; p = 0.018*).
Rapid resolution of metabolic abnormalities induced by SGLT2i was observed after initiating insulin and dextrose infusions, as stated by Aaron Lau et al. in their case series 31 . Our findings were consistent with this; resolution of postoperative KA related to SGLT2i was easily achieved in our cases as well [Table 4 ]. Given that the ICU hyperglycemic management protocol for cardiac surgery patients targets a postoperative blood sugar range of 110 to 150 mg/dL and initiates a corrective protocol for levels above 150 mg/dL 20 , it was hypothesized that this protocol could incidentally manage undiagnosed cases of postoperative MA or KA related to SGLT2i. Despite this assumption, our analysis found that postoperative insulin infusion did not significantly affect the incidence of MA associated with SGLT2i (unadjusted OR, 0.44; 95% CI 0.12–1.49; p = 0.197; adjusted OR, 0.40; 95% CI 0.07–2.22; p = 0.298). [Table 2 ].
For our strategy in managing post-cardiac surgery patients without kidney disease, we utilized a GIK solution to treat KA associated with SGLT2i. Electrolyte imbalances in post-cardiac surgery patients can provoke arrhythmias, making the restoration of potassium levels especially crucial in this patient population.
Based on our findings, we advocate for the verification of ketone bodies using urine or serum ketone tests in post-cardiac surgery patients who present with high anion-gap MA, a condition solely attributable to the preoperative use of SGLT2i. As a time and cost-effective approach, we assess urine ketone levels through routine urinalysis, which provides results within 30 min in these patients. When patients present with a urine ketone level exceeding 2 +, we subsequently conduct a serum ketone test, which often requires 2–3 days for results, and initiate GIK solution administration concomitantly.
Limitations
Given the nature of a retrospective observational study, ketone body tests were not routinely included in postoperative laboratory tests, so ketone body confirmations could not be conducted for all patients. Regarding ICU management, there was a lack of uniformity among physicians, and unnecessary injections of sodium bicarbonate affected anion gap and pH levels. Furthermore, this issue spoiled anion gap, which is crucial for differentiating postoperative hyperchloremic metabolic acidosis, thereby preventing the establishment of an accurate exclusion criteria. As a single-center study, there were deviations in operation entities, with a scarcity of aortic surgeries.
The CV benefits of SGLT2i in patients with CV disease are undeniably profound. Not every case of KA is necessarily harmful, but the FDA-issued warnings about these risks have not been sufficiently integrated into perioperative care protocols. The lack of awareness complicates diagnosis and treatment of postoperative metabolic issues related to SGLT2i. However, our research shows that timely diagnosis and proper treatment can ease the patient's transition from ICU support to general wards.
In describing the postoperative metabolic issues observed in our study, we chose not to use the term 'diabetes' to highlight the possibility that these conditions might extend beyond patients with T2D. Further research is needed concerning the optimal duration for preoperative cessation of SGLT2i. Additionally, it is imperative to study the effects of CPB institution on SGLT2i-related postoperative KA in patients, including the assessment of SGLT2i-induced KA among nondiabetic individuals.
Data availability
The datasets generated during and/or analyzed during the current study are available from the corresponding author on reasonable request.
Abbreviations
Food and Drug administration
Sodium-glucose transporter 2 inhibitors
Type 2 diabetes
Cardiovascular
Heart failure with reduced ejection fraction
Heart failure with preserved ejection fraction
Heart failure with mildly reduced ejection fraction
Hospitalization for heart failure
Diabetic ketoacidosis
Euglycemic diabetic ketoacidosis
Ketoacidosis
Cardiopulmonary bypass
Metabolic acidosis
Intensive care unit
Glucose-insulin-potassium solution
Standard deviation
Arterial blood gas analyses
Mechanical ventilator
Body mass index
Hazard ratio
Confidence interval
Hemoglobin A1C
Estimated glomerular filtration rate
McMurray, J. V. et al. Dapagliflozin in patients with heart failure and reduced ejection fraction. N. Engl. J. Med. 381 (21), 1995–2008 (2019).
Article CAS PubMed Google Scholar
Packer, M. et al. Cardiovascular and renal outcomes with empagliflozin in heart failure. N. Engl. J. Med. 383 (15), 1413–1424 (2020).
Anker, S. D. et al. Empagliflozin in heart failure with a preserved ejection fraction. N. Engl. J. Med. 385 (16), 1451–1461 (2021).
Solomon, S. D. et al. Dapagliflozin in heart failure with mildly reduced or preserved ejection fraction. N. Engl. J. Med. 387 (12), 1089–1098 (2022).
Article PubMed Google Scholar
McDonagh, T. A. et al. 2021 ESC Guidelines for the diagnosis and treatment of acute and chronic heart failure. Eur. Heart J. 42 (36), 3599–3726 (2021).
Paul, A. H. et al. 2022 AHA/ACC/HFSA guideline for the management of heart failure. J. Card. Fail. 28 (5), e1–e167 (2022).
Article Google Scholar
Larkin, H. D. FDA expands empagliflozin heart failure indication. JAMA 327 (13), 1219–1219. https://doi.org/10.1001/jama.2022.3970 (2022).
Marilly, E. et al. SGLT2 inhibitors in type 2 diabetes: A systematic review and meta-analysis of cardiovascular outcome trials balancing their risks and benefits. Diabetologia 65 (12), 2000 (2022).
Liu, J. et al. Sodium-glucose co-transporter-2 inhibitors and the risk of diabetic ketoacidosis in patients with type 2 diabetes: A systematic review and meta-analysis of randomized controlled trials. Diabetes Obes. Metab. 22 (9), 1619 (2020).
FDA Drug Safety Communication: FDA revises labels of SGLT2 inhibitors for diabetes to include warnings about too much acid in the blood and serious urinary tract infections. Food and Drug Administration. http://www.fda.gov/Drugs/DrugSafety/ucm475463.htm (2021).
Heidi, G. & David, W. L. Endocrine emergencies. In Diabetic Emergencies: Ketoacidosis, Hyperglycemic Hyperosmolar State, and Hypoglycemia 5th edn (eds Heidi, G. & David, W. L.) 167–182 (Elsevier, 2020).
Google Scholar
Peters, A. L. et al. Euglycemic diabetic ketoacidosis: A potential complication of treatment with sodium-glucose cotransporter 2 inhibition. Diabetes Care 38 (9), 1687 (2015).
Article CAS PubMed PubMed Central Google Scholar
Fralick, M., Chneeweiss, S. & Patorno, E. Risk of diabetic ketoacidosis after initiation of an SGLT2 inhibitor. N. Engl. J. Med. 376 (23), 2300 (2017).
Ueda, P. et al. Sodium glucose cotransporter 2 inhibitors and risk of serious adverse events: Nationwide register based cohort study. BMJ 363 , k4365 (2018).
Article PubMed PubMed Central Google Scholar
Thiruvenkatarajan, V. et al. Perioperative diabetic ketoacidosis associated with sodium-glucose co-transporter-2 inhibitors: A systematic review. Br. J. Anaesth. 123 , 27–36 (2019).
Blau, J. E., Tella, S. H., Taylor, S. I. & Rother, K. I. Ketoacidosis associated with SGLT2 inhibitor treatment: Analysis of FAERS data. Diabetes Metab. Res. Rev. https://doi.org/10.1002/dmrr.2924 (2017).
Murugesan, K. B., Balakrishnan, S., Arul, A., Ramalingam, S. & Srinivasan, M. A retrospective analysis of the incidence, outcome and factors associated with the occurrence of euglycemic ketoacidosis in diabetic patients on sodium glucose co-transporter—2 inhibitors undergoing cardiac surgery. Ann. Card. Anaesth. 25 (4), 460–465 (2022).
Plewa, M. C., Bryant, M. & King-Thiele, R. Euglycemic Diabetic Ketoacidosis (StatPearls Publishing, 2022).
English, T. A. H., Stan, D. & Kirklin, J. W. Changes in colloid osmotic pressure during and shortly after open intracardiac operation. J. Thorac. Cardiovasc. Surg. 61 (3), 338–341 (1971).
Bojar, R. M. Manual of Perioperative Care in Adult Cardiac Surgery 6th edn. (Wiley, 2020).
Ngozi, E., Mehul, D., Kirk, W. & Gary, M. Diabetic ketoacidosis and related events in the Canagliflozin type 2 diabetes clinical program. Diabetes Care 38 , 1680–1686 (2015).
Zinman, B., Wanner, C. & Lachin, J. M. Empagliflozin, cardiovascular outcomes, and mortality in type 2 diabetes. N. Engl. J. Med. 373 (22), 2117–2128 (2015).
Shi, Q. et al. Benefits and harms of drug treatment for type 2 diabetes: Systematic review and network meta-analysis of randomized controlled trials. BMJ 381 , e074068 (2023).
Douros, A. et al. Sodium-glucose cotransporter-2 inhibitors and the risk for diabetic ketoacidosis: A multicenter cohort study. Ann. Intern. Med. 173 (6), 417–425 (2020).
Handelsman, Y. et al. American association of clinical endocrinologists and American college of endocrinology position statement on the association of SGLT-2 inhibitors and diabetic ketoacidosis. Endocr. Pract. 22 (6), 753–762 (2016).
Fitchett, D. A safety update on sodium glucose co-transporter 2 inhibitors. Diabetes Obes. Metab. 21 (Suppl 2), 34–42 (2019).
Ogawa, W. & Sakaguchi, K. Euglycemic diabetic ketoacidosis induced by SGLT2 inhibitors: Possible mechanism and contributing factors. J. Diabetes Investig. 7 (2), 135–138 (2016).
Fenves, A. Z. & Emmett, M. Approach to patients with high anion gap metabolic acidosis: Core curriculum 2021. Am. J. Kidney Dis. 78 (4), 590–600 (2021).
Wilmington, D. E. Farxiga (Dapagliflozin) [Prescribing Information] (AstraZeneca Pharmaceuticals LP, 2023).
Ridgefield, C. T. Jardiance (Empagliflozin) [Prescribing Information] (Boehringer Ingelheim Pharmaceuticals Inc, 2023).
Lau, A. et al. Perioperative implications of sodium-glucose cotransporter-2 inhibitors: A case series of euglycemic diabetic ketoacidosis in three patients after cardiac surgery. Can. J. Anaesth. 65 (2), 188–193 (2018).
Download references
Author information
Authors and affiliations.
Department of Cardiovascular and Thoracic Surgery, Ewha Womans University Medical Center, Seoul, Republic of Korea
Hyeon A Kim & Joo Yeon Kim
Department of Cardiovascular and Thoracic Surgery, Incheon Sejong Hospital, Incheon, Republic of Korea
Young Hwan Kim, Young Tak Lee & Pyo Won Park
You can also search for this author in PubMed Google Scholar
Contributions
Conceptualization and design: HA Kim, JY Kim. Analysis and interpretation: HA Kim. Data collection: HA Kim, JY Kim. Writing original draft, Fig. 1 and Tables 1 , 2 , 3 , 4 : HA Kim. Review and editing: All authors. Statistical analysis: HA Kim. Funding: N/A. The requirement for informed consent was waived due to the retrospective nature of the study. All personal and clinical data, including laboratory results and patient outcomes, were obtained from the patients' medical records.
Corresponding author
Correspondence to Joo Yeon Kim .
Ethics declarations
Competing interests.
The authors declare no competing interests.
Additional information
Publisher's note.
Springer Nature remains neutral with regard to jurisdictional claims in published maps and institutional affiliations.
Rights and permissions
Open Access This article is licensed under a Creative Commons Attribution 4.0 International License, which permits use, sharing, adaptation, distribution and reproduction in any medium or format, as long as you give appropriate credit to the original author(s) and the source, provide a link to the Creative Commons licence, and indicate if changes were made. The images or other third party material in this article are included in the article's Creative Commons licence, unless indicated otherwise in a credit line to the material. If material is not included in the article's Creative Commons licence and your intended use is not permitted by statutory regulation or exceeds the permitted use, you will need to obtain permission directly from the copyright holder. To view a copy of this licence, visit http://creativecommons.org/licenses/by/4.0/ .
Reprints and permissions
About this article
Cite this article.
Kim, H.A., Kim, J.Y., Kim, Y.H. et al. Missed postoperative metabolic acidosis associated with sodium-glucose transporter 2 inhibitors in cardiac surgery patients: a retrospective analysis. Sci Rep 14 , 8087 (2024). https://doi.org/10.1038/s41598-024-58853-7
Download citation
Received : 13 September 2023
Accepted : 03 April 2024
Published : 06 April 2024
DOI : https://doi.org/10.1038/s41598-024-58853-7
Share this article
Anyone you share the following link with will be able to read this content:
Sorry, a shareable link is not currently available for this article.
Provided by the Springer Nature SharedIt content-sharing initiative
By submitting a comment you agree to abide by our Terms and Community Guidelines . If you find something abusive or that does not comply with our terms or guidelines please flag it as inappropriate.
Quick links
- Explore articles by subject
- Guide to authors
- Editorial policies
Sign up for the Nature Briefing newsletter — what matters in science, free to your inbox daily.


An official website of the United States government
The .gov means it’s official. Federal government websites often end in .gov or .mil. Before sharing sensitive information, make sure you’re on a federal government site.
The site is secure. The https:// ensures that you are connecting to the official website and that any information you provide is encrypted and transmitted securely.
- Publications
- Account settings
Preview improvements coming to the PMC website in October 2024. Learn More or Try it out now .
- Advanced Search
- Journal List
- v.3(5); Sep-Oct 2021
Metabolic Acidosis and Cardiovascular Disease in CKD
David collister.
1 Department of Medicine, McMaster University, Hamilton, ON, Canada
Thomas W. Ferguson
2 Department of Internal Medicine, Faculty of Health Sciences, Max Rady College of Medicine, University of Manitoba, Winnipeg, MB, Canada
Susan E. Funk
3 Strategic Health Resources, La Cañada, CA
Nancy L. Reaven
Vandana mathur.
4 Mathur Consulting, Woodside, CA
Navdeep Tangri
Associated data, rationale & objective.
Metabolic acidosis related to chronic kidney disease (CKD) is associated with an accelerated decline in glomerular filtration rate (GFR) and the development of end-stage kidney disease. Whether metabolic acidosis is associated with cardiovascular (CV) events in patients with CKD is unclear.
Study Design
Retrospective cohort study.
Setting & Participants
The Optum De-identified Electronic Health Records Dataset, 2007–2017, was used to generate a cohort of patients with non-dialysis-dependent CKD who had at least 3 estimated GFR < 60 mL/min/1.73 m 2 . Patients with metabolic acidosis (serum bicarbonate 12 to <22 mEq/L) or normal serum bicarbonate (22‒29 mEq/L) at baseline were identified by 2 consecutive measurements 28‒365 days apart.
Serum bicarbonate as a continuous variable.
Primary outcome was a composite of major adverse cardiovascular events (MACE+). Secondary outcomes included individual components of the composite outcome.
Analytical Approach
Cox proportional hazards models to evaluate the association between 1-mEq/L increments in serum bicarbonate and MACE+.
A total of 51,558 patients were evaluated, 34% had metabolic acidosis. The median follow-up period was 3.9–4.5 years, depending on the outcome assessed. The adjusted hazard ratio (HR) for MACE+ was 0.964 (95% CI, 0.961–0.968). For the individual components of incident heart failure (HF), stroke, myocardial infarction (MI), and CV death, HRs were 0.98 (95% CI, 0.97–0.98), 0.98 (95% CI, 0.97–0.99), 0.96 (95% CI, 0.96–0.97), and 0.94 (95% CI, 0.93–0.94), respectively, for every 1-mEq/L increase in serum bicarbonate.
Limitations
Possible residual confounding.
Conclusions
Metabolic acidosis in CKD is associated with an increased risk of MACE+ as well as the individual components of incident HF, stroke, MI, and CV death. Randomized controlled trials evaluating treatments for the correction of metabolic acidosis in CKD to prevent CV events are needed.
Graphical abstract

Plain-Language Summary
The retention of acid in patients with kidney disease related to diet and reduced kidney function (known as “metabolic acidosis”) is associated with both a faster loss of kidney function and kidney failure (dialysis or kidney transplant). Whether metabolic acidosis is harmful to the heart and brain is unknown. In this study of over 50,000 patients with kidney disease not on dialysis from the United States over a 10-year period, metabolic acidosis was associated with an increased risk of heart failure, stroke, heart attack, and death, independent of other risk factors for these negative outcomes. Studies evaluating new drugs to correct metabolic acidosis and reduce heart disease in patients with kidney disease are needed.
Cardiovascular events are the leading cause of death among patients with chronic kidney disease (CKD). In a study of over 1 million patients with CKD, the risk of cardiovascular events, defined as hospitalization for coronary artery disease, heart failure (HF), and ischemic stroke, increased progressively with advancing CKD stage. 1 The high burden of cardiovascular disease in this population reflects traditional cardiovascular risk factors as well CKD-specific risk factors. 2
Metabolic acidosis is common in later-stage CKD because of the metabolism of dietary protein, generation of nonvolatile acids, and impaired net acid excretion. 3 , 4 The prevalence of metabolic acidosis, defined by a reduced serum bicarbonate level, increases as glomerular filtration rate (GFR) decreases. 5 , 6 Both the decrease in serum bicarbonate and net acid excretion in CKD are associated with an accelerated decline in GFR and end-stage kidney disease (ESKD) in addition to adverse musculoskeletal effects and increased mortality. 7 , 8 , 9 , 10 , 11 , 12 Metabolic acidosis is postulated to be a CKD-specific cardiovascular risk factor because of its negative effects on the cardiovascular system, including inflammation and activation of the renin-angiotensin-aldosterone system (RAAS). 13 , 14 , 15
Prior studies have not clearly elucidated an effect of metabolic acidosis on the risk of cardiovascular events. 7 , 16 , 17 , 18 , 19 However, these observational studies were limited by their size, number of events, incomplete adjustment of covariates, and included cohorts with and without baseline cardiovascular disease. We report the findings of a large, observational, retrospective, community-based cohort study of over 51,000 adults with stages 3–5 CKD to examine the association of metabolic acidosis with HF and major adverse cardiovascular events.
Study Design and Data Sources
This was an observational, retrospective cohort study of US patients with CKD and serum bicarbonate measurements who had at least 2 years of longitudinal follow-up observation or who died during the 2-year period. Data from January 1, 2007, to March 31, 2017, was extracted from the Optum EHR+Integrated Electronic Health Record (EHR) dataset, which is deidentified in compliance with the Health Insurance Portability and Accountability Act (HIPAA). This database is a longitudinal clinical repository that includes 81 million insured and uninsured patients from large health care providers in all 50 US states and Puerto Rico. Data elements were derived from inpatient and outpatient EHRs and administrative systems, including laboratory results, medication prescriptions, coded diagnoses and procedures, and provider notes extracted by natural language processing. Data cleaning by investigators included application of validity parameters to reported laboratory values ( Table S1 ). Neither informed consent nor institutional review board approval was required as the study accesses deidentified information in compliance with HIPAA regulations and requirements.
Study Cohort
For inclusion in the initial database extract, patients were required to have at least 3 estimated GFR (eGFR) results < 60 mL/min/1.73 m 2 and at least 3 serum bicarbonate results, with at least 1 result between 12 and 29 mEq/L and at least 1 year of EHR activity. For entry into the study cohort, patients were required to have 2 consecutive valid serum bicarbonate results between 28 and 365 days apart, both between either 12 and <22 mEq/L (metabolic acidosis) or 22 and 29 mEq/L (normal per laboratory reference ranges). The first of the 2 values was considered the baseline serum bicarbonate level, and the date of the test was considered the index date. Serum bicarbonate and eGFR values collected during hospital inpatient admissions or emergency department visits with a concurrent diagnosis code of acute kidney injury ( Table S1 ) were excluded because these may have represented acute events. In addition, patients were required to have at least 1 year of preindex EHR activity (eg, clinic visit, health care facility encounter, or laboratory test), and at least 2 years of postindex activity, unless the patient died within this 2-year period.
The eGFR was calculated using the Chronic Kidney Disease Epidemiology Collaboration (CKD-EPI) equation. 20 Baseline eGFR was defined as the mean of eGFR values from the 90 days preceding the last eGFR test on or before the index date. Patients who had evidence of long-term dialysis or kidney transplantation at baseline (diagnosis or procedure code, or outpatient eGFR result ≤10 mEq/L; Table S1 ) were excluded. We created analysis groups for specific outcomes that excluded patients with a history of those outcomes.
To ensure enough patients with decreased serum bicarbonate levels, an iterative patient selection algorithm was used to oversample patients with metabolic acidosis. Specifically, the selection algorithm examined records starting after 1 year of patient activity and initially searched for a qualifying pair of consecutive bicarbonate values between 12 and <22 mEq/L before examining bicarbonate values between 22 and 29 mEq/L.
The primary predictor was baseline serum bicarbonate. Information regarding the use of specific serum bicarbonate assays was not available, but they likely varied depending on local laboratory practices. Baseline serum bicarbonate was treated as a continuous variable. Demographic and clinical variables known or hypothesized to be associated with cardiovascular events were assessed at the index date and included age, sex, race, diabetes mellitus (DM), hypertension, heart failure, coronary artery disease (CAD), peripheral vascular disease (PVD), comorbidity burden as measured by the Charlson Comorbidity Index (CCI), 21 and baseline eGFR, hemoglobin, and serum albumin values. Individuals born in 1928 or earlier were assigned 1928 as their birth year to ensure HIPAA compliance. Comorbidities were defined by International Classification of Diseases, Ninth Revision, Clinical Modification (ICD-9-CM) and ICD-10-CM codes according to the US Renal Data System definitions. 22 Baseline comorbidities were assessed by single occurrence of diagnosis codes and used all available data before the index date. Complete variable definitions are provided in Table S1 .
The primary outcome was a composite of major adverse cardiovascular events (MACE+), defined as myocardial infarction (MI), ischemic stroke, incident heart failure (a new heart failure diagnosis in a patient without evidence of heart failure at baseline), heart failure admission (an inpatient admission with a concurrent diagnosis of heart failure in patients with a history of heart failure), or cardiovascular death (death within the same or next calendar month as discharge from an inpatient hospital stay involving a diagnosis code for heart failure, stroke, or MI). Secondary outcomes of incident heart failure, stroke, MI, and cardiovascular death were analyzed individually. All outcomes were identified using ICD-9-CM and ICD-10-CM diagnosis codes in all available postindex data ( Table S2 ). Patients were excluded from analysis of secondary outcomes as follows: from incident heart failure if they had a history of heart failure before the index date and from stroke or MI analyses if they had any diagnosis code for stroke or MI, respectively, for 12 months before the index date.
Statistical Analysis
Patient characteristics were compared at baseline between the metabolic acidosis versus normal serum bicarbonate groups using the χ 2 test, t test, or 2-tailed Wilcoxon rank-sum test, as appropriate. Multivariable Cox proportional hazards models were used to model the time to MACE+, incident heart failure, stroke, MI, and cardiovascular death in each group. Patients were censored at death or loss to follow-up (defined as the patient’s last known interaction with the health system through end of data availability in March 2017), but not at the development of ESKD. Fine-Gray subdistribution hazards models were used to evaluate the competing risk of noncardiovascular death in the MACE+ model. All covariates were forced into the final multivariable models. Models were adjusted for age, sex, race, eGFR, DM, hypertension, CAD, PVD, hemoglobin, and serum albumin. The proportional hazards assumption was tested by plotting Schoenfeld residuals against time and visual inspection for uniformity. Patients with missing data for any independent variable were excluded from the analysis; missing data were only relevant for laboratory measurements. Unadjusted incidence rates of MACE+, incident heart failure, stroke, MI, and cardiovascular death were assessed over the 2-year period of required data and compared between patients with metabolic acidosis or normal serum bicarbonate at baseline by χ 2 tests.
All statistical analyses were performed using SAS/STAT software version 9.4 (SAS, Inc). P < 0.05 was considered statistically significant.
Additional Analyses
The effect of oversampling of patients with metabolic acidosis was evaluated by constructing alternative patient cohorts without oversampling, in which the effect of serum bicarbonate on MACE+ and the individual components of incident heart failure, stroke, and MI were evaluated in Cox proportional hazards models with the same predictors. We also considered a Cox proportional hazards model with additional adjustment for urinary albumin-to-creatinine ratio (UACR) at baseline (not included in the primary analysis because of the degree of missing data).
Study Population and Characteristics
Of 81 million patient records located in the Optum EHR+Integrated Electronic Health Record database, 319,126 met the criteria for inclusion in the database extract. From within this extract, we identified a study population of 51,558 patients who met the data sufficiency requirements, had stages 3–5 CKD and no indication of dialysis or transplant, and who qualified for inclusion in the metabolic acidosis group (n = 17,350) or normal serum bicarbonate group (n = 34,208) ( Fig 1 ). Baseline demographics and clinical characteristics are presented for the overall study cohort ( Table 1 ) and for the incident heart failure, stroke, and MI analysis groups separately ( Table S3 ). Individuals with metabolic acidosis were younger (mean age, 70 vs 74 years) and more likely to be African American (15% vs 7%). They also had more advanced CKD (eGFR 37 vs 43 mL/min/1.73 m 2 ), a greater burden of comorbidities (CAD, DM, heart failure, hypertension, PVD), a higher comorbidity burden as measured by the CCI, and higher UACR (277 vs 127 mg/g). The median follow-up period was 3.9 years in the MACE+ model, 4.5 years in the heart failure model, and 4.3 years in the stroke and MI models. The laboratory values missing in metabolic acidosis group versus normal serum bicarbonate group were UACR (22% vs 46%), serum albumin (12% vs 26%), and hemoglobin (4% vs 17%).

Study cohort selection diagram. MACE+ analysis group and cardiovascular death analysis group: n = 51,558; complete data, n = 36,917. Incident HF analysis group: n = 41,529, with 10,029 excluded; complete data, n = 28,817. Stroke analysis group: n = 47,103, with 4,455 excluded; complete data, n = 33,325. MI analysis group: n = 48,789, with 2,769 excluded; complete data, n = 34,573. Abbreviations: AKI, acute kidney injury; BL, baseline; CKD, chronic kidney disease; CKD-EPI, Chronic Kidney Disease Epidemiology Collaboration; CV, cardiovascular; eGFR, estimated glomerular filtration rate; EHR, electronic health record; HF, heart failure; MACE+, major adverse cardiovascular event, defined as any MI, any stroke, incident HF (a new HF diagnosis in a patient without HF at baseline), or HF admission (an inpatient admission with a concurrent diagnosis of HF in a patient with comorbid HF at baseline), or cardiovascular death (death within the same or next calendar month as discharge from an inpatient hospital stay involving a diagnosis code for HF, stroke, or MI); MI, myocardial infarction.
Table 1
Demographics and Clinical Characteristics of the Study Cohort
Values are presented as number (percentage), mean ± SD, or median [interquartile range]. UACR and other characteristics with non-normal distributions were assessed using the nonparametric Wilcoxon rank sum test. The reference race was White. Conversion factor for units: serum calcium in mg/dL to μmol/L, × 0.2495. Number of patients with data are reported for each test in Table S3C .
Abbreviations: CCI, Charlson Comorbidity Index; CKD, chronic kidney disease; eGFR, estimated glomerular filtration rate; SD, standard deviation; UACR, urinary albumin-creatinine ratio.
Primary Outcome
Of the entire study population, 24,873 (48%) experienced a MACE+ event within 2 years; metabolic acidosis at baseline was associated with a higher rate of MACE+ events (58% vs 44% ( Fig 2 ). In the Cox proportional hazards model, every 1-mEq/L increase in serum bicarbonate was associated with a 4% decrease in the risk of MACE+ events (adjusted hazard ratio [HR], 0.96 [95% CI, 0.96–0.97]) ( Table 2 ). The Fine-Gray model using the same covariates and including noncardiovascular death as a competing risk showed similar results (HR, 0.98 [95% CI, 0.98-0.99]) ( Table S4 ). Over up to 10 years, 32,526 patients experienced a MACE+ outcome (161 events per 1,000 patient-years).

Unadjusted 2-year incidence rates of MACE+, incident heart failure, stroke, myocardial infarction, and cardiovascular death in the metabolic acidosis versus normal serum bicarbonate groups. Abbreviations: CV, cardiovascular; HF, heart failure; MACE+, major adverse cardiovascular event, defined as any MI, any stroke, incident HF (a new HF diagnosis in a patient without HF at baseline), or HF admission (an inpatient admission with a concurrent diagnosis of HF in a patient with comorbid HF at baseline), or CV death (death within the same or next calendar month as discharge from an inpatient hospital stay involving a diagnosis code for HF, stroke, or MI); MI, myocardial infarction. ∗ P < 0.001 by χ 2 test.
Table 2
Factors Associated With MACE+ and Secondary Outcomes, up to 10 Years
Values are hazard ratio (95% confidence interval). The reference race was White. Individuals with baseline HF or with stroke or MI within the year preceding the index date were excluded from the incident HF, stroke, and MI analyses, respectively.
Abbreviations: CAD, coronary artery disease; CV, cardiovascular; eGFR, estimated glomerular filtration rate; HF, heart failure; MACE+, major adverse cardiovascular event, defined as any MI, any stroke, incident HF (a new HF diagnosis in a patient without HF at baseline), or HF admission (an inpatient admission with a concurrent diagnosis of HF in a patient with comorbid HF at baseline), or CV death (death within the same or next calendar month as discharge from an inpatient hospital stay involving a diagnosis code for HF, stroke, or MI); MI, myocardial infarction; NA, not applicable; PVD, peripheral vascular disease.
Secondary Outcomes
The 2-year unadjusted incidence rates of incident heart failure, stroke, MI, and cardiovascular death in patients with metabolic acidosis versus patients with normal serum bicarbonate were 30% versus 23%, 20% versus 17%, 17% versus 12%, and 13% versus 5%, respectively (all P < 0.001) ( Fig 2 ). In the Cox proportional hazards models, the adjusted hazard ratios for incident heart failure, stroke, MI, and cardiovascular death were 0.98 (95% CI, 0.97–0.98), 0.98 (95% CI, 0.97–0.99), 0.96 (95% CI, 0.96−0.97), and 0.94 (95% CI, 0.93–0.94), respectively ( Table 2 ), with a risk reduction of 2%, 2%, 4%, and 6%, respectively, for each 1-mEq/L increase in serum bicarbonate.
In the sensitivity analysis that did not include oversampling to identify metabolic acidosis, each 1-mEq/L increase in serum bicarbonate was associated with MACE+ and the secondary outcomes of stroke, and MI (all P < 0.001) but not incident heart failure ( P = 0.08) ( Table S5 ). In the sensitivity analysis controlling for UACR, the adjusted hazard ratio associated with a 1−mEq/L increase in serum bicarbonate was unchanged: 0.96 (95% CI, 0.96–0.97) ( Table S6 ).
In this large, retrospective cohort study of 51,558 patients with nondialysis stages 3–5 CKD, a higher serum bicarbonate value was independently associated with a reduced risk of incident heart failure, stroke, MI, and a composite that included cardiovascular death and heart failure admissions (MACE+), after adjustment for demographics, comorbidities, and laboratory values that included eGFR. Given the high cardiovascular event rates in the CKD population 1 and underdiagnosis and undertreatment of metabolic acidosis, 7 , 23 these findings suggest a potential opportunity for reduction in the risk of cardiovascular events.
Previous studies of patients with diabetes and heart failure demonstrated that metabolic acidosis was associated with an increased risk of cardiovascular events. In the Australian Fremantle Diabetes Study, lower serum bicarbonate levels were associated with an increased risk of cardiovascular events. 24 In addition, data from a Korean heart failure registry showed that serum pH < 7.36 was associated with decreased 30-day and 1-year survival, and a decrease in serum bicarbonate (and not hypercapnia) was associated with mortality. 25 In a study of a healthy population without cardiovascular disease, metabolic alkalosis (not metabolic acidosis) was associated with an increase in incident heart failure but not cardiovascular events; 26 however, these findings may have been confounded by an increased need for diuretics among individuals with metabolic alkalosis.
To our knowledge, this is the largest observational study to date to report an association between metabolic acidosis in CKD and an increased risk of cardiovascular events, including heart failure, stroke, and MI. Previous studies in CKD populations have yielded inconsistent results regarding an association between metabolic acidosis and cardiovascular events, 7 , 16 , 18 likely due to several differences among studies, including study design, patient population, sample size, and length of follow-up. In a post hoc analysis of the Irbesartan Diabetic Nephropathy Trial (IDNT) and the Reduction of Endpoints in NIDDM with the Angiotensin II Antagonist Losartan (RENAAL) trial, there was no association between serum bicarbonate and adjudicated MI, stroke, coronary revascularization, or hospitalization for heart failure. 17 In the Chronic Renal Insufficiency Cohort (CRIC) study, adjudicated heart failure was more common in those with a serum bicarbonate level > 26 mEq/L (HR, 1.66 [95% CI, 1.22‒2.33]; P < 0.001) but not in those with a serum bicarbonate level < 22 mEq/L, even after excluding those with alkali therapy, chronic obstructive pulmonary disease (COPD), or a history of cardiovascular disease. In the CRIC study, for every 1-mEq/L increase in serum bicarbonate level > 24 mEq/L, there was a 14% higher risk of heart failure (HR, 1.14 [95% CI, 1.03‒1.26]), 7 but there was no difference in adjudicated MI, stroke, or PVD by acid-base status. 7 , 16 Our analysis differs from the CRIC study in that we excluded all patients with serum bicarbonate levels > 29 mEq/L because we were primarily interested in the risk associated with metabolic acidosis compared with patients with normal serum bicarbonate levels, and we did not want to include patients with respiratory acidosis and metabolic compensation. In a recent retrospective cohort study of kidney transplant recipients without cardiovascular events, a serum bicarbonate level < 20 mEq/L (but not < 22 mEq/L) was associated with an increased risk of cardiovascular events (HR, 2.00 [95% CI, 1.29‒3.10]), which was driven by ischemic events (HR, 2.28 [95% CI, 1.34‒3.90]) but not arrhythmic events or heart failure. 18 Our relative risks are smaller in magnitude but similar in direction, and may reflect differences in baseline cardiovascular risk profile of kidney transplant recipients.
The mechanism by which metabolic acidosis increases the risk of cardiovascular events is unclear. Metabolic acidosis is associated with inflammation, 10 which may contribute to endothelial dysfunction and an increased risk of cardiovascular events. 27 , 28 It is also associated with activation of the RAAS, as shown by elevated levels of aldosterone and endothelin, 13 , 14 , 15 which may contribute to kidney injury and heart failure. 29 , 30 In an open-label, placebo-controlled, pilot crossover trial examining the effect of oral sodium bicarbonate on vascular function in 20 patients with CKD and metabolic acidosis, sodium bicarbonate improved endothelial function as measured by brachial artery flow-mediated dilation. 31 Serum bicarbonate is associated with insulin resistance in CKD, as evidenced by a decrease in adiponectin production by adipocytes, which in turn is associated with cardiovascular events, as adipokines are potentially implicated in the pathogenesis of cardiovascular disease. 32 , 33 , 34 , 35 Adipokines are also implicated in the pathogenesis of heart failure via remodeling due to hypertrophy, fibrosis, and cell death. 36 Acidosis also decreases myocardial contractility and β-adrenergic response, but in the CRIC study metabolic acidosis did not correlate with left ventricular geometry, function, or relaxation. 37 , 38 , 39 Metabolic alkalosis, however, may be harmful because of increased precipitation of vascular calcification and activation of myoblast signaling pathways that influence cell survival. 40 , 41
Although most patients with CKD are not currently treated for metabolic acidosis, 7 , 23 existing treatments may negate the potential cardiovascular benefits of treatment. For example, although treatment of metabolic acidosis with sodium bicarbonate reduces the rate of CKD progression, it has been associated with increased blood pressure and/or requirement for increased antihypertensive treatment in some studies. 42 Furthermore, the beneficial effects of RAAS blockade on cardiovascular outcomes in patients with CKD are negated by a high sodium intake. 43 Even though the recently conducted BASE trial did not show a change in blood pressure with sodium bicarbonate treatment, there was a dose-dependent increase in albuminuria, and the trial was conducted in a nonacidotic population. 44 Novel treatments may avoid such trade-offs. For example, veverimer, a counterion-free, nonabsorbed polymer that selectively binds and removes hydrogen and chloride from the gastrointestinal tract, effectively corrected acidosis related to CKD compared with placebo and improved physical function and is currently being investigated in an ongoing trial for the slowing of CKD progression. 45 , 46 , 47
The strengths of this study include its size, power, adjustment of most clinically relevant covariates, and generalizability given its diverse patient population. CKD and metabolic acidosis were both identified conservatively, with metabolic acidosis requiring consecutive abnormal laboratory results separated in time and CKD established by the mean of laboratory values over 3 months.
However, the study has several limitations. The requirement of a minimum of 28 days to establish a confirming index serum bicarbonate value introduces survival bias that investigators weighed against the risk of incorrectly identifying metabolic acidosis through the use of a single serum bicarbonate measurement. Comorbidities and outcomes were identified using ICD codes, but they have not been validated in this EHR. 48 Our analysis did not adjust for all factors that can influence both acid-base status and outcomes, including dietary intake (protein and fruits/vegetables 49 , 50 ), respiratory disorders (other than COPD which is included in the CCI), 51 diuretics, and other covariates that may influence both serum bicarbonate and cardiovascular risk (eg, smoking, blood pressure, 52 and statins 53 ). Metabolic acidosis was only assessed at baseline and was not modeled as a time-varying covariate, which does not reflect longitudinal CKD care (ie, GFR decline, treatment with diuretics, and oral alkali). Oversampling could have identified less severe or persistent metabolic acidosis, but this is not supported by the sensitivity analysis. Residual confounding is a concern because individuals with metabolic acidosis related to CKD may have a phenotype independently associated with cardiovascular events. Finally, ascertainment of serum bicarbonate may vary by laboratory and method of measurement and was not captured by blood gases, and as such there may be a risk of misclassification.
In conclusion, a lower serum bicarbonate level was associated with a higher risk of MACE+ in patients with CKD. Further research is needed to explain the mechanisms that drive the association between metabolic acidosis and cardiovascular events, and randomized controlled trials are needed to examine if the correction of acidosis in CKD improves cardiovascular morbidity and mortality.
Article Information
Authors’ full names and academic degrees.
David Collister, MD, Thomas W. Ferguson, MSc, Susan E. Funk, MBA, Nancy L. Reaven, MA, Vandana Mathur, MD, and Navdeep Tangri, MD, PhD.
Authors’ Contributions
Research idea and study design: NT, VM, NR, SF; data acquisition: NR, SF; data analysis/interpretation: NT, VM, DC, NR, SF, TF; statistical analysis: NR, SF, TF. Each author contributed important intellectual content during manuscript drafting or revision and accepts accountability for the overall work by ensuring that questions pertaining to the accuracy or integrity of any portion of the work are appropriately investigated and resolved.
This work was supported by Tricida, Inc. Tricida did not have a role in study design, analysis, reporting or the decision to submit for publication. Tricida provided funding for data collection.
Financial Disclosure
Drs Mathur and Tangri and Ms Reaven report consultancy, personal fees, and equity ownership from Tricida, Inc, related to the submitted work. Mr Ferguson and Ms Funk report consultancy and personal fees from Tricida, Inc. Dr Collister has nothing to disclose.
Acknowledgements
The authors would like to thank Jason Tuffree, MSc (Ashfield Healthcare Communications, Inc.), for medical writing support, and Jun Shao, Ph.D. (Tricida, Inc), and Kathryn Boorer, PhD, for editorial support.
Peer Review
Received November 18, 2020. Evaluated by 2 external peer reviewers, with direct editorial input by the Statistical Editor, an Associate Editor, and the Editor-in-Chief. Accepted in revised form April 4, 2021.
Complete author and article information provided before references.
Supplementary File (PDF)
Table S1: Sources, measurements, and definitions.
Table S2: Comorbidity and outcome definitions by diagnosis code.
Table S3: (A) Patient characteristics by outcome analysis group. (B) Patients with lab values, analysis groups, N (%). (C) Patient with lab values, study cohort.
Table S4: Fine and Gray model of major adverse cardiovascular event (MACE+) outcome, using noncardiovascular death as the competing event (n = 36,917).
Table S5: Cox proportional hazard models from sensitivity analysis without oversampling.
Table S6: Sensitivity analyses: adding log urine ACR as covariate associated with major adverse cardiovascular event (MACE+) outcome.
Supplementary Material
Tables S1-S6.
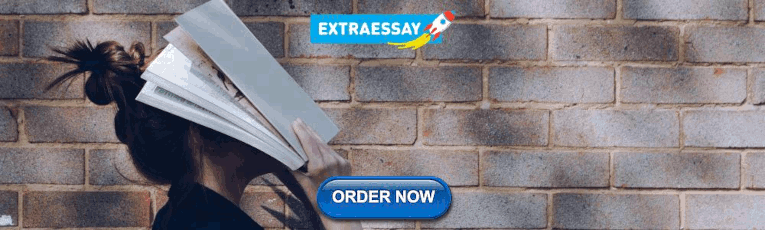
COMMENTS
Acid-base disorders, including metabolic acidosis, are disturbances in the homeostasis of plasma acidity. Any process that increases the serum hydrogen ion concentration is a distinct acidosis. The term acidemia is used to define the total acid-base status of the serum pH. For example, a patient can have multiple acidoses contributing to a net acidemia. Its origin classifies acidosis as either ...
Metabolic acidosis is a condition in which acids build up in your body. Causes include untreated diabetes, the loss of bicarbonate in your body and kidney conditions. Symptoms include an accelerated heartbeat, confusion and fatigue. Blood and urine tests can help diagnose it.
Patients, especially children, also may present with nausea, vomiting, and decreased appetite. The clinical history in metabolic acidosis is helpful in establishing the etiology when symptoms relate to the underlying disorder. The age of onset and a family history of acidosis may point to inherited disorders, which usually start during childhood.
Show Details Hide Details . With metabolic acidosis, "acidosis" refers to a process that lowers blood pH below 7.35, and "metabolic" refers to the fact that it's a problem caused by a decrease in the bicarbonate HCO3− concentration in the blood. ... If the urinary anion gap in a patient with non-anion gap metabolic acidosis is -30 ...
Acid-base balance is usually assessed in terms of the bicarbonate-carbon dioxide buffer system: Dissolved CO 2 + H 2 O ↔ H 2 CO 3 ↔ HCO 3- + H +. The ratio between these reactants can be expressed by the Henderson-Hasselbalch equation. By convention, the pKa of 6.10 is used when the denominator is the concentration of dissolved CO 2, and ...
The Henderson-Hasselbalch method defines metabolic acidosis by the presence of an acid-base imbalance associated with a plasma bicarbonate concentration below 20 mmol/L. The association of this imbalance with decreased pH is called "acidemia," which is often described as severe when the pH is equal to or below 7.20.
People with metabolic acidosis have a blood bicarbonate level between 12-22 mEq/L, while people with severe metabolic acidosis fall below 12 mEq/L. There are medicines that can add more bicarbonate to your body to balance out the acid. One way to increase bicarbonate levels is through alkali therapy, which could include taking sodium bicarbonate.
Acute metabolic acidosis is relatively common among seriously ill patients, with one study showing that the disorder affected approximately 64% of patients in a large intensive care unit in the US ...
To diagnose metabolic acidosis the clinician must first prove the patient actually has acidosis. Patients with low serum bicarbonate (HCO 3) concentration, or hypobicarbonatemia, are commonly assumed to have metabolic acidosis, but hypobicarbonatemia is also an expected consequence of respiratory alkalosis (Fig. 2.1).In the absence of a simultaneous blood pH measurement a low serum HCO 3 ...
Patients with acute metabolic acidosis demonstrate tachypnea and hyperpnea as prominent physical signs. Kussmaul respiration, an extremely intense respiratory effort, may be present. Hyperventilation, in the absence of obvious lung disease, should alert the clinician to the possibility of an underlying metabolic acidosis.
Metabolic acidosis is a condition in which the body produces too much acid (e.g. acetic acid or ketones), or excessively loses bicarbonate ions (e.g. diarrhea or renal failure ). In metabolic acidosis, the respiratory center is stimulated in order to compensate for the acidosis and the individual hyperventilates, leading to dyspnea.
With metabolic acidosis, "acidosis" refers to a process that lowers blood pH below 7.35, and "metabolic" refers to the fact that it's a problem caused by a decrease in the bicarbonate HCO3− concentration in the blood. Normally, blood pH depends on the balance or ratio between the concentration of bases, mainly bicarbonate HCO3− ...
Long and deep breaths. Fast heartbeat. Headache and/or confusion. Weakness. Feeling very tired. Vomiting and/or feeling sick to your stomach (nausea) Loss of appetite. If you experience any of these, it is important to let your healthcare provider know immediately.
bolic acidosis. A patient with hyperchloremic metabolic acidosis and a urine pH over 5.5 or a positive UAG has RTA until proven otherwise. Several distinct types of RTA exist (Table 2.1). When the urine pH exceeds 5.5 or the UAG is positive in the presence of meta-bolic acidosis the evaluation of RTA continues as shown in Fig. 2.3. Although clas-
Purpose of review Metabolic acidosis has a wide array of etiologies making the initial evaluation imperative in order to identify the proper treatment pathway. The clinical sequela of metabolic acidosis can often be vague and nonspecific. This underscores the importance of being able to comfortably recognize and evaluate acid-base disturbances. Recent findings The diagnostic modalities used to ...
Numerous drugs and toxins can cause metabolic acidosis. The treating clinician should be aware of the many compounds that can produce metabolic acidosis following an accidental exposure, an overdose, or with therapeutic use. Awareness and comprehension of those substances associated with metabolic acidosis will facilitate the diagnosis and treatment of poisoned patients.
Metabolic acidosis. You can identify metabolic acidosis on an ABG using two steps: Check the pH. A pH under 7.35 is acidic, and indicative of acidosis.*. Determine which system, respiratory or metabolic, is to blame for the acidosis. The value for bicarbonate (HCO₃) represents the metabolic system.
Metabolic acidosis is common in chronic kidney disease (CKD) patients and can lead to poor outcomes, such as bone demineralization, muscle mass loss, and worsening of renal function. Metabolic acidosis is usually approached with evaluating the serum bicarbonate levels but should be assessed by counting blood pH.
Unexplained metabolic acidosis. A man in his 20s was found to be unresponsive after experiencing headache and vomiting for two days. His medical history included depression, paracetamol (acetaminophen) overdose on several occasions, and an episode of unexplained encephalopathy five months earlier. He experienced two generalised tonic-clonic ...
Diabetic ketoacidosis (DKA) is an acute complication of diabetes that results from absolute or relative insulin deficiency. 1,2 It is characterized by the presence of metabolic acidosis, ketonemia, and hyperglycemia. 2 With prompt treatment, most patients with DKA recover, but in rare cases, such as patients who develop cerebral edema or have an underlying illness, DKA can become life ...
Patients undergoing cardiac surgery, due to their pre-existing CV disease which often requires SGLT2i prescriptions, face an increased risk of postoperative metabolic acidosis (MA) or ketoacidosis ...
Rationale & Objective. Metabolic acidosis related to chronic kidney disease (CKD) is associated with an accelerated decline in glomerular filtration rate (GFR) and the development of end-stage kidney disease. Whether metabolic acidosis is associated with cardiovascular (CV) events in patients with CKD is unclear.
Grade 3 or higher events that may be associated with CRS include hypotension, hypoxia, hyperbilirubinemia, hypofibrinogenemia, ARDS, atrial fibrillation, hepatocellular injury, metabolic acidosis, pulmonary edema, coagulopathy, renal failure, multiple organ dysfunction syndrome and HLH/MAS. Identify CRS based on clinical presentation.
Abstract. Introduction: Metabolic acidosis is a dangerous and potentially life-threatening condition encountered in the inpatient and emergency department setting. Metabolic acidoses due to renal failure, bicarbonate losses, or lactic acidosis are common conditions, and the appropriate medical management of each is relevant to any inpatient ...