Newly discovered stem cell offers clues to a cancer mystery

Scientists have discovered a new type of stem cell in the spine that appears crucial to resolving a long-standing mystery: why far more cancer cells spread to the spine than to other bones in the body.
When breast, lung and prostate cancers metastasize to multiple bones in the body, three to five times more cancer winds up in the spine than in the lower and upper limbs. Scientists have known of this disparity for decades , but the reason for it has remained unclear.
One theory held that differences in blood flow might be the cause. But the new findings suggest an alternative that could have implications for cancer care, spine fusion surgery and osteoporosis, a bone-weakening disease that afflicts about 10 million Americans.
Stem cells are like the body’s raw materials. They can divide and form more stem cells, or develop to reach a more specific destiny as skin, red blood cells, neurons or any of the estimated 200 different cell types in the human body.
In the journal Nature, researchers from Weill Cornell Medicine and the Hospital for Special Surgery in New York report the discovery of what they have called vertebral skeletal stem cells in the spine. These cells make a protein that acts as a “come here” signal to tumor cells, a finding that raises new treatment possibilities.
“We predict this discovery will lead to the targeting of these cells to disrupt the function and ultimately reduce the spread of cancer to the spine,” said Matthew B. Greenblatt, one of the study’s authors and a pathologist at Weill Cornell Medicine.
In work spanning five years, scientists found the cells first in mice, then in humans. The cells, which are responsible for bone formation in the vertebrae, appear as the bone hardens.
To demonstrate the crucial role of vertebral skeletal stem cells, researchers developed a mouse from which they could snip out some of its DNA. Using an enzyme, they removed a specific bone-forming gene from the newly discovered vertebral stem cells. Mice that had the gene removed displayed clear spinal defects, proving the importance of the new stem cells in forming the spine.
Scientists then transplanted the stem cells into the leg muscle of a mouse. The transplanted cells made new miniature bones from scratch, and produced all of the types of skeletal cells that are found in the spine. Researchers concluded that the vertebral skeletal stem cells help to form the spine before birth, then help maintain it after birth.
To find the same stem cells in humans, researchers studied very small pieces of vertebrae removed during laminectomies, surgeries that relieve pressure on the nerves and spinal cord.
“It’s very rare that you find a new stem cell, and that’s one of the things that makes us excited about this,” Greenblatt said. “We think there are more to discover.”
By comparing the stem cells that form spinal bone with those that form limb bones, they discovered one protein that is made at much higher levels in the vertebrae. Mice that lacked this protein experienced far less cancer spreading to their spines.
Feini Qu, a core faculty member at the University of Washington’s Institute for Stem Cell and Regenerative Medicine, called the study “a breakthrough that helps us understand the developmental origin of the vertebrae.” Qu, who was not involved in the project, added that the research “might help us understand ways to be more creative” in slowing or stopping spinal metastasis.
Sean Morrison, founding director of the Children’s Research Institute at UT Southwestern Medical Center in Dallas, said that he considers the cells described by the researchers to be skeletal stem cells, a type whose existence has been known about for years.
But Morrison, who is also an investigator for Howard Hughes Medical Institute, added that the paper still shows skeletal stem cells found in different parts of the body have somewhat different properties.
“The skeletal stem cells in the vertebrae have a surprising capacity to attract cancer cells that you don’t see in other skeletal stem cells,” he said.
C. Rory Goodwin, a neurosurgeon and spine surgeon at Duke Health, commended the paper’s authors and said the discovery of the stem cells may help researchers improve the poor outlook for cancer patients whose disease has spread to the spine.
“When patients have spine metastasis, that’s usually toward the end of the line,” Goodwin said. Average survival, he said, is between 10 and 14 months once cancer reaches the spine, “with some patients surviving much less than that.”
In addition, the discovery provides an explanation for why osteoporosis varies significantly in the spine vs. other parts of the skeleton. This knowledge could help doctors tailor treatment of the disease when it appears in the spine.
In separate work, Greenblatt and his co-author on the Nature paper, Sravisht Iyer, a spine surgeon at the Hospital for Special Surgery, are investigating the role the new stem cell plays in responding to spine fusion surgery. They want to determine whether an implant of the new stem cell at the time of surgery can improve fusion.
The two scientists also suspect there may be a second type of vertebral skeletal stem cell. When they blocked the ability of the new stem cell to form bone, they still found small amounts of bone in some regions of the spine, raising the question of whether a second stem cell type might be responsible.

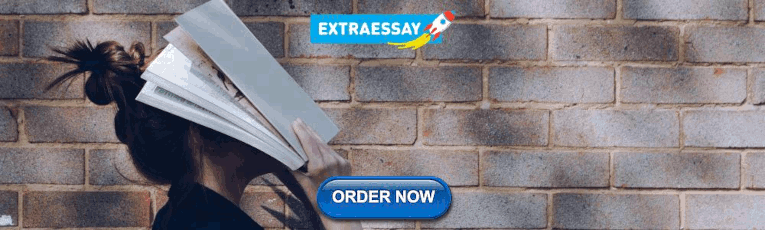
Cancer stem cells: advances in biology and clinical translation-a Keystone Symposia report
Affiliations.
- 1 PhD Science Writer, New York, New York.
- 2 CAS Key Laboratory of Regenerative Biology, Guangdong Provincial Key Laboratory of Stem Cell and Regenerative Medicine, Guangzhou, China.
- 3 Guangzhou Institutes of Biomedicine and Health, Chinese Academy of Sciences, Guangzhou, China.
- 4 Guangzhou Regenerative Medicine and Health Guangdong Laboratory (GRMH-GDL), Guangzhou, China.
- 5 Department of Cell Biology and Physiology, University of North Carolina School of Medicine, Chapel Hill, North Carolina.
- 6 Laboratory of Human Carcinogenesis, and Liver Cancer Program, Center for Cancer Research, National Cancer Institute, National Institutes of Health, Bethesda, Maryland.
- 7 Cold Spring Harbor Laboratory, Cold Spring Harbor, New York.
- 8 Laboratory for Molecular Cancer Biology, Center for Cancer Biology and Laboratory for Molecular Cancer Biology, Department of Oncology, Leuven, Belgium.
- 9 Glioblastoma Translational Center of Excellence, The Abramson Cancer Center and Department of Pathology & Laboratory Medicine, Perelman School of Medicine and Center for Cellular Immunotherapies, University of Pennsylvania, Philadelphia, Pennsylvania.
- 10 Department of Molecular and Medical Genetics, Department of Pediatrics, and Oregon Stem Cell Center, Oregon Health & Science University, Portland, Oregon.
- 11 Department of Cardiovascular and Metabolic Sciences, Lerner Research Institute and Case Comprehensive Cancer Center, Case Western Reserve University School of Medicine, Cleveland Clinic, Cleveland, Ohio.
- 12 Guangdong Provincial Key Laboratory of Malignant Tumor Epigenetics and Gene Regulation, Medical Research Center and Breast Tumor Center, Sun Yat-Sen Memorial Hospital, Sun Yat-Sen University, Guangzhou, China.
- 13 Bioland Laboratory; Program of Molecular Medicine, Zhongshan School of Medicine, Sun Yat-Sen University; and Fountain-Valley Institute for Life Sciences, Guangzhou Institute of Biomedicine and Health, Chinese Academy of Sciences, Guangzhou, China.
- 14 Division of Hematology, Department of Medicine, Stanford University, Stanford, California.
- 15 Translational Cancer Research Center, Peking University First Hospital, Beijing, China.
- 16 Cancer Biology and Genetics, Memorial Sloan Kettering Cancer Center, New York, New York.
- 17 School of Biomedical Sciences and State Key Laboratory of Liver Research, The University of Hong Kong, Hong Kong.
- 18 Laboratory of Human Carcinogenesis, Center for Cancer Research, National Cancer Institute, Bethesda, Maryland.
- 19 Department of Biomedical Sciences, City University of Hong Kong, Hong Kong SAR, China.
- 20 Departments of Medicine, Genetics and Development, Urology, and Systems Biology, Herbert Irving Comprehensive Cancer Center, Columbia University College of Physicians and Surgeons, New York, New York.
- 21 Department of Pathology and State Key Laboratory of Liver Research, The University of Hong Kong, Hong Kong, China.
- 22 Department of Genetics and Institute for Diabetes, Obesity, and Metabolism, Perelman School of Medicine, University of Pennsylvania, Philadelphia, Pennsylvania.
- 23 School of Biomedical Sciences, Li Ka Shing Faculty of Medicine, Hong Kong.
- 24 Institute for the Biology of Stem Cells, University of California, Santa Cruz, Santa Cruz, California.
- 25 Charité - Universitätsmedizin Berlin, Hematology/Oncology, and Max-Delbrück-Center for Molecular Medicine in the Helmholtz Association, Berlin, Germany, and Johannes Kepler University, Kepler Universitätsklinikum, Hematology/Oncology, Linz, Austria.
- 26 Department of Biology, School of Arts & Sciences, University of Pennsylvania, Philadelphia, Pennsylvania.
- 27 MOE Key Laboratory of Biosystems Homeostasis & Protection, and Zhejiang Provincial Key Laboratory for Cancer Molecular Cell Biology, Life Sciences Institute, Zhejiang University, Hangzhou, China.
- 28 Department of Pharmacology & Therapeutics, Roswell Park Comprehensive Cancer Center, and Experimental Therapeutics (ET) Graduate Program, University at Buffalo, Buffalo, New York.
- 29 OncoRay National Center for Radiation Research in Oncology, Faculty of Medicine and University Hospital Carl Gustav Carus, Technische Universität Dresden and Helmholtz-Zentrum Dresden-Rossendorf, Dresden, Germany.
- 30 Helmholtz-Zentrum Dresden-Rossendorf, Institute of Radiooncology-OncoRay, Dresden, Germany.
- 31 German Cancer Consortium (DKTK), Partner Site Dresden, and German Cancer Research Center (DKFZ), Heidelberg, Germany.
- 32 National Center for Tumor Diseases (NCT), Partner Site Dresden, Heidelberg, Germany.
- 33 Neuro-Oncology Branch, National Cancer Institute, Center for Cancer Research, National Institutes of Health, Bethesda, Maryland.
- 34 AbbVie, Inc., Lake Bluff, Illinois.
- 35 Institute for Stem Cell Biology and Regenerative Medicine, Ludwig Center for Cancer Stem Cell Research, Stanford University, Stanford, California.
- PMID: 34850398
- PMCID: PMC9153245
- DOI: 10.1111/nyas.14719
The test for the cancer stem cell (CSC) hypothesis is to find a target expressed on all, and only CSCs in a patient tumor, then eliminate all cells with that target that eliminates the cancer. That test has not yet been achieved, but CSC diagnostics and targets found on CSCs and some other cells have resulted in a few clinically relevant therapies. However, it has become apparent that eliminating the subset of tumor cells characterized by self-renewal properties is essential for long-term tumor control. CSCs are able to regenerate and initiate tumor growth, recapitulating the heterogeneity present in the tumor before treatment. As great progress has been made in identifying and elucidating the biology of CSCs as well as their interactions with the tumor microenvironment, the time seems ripe for novel therapeutic strategies that target CSCs to find clinical applicability. On May 19-21, 2021, researchers in cancer stem cells met virtually for the Keystone eSymposium "Cancer Stem Cells: Advances in Biology and Clinical Translation" to discuss recent advances in the understanding of CSCs as well as clinical efforts to target these populations.
Keywords: cancer stem cell; hepatocellular carcinoma; organoids; pluripotent; progenitors; stemness; tumor heterogeneity; tumorigenesis.
© 2021 New York Academy of Sciences.
Publication types
- Research Support, N.I.H., Extramural
- Research Support, Non-U.S. Gov't
- Biomarkers, Tumor / genetics
- Biomarkers, Tumor / metabolism
- Congresses as Topic / trends*
- Neoplasms / genetics*
- Neoplasms / metabolism
- Neoplastic Stem Cells / physiology*
- Research Report*
- Translational Research, Biomedical / methods
- Translational Research, Biomedical / trends*
- Tumor Microenvironment / physiology*
- Biomarkers, Tumor
Grants and funding
- R35 CA220434/CA/NCI NIH HHS/United States
- Z01 BC010876/ImNIH/Intramural NIH HHS/United States
Find Info For
- Current Students
- Prospective Students
- Research and Partnerships
- Entrepreneurship and Commercialization
Quick Links
- Health and Life Sciences
- Info Security and AI
- Transformative Education
- Purdue Today
- Purdue Global
- Purdue in the News
April 22, 2024
Genetically engineering a treatment for incurable brain tumors
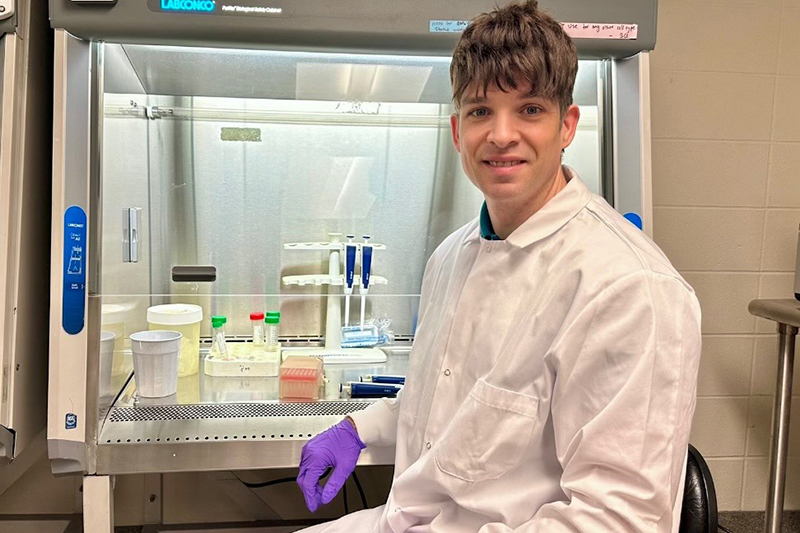
Sandro Matosevic, associate professor in the Department of Industrial and Molecular Pharmaceutics in Purdue’s College of Pharmacy, leads a team of researchers that is developing a novel immunotherapy to be used against glioblastoma. (Purdue University photo/Shambhavi Borde)
Purdue researchers develop fully off-the-shelf, stem cell-derived, natural killer cells against glioblastoma
WEST LAFAYETTE, Ind. — Purdue University researchers are developing and validating a patent-pending treatment for incurable glioblastoma brain tumors. Glioblastomas are almost always lethal with a median survival time of 14 months. Traditional methods used against other cancers, like chemotherapy and immunotherapy, are often ineffective on glioblastoma.
Sandro Matosevic , associate professor in the Department of Industrial and Molecular Pharmaceutics in Purdue’s College of Pharmacy , leads a team of researchers that is developing a novel immunotherapy to be used against glioblastoma. Matosevic is also on the faculty of the Purdue Institute for Cancer Research and the Purdue Institute for Drug Discovery .
The Matosevic-led research has been published in the peer-reviewed journal Nature Communications .
The Purdue glioblastoma treatment
Matosevic said traditional cell therapies have almost exclusively been autologous, meaning taken from and returned to the same patient. Blood cells from a patient are engineered to better recognize and bind to proteins on cancer cells, then given back to the same patient to bind to and attack cancer cells. Unfortunately, these therapies have limited to no effect on glioblastoma.
“By contrast, we are developing immunotherapy based on novel, genetically engineered, fully off-the-shelf or allogeneic immune cells. Allogeneic cells are not sourced from the same patient, but rather another source,” Matosevic said. “In our study, we sourced — or rather engineered — cells from induced pluripotent stem cells. So we eliminated the need for blood and instead differentiated stem cells into immune cells, or natural killer cells, and then genetically engineered those.”
Matosevic said novel Purdue immunotherapy can be considered to have a true off-the-shelf source.
“We can envision having unlimited supplies of these stem cells ready to be engineered,” Matosevic said. “This does not require blood to be sourced. And because these are human cells, they are directly usable in human patients.”
Validation and next development steps
The research team tested its treatment by conducting animal studies with mice bearing human brain tumors, which were treated by direct injection of the newly engineered immune cells.
“Our preclinical studies showed these immune cells to be particularly remarkable in targeting and completely eliminating the growth of the tumors,” Matosevic said. “We found that we can engineer these cells at doses suitable for clinical use in humans. This is significant because one of the major hurdles to clinical translation of cell-based therapies to humans has been the poor expansion and lack of potency of cells that were sourced directly from patients. Using an off-the-shelf, fully synthetic approach breaks down significant barriers to the manufacturing of these cells.”
Matosevic said the next step to develop the glioblastoma treatment is to conduct clinical trials to treat patients with brain tumors, including those that were not successfully eliminated by surgery.
“Our ultimate goal is to bring this therapy to patients with brain tumors,” Matosevic said. “These patients urgently deserve better, and more effective, treatment options. We believe there is true potential for this therapy, and we have the motivation and capacity to bring it to the clinic.
“We are working with neurosurgical clinician collaborators to not only obtain funding, but also initiate clinical protocols,” he added. “We are also open to and always seeking new collaborations and partnerships with those who have interest in supporting our mission to translate this therapy to the clinic, where it is needed the most.”
Matosevic disclosed the innovative glioblastoma treatment to the Purdue Innovates Office of Technology Commercialization , which has applied for a patent from the U.S. Patent and Trademark Office to protect the intellectual property. Inquiries about the status of the intellectual property may be directed to Joe Kasper, assistant director of business development and licensing — life sciences, at [email protected] .
Matosevic and the research team received funding from the National Institutes of Health, the V Foundation for Cancer Research, the Purdue Institute for Cancer Research and industry partners.
About Purdue Innovates Office of Technology Commercialization
The Purdue Innovates Office of Technology Commercialization operates one of the most comprehensive technology transfer programs among leading research universities in the U.S. Services provided by this office support the economic development initiatives of Purdue University and benefit the university’s academic activities through commercializing, licensing and protecting Purdue intellectual property. In fiscal year 2023, the office reported 150 deals finalized with 203 technologies signed, 400 disclosures received and 218 issued U.S. patents. The office is managed by the Purdue Research Foundation, which received the 2019 Innovation & Economic Prosperity Universities Award for Place from the Association of Public and Land-grant Universities. In 2020, IPWatchdog Institute ranked Purdue third nationally in startup creation and in the top 20 for patents. The Purdue Research Foundation is a private, nonprofit foundation created to advance the mission of Purdue University. Contact [email protected] for more information.
About Purdue University
Purdue University is a public research institution demonstrating excellence at scale. Ranked among top 10 public universities and with two colleges in the top four in the United States, Purdue discovers and disseminates knowledge with a quality and at a scale second to none. More than 105,000 students study at Purdue across modalities and locations, including nearly 50,000 in person on the West Lafayette campus. Committed to affordability and accessibility, Purdue’s main campus has frozen tuition 13 years in a row. See how Purdue never stops in the persistent pursuit of the next giant leap — including its first comprehensive urban campus in Indianapolis, the new Mitchell E. Daniels, Jr. School of Business, and Purdue Computes — at https://www.purdue.edu/president/strategic-initiatives .
Writer/Media contact: Steve Martin, [email protected]
Source: Sandro Matosevic, [email protected]
Research Foundation News
Communication.
- OneCampus Portal
- Brightspace
- BoilerConnect
- Faculty and Staff
- Human Resources
- Colleges and Schools
Info for Staff
- Purdue Moves
- Board of Trustees
- University Senate
- Center for Healthy Living
- Information Technology
- Ethics & Compliance
- Campus Disruptions
Purdue University, 610 Purdue Mall, West Lafayette, IN 47907, (765) 494-4600
© 2015-24 Purdue University | An equal access/equal opportunity university | Copyright Complaints | Maintained by Office of Strategic Communications
Trouble with this page? Disability-related accessibility issue? Please contact News Service at [email protected] .
Thank you for visiting nature.com. You are using a browser version with limited support for CSS. To obtain the best experience, we recommend you use a more up to date browser (or turn off compatibility mode in Internet Explorer). In the meantime, to ensure continued support, we are displaying the site without styles and JavaScript.
- View all journals
Stem cells articles from across Nature Portfolio
Stem cells are cells that have the capacity to self-renew by dividing and to develop into more mature, specialised cells. Stem cells can be unipotent, multipotent, pluripotent or totipotent, depending on the number of cell types to which they can give rise.
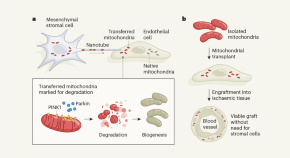
Cells destroy donated mitochondria to build blood vessels
Organelles called mitochondria are transferred to blood-vessel-forming cells by support cells. Unexpectedly, these mitochondria are degraded, kick-starting the production of new ones and boosting vessel formation.
- Chantell S. Evans
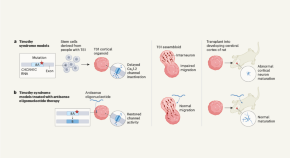
Targeting RNA opens therapeutic avenues for Timothy syndrome
A therapeutic strategy that alters gene expression in a rare and severe neurodevelopmental condition has been tested in stem-cell-based models of the disease, and has been shown to correct genetic and cellular defects.
- Silvia Velasco
Related Subjects
- Adult stem cells
- Cancer stem cells
- Embryonic germ cells
- Embryonic stem cells
- Epigenetic memory
- Haematopoietic stem cells
- Heart stem cells
- Intestinal stem cells
- Mammary stem cells
- Mesenchymal stem cells
- Multipotent stem cells
- Muscle stem cells
- Neural stem cells
- Pluripotent stem cells
- Regeneration
- Reprogramming
- Self-renewal
- Skin stem cells
- Stem-cell differentiation
- Stem-cell niche
- Totipotent stem cells
- Transdifferentiation
Latest Research and Reviews
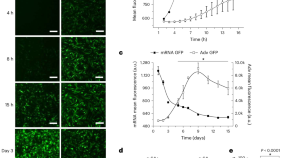
Transient pacing in pigs with complete heart block via myocardial injection of mRNA coding for the T-box transcription factor 18
Intramyocardial injection of synthetic mRNA coding for the embryonic T-box transcription factor 18 gene generates rate-adaptive cardiac pacing and limits innate and inflammatory immune responses, as shown in rodents and pigs.
- David W. Wolfson
- Nam Kyun Kim
- Hee Cheol Cho
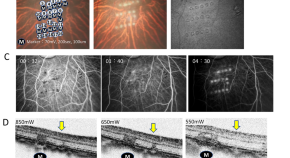
Graft cell expansion from hiPSC-RPE strip after transplantation in primate eyes with or without RPE damage
- Keisuke Kajita
- Mitsuhiro Nishida
- Michiko Mandai
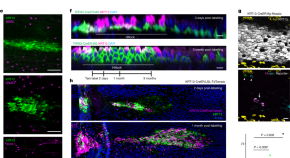
Airway hillocks are injury-resistant reservoirs of unique plastic stem cells
In the lungs, recently identified epithelial structures known as hillocks can act as injury-resistant reservoirs of stem cells.
- Viral S. Shah
- Jayaraj Rajagopal
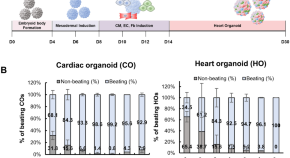
Modeling acute myocardial infarction and cardiac fibrosis using human induced pluripotent stem cell-derived multi-cellular heart organoids
- Myeongjin Song
- Da Bin Choi
- Dong-Hun Woo
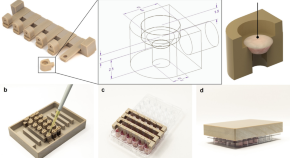
Development of a novel high-throughput culture system for hypoxic 3D hydrogel cell culture
- Dominik Egger
- Luisa Baier
- Cornelia Kasper
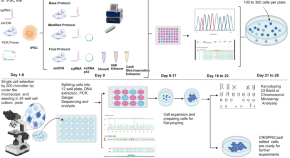
A high efficiency precision genome editing method with CRISPR in iPSCs
- Avinash Singh
- G. Dalton Smedley
- Shauna H. Yuan
News and Comment
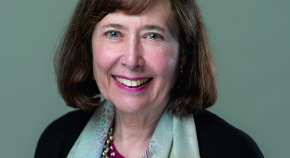
Connie J. Eaves (1944–2024)
- Nagarajan Kannan
- Aniruddha J. Deshpande
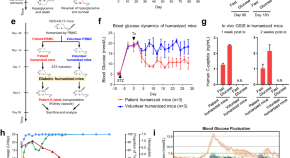
Treating a type 2 diabetic patient with impaired pancreatic islet function by personalized endoderm stem cell-derived islet tissue
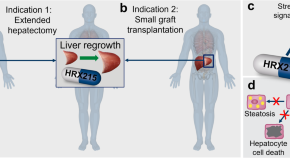
Prometheus 2.0: drug-induced liver regeneration arising
- Jan S. Tchorz
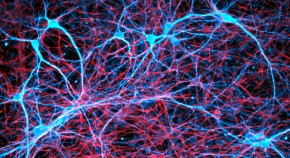
Rat neurons repair mouse brains — and restore sense of smell
Scientists develop hybrid mice by filling in missing cells and structures in their brains with rat stem cells.
- Sara Reardon
Quick links
- Explore articles by subject
- Guide to authors
- Editorial policies

- See us on facebook
- See us on twitter
- See us on youtube
- See us on linkedin
- See us on instagram
Stanford Medicine scientists transform cancer cells into weapons against cancer
Researchers found that when they turned cancer cells into immune cells, they were able to teach other immune cells how to attack cancer.
March 1, 2023 - By Christopher Vaughan
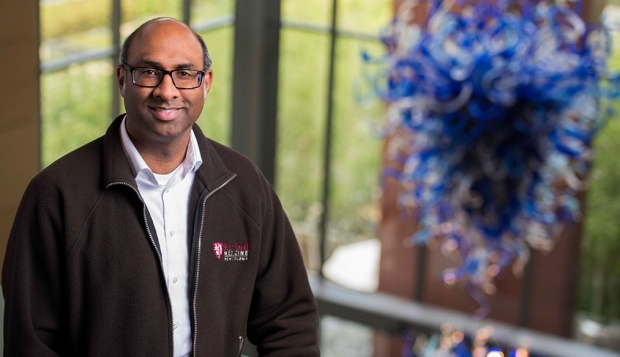
Ravi Majeti and his colleagues programmed mouse leukemia cells so that some of them could be induced to transform themselves into cells that attacked the cancer from which the cells were derived. Steve Fisch
Some cities fight gangs with ex-members who educate kids and starve gangs of new recruits. Stanford Medicine researchers have done something similar with cancer — altering cancer cells so that they teach the body’s immune system to fight the very cancer the cells came from.
“This approach could open up an entirely new therapeutic approach to treating cancer,” said Ravi Majeti , MD, PhD, a professor of hematology and the study’s senior author. The research was published March 1 in Cancer Discovery . The lead author is Miles Linde, PhD, a former PhD student in immunology who is now at the Fred Hutchinson Cancer Institute in Seattle.
Some of the most promising cancer treatments use the patient’s own immune system to attack the cancer, often by taking the brakes off immune responses to cancer or by teaching the immune system to recognize and attack the cancer more vigorously. T cells, part of the immune system that learns to identify and attack new pathogens such as viruses, can be trained to recognize specific cancer antigens, which are proteins that generate an immune response.
For instance, in CAR T-cell therapy, T cells are taken from a patient, programmed to recognize a specific cancer antigen, then returned to the patient. But there are many cancer antigens, and physicians sometimes need to guess which ones will be most potent.
Like an immune response
A better approach would be to train T cells to recognize cancer via processes that more closely mimic the way things naturally occur in the body — like the way a vaccine teaches the immune system to recognize pathogens. T cells learn to recognize pathogens because special antigen presenting cells (APCs) gather pieces of the pathogen and show them to the T cells in a way that tells the T cells, “Here is what the pathogen looks like — go get it.”
Something similar in cancer would be for APCs to gather up the many antigens that characterize a cancer cell. That way, instead of T cells being programmed to attack one or a few antigens, they are trained to recognize many cancer antigens and are more likely to wage a multipronged attack on the cancer.
Now that researchers have become adept at transforming one kind of cell into another, Majeti and his colleagues had a hunch that if they turned cancer cells into a type of APC called macrophages, they would be naturally adept at teaching T cells what to attack.
“We hypothesized that maybe cancer cells reprogrammed into macrophage cells could stimulate T cells because those APCs carry all the antigens of the cancer cells they came from,” said Majeti, who is also the RZ Cao Professor, assistant director of the Institute for Stem Cell Biology and Regenerative Medicine and director of the Ludwig Center for Cancer Stem Cell Research and Medicine .
Cell conversion
The study builds on prior research from the Majeti lab showing that cells taken from patients with a type of acute leukemia could be converted into non-leukemic macrophages with many of the properties of APCs.
In the current study, the researchers programmed mouse leukemia cells so that some of them could be induced to transform themselves into APCs. When they tested their cancer vaccine strategy on the mouse immune system, the mice successfully cleared the cancer.
“When we first saw the data showing clearance of the leukemia in the mice with working immune systems, we were blown away,” Majeti said. “We couldn’t believe it worked as well as it did.”
Other experiments showed that the cells created from cancer cells were indeed acting as antigen-presenting cells that sensitized T cells to the cancer. “What’s more, we showed that the immune system remembered what these cells taught them,” Majeti said. “When we reintroduced cancer to these mice over 100 days after the initial tumor inoculation, they still had a strong immunological response that protected them.”
“We wondered, If this works with leukemias, will it also work with solid tumors?” Majeti said. The team tested the same approach using mouse fibrosarcoma, breast cancer, and bone cancer. “The transformation of cancer cells from solid tumors was not as efficient, but we still observed positive results,” Majeti said. With all three cancers, the creation of tumor-derived APCs led to significantly improved survival.
Lastly, the researchers returned to the original type of acute leukemia. When the human leukemia cell-derived APCs were exposed to human T cells from the same patient, they observed all the signs that would be expected if the APCs were indeed teaching the T cells how to attack the leukemia.
“We showed that reprogrammed tumor cells could lead to a durable and systemic attack on the cancer in mice and a similar response with human patient immune cells,” Majeti said. “In the future we might be able to take out tumor cells, transform them into APCs and give them back to patients as a therapeutic cancer vaccine.”
“Ultimately, we might be able to inject RNA into patients and transform enough cells to activate the immune system against cancer without having to take cells out first,” Majeti said. “That’s science fiction at this point, but that’s the direction we are interested in going.”
The work was supported by funding from the Ludwig Foundation for Cancer Research, the Emerson Collective Cancer Research Fund, the New York Stem Cell Foundation, the Stinehart-Reed Foundation, the Leukemia and Lymphoma Society, the J. Benjamin Eckenhoff Fund, the Blavatnik Family Fellowship, the Deutsche Forschungsgemainshaft, the Knut and Alice Wallenberg Foundation, the Stanford Human Biology Research Exploration Program, the National Institutes of Health (grant F31CA196029), the American Society of Hematology, the A.P. Giannini Foundation, and the Stanford Cancer Institute.

About Stanford Medicine
Stanford Medicine is an integrated academic health system comprising the Stanford School of Medicine and adult and pediatric health care delivery systems. Together, they harness the full potential of biomedicine through collaborative research, education and clinical care for patients. For more information, please visit med.stanford.edu .
Artificial intelligence
Exploring ways AI is applied to health care

- Open access
- Published: 08 February 2023
Shining the light on mesenchymal stem cell-derived exosomes in breast cancer
- Ghaidaa Raheem Lateef Al-Awsi 1 ,
- Fahad Alsaikhan 2 ,
- Ria Margiana 3 , 4 , 5 , 6 ,
- Irfan Ahmad 7 ,
- Indrajit Patra 8 ,
- Mazin A. A. Najm 9 ,
- Ghulam Yasin 10 ,
- Iroda Rasulova 11 ,
- Ali Thaeer Hammid 12 ,
- Hamzah H. Kzar 13 ,
- Moaed E. Al-Gazally 14 &
- Homayoon Siahmansouri 15
Stem Cell Research & Therapy volume 14 , Article number: 21 ( 2023 ) Cite this article
2898 Accesses
6 Citations
1 Altmetric
Metrics details
In women, breast cancer (BC) is the second most frequently diagnosed cancer and the leading cause of cancer death. Mesenchymal stem cells (MSCs) are a subgroup of heterogeneous non-hematopoietic fibroblast-like cells that have the ability to differentiate into multiple cell types. Recent studies stated that MSCs can migrate into the tumor sites and exert various effect on tumor growth and development. Multiple researches have demonstrated that MSCs can favor tumor growth, while other groups have indicated that MSCs inhibit tumor development. Emerging evidences showed exosomes (Exo) as a new mechanism of cell communication which are essential for the crosstalk between MSCs and BC cells. MSC-derived Exo (MSCs-Exo) could mimic the numerous effects on the proliferation, metastasis, and drug response through carrying a wide scale of molecules, such as proteins, lipids, messenger RNAs, and microRNAs to BC cells. Consequently, in the present literature, we summarized the biogenesis and cargo of Exo and reviewed the role of MSCs-Exo in development of BC.
Introduction
Breast cancer (BC) is the second most invasive cancer in the world and the second leading cause of malignancy death among females overall [ 1 , 2 ]. Several factors including genetic and epigenetic mutations, unusual hormone levels, and environmental agents play an important role in BC development [ 3 ]. BC can be classified according to the expression of estrogen receptor-positive, progesterone receptor-positive, and human epidermal growth factor receptor-2-positive [ 4 ]. Also, triple-negative breast cancer (TNBC) is a class of aggressive BC lacking the expression of ER, PR, and HER2 [ 5 ].
Mesenchymal stem cells (MSCs), also mentioned as mesenchymal stromal cells, are non-hematopoietic multipotent cells, chiefly found in the bone marrow (BM) that possess the ability of self-renewal and also display multilineage differentiation [ 6 , 7 , 8 ]. MSCs were identified in variety of adult and fetal/perinatal tissues, such as BM, placenta, Wharton’s jelly, adipose tissue (AD), human umbilical cord (hUC), peripheral blood, and dental pulp [ 9 , 10 , 11 , 12 ]. MSCs can display diverse features according to their origin; however, they must show three minimal principles defined by the International Society for Cellular Therapy [ 13 ]. First, MSCs have plastic-adherence capability when maintained in growth culture media. Second, MSCs must have a particular cell surface antigen expression such as CD73, CD90, CD105, and lacking expression of CD45, CD34, CD14 or CD11b, CD79α or CD19 and HLA-DR. Third, these cells should have the capacity to differentiate into various mesodermal cell types (i.e., adipocytes, chondrocytes, and osteoblasts) when cultured under special conditions. Furthermore, MSCs have ability to differentiate into non-mesodermal cells, such as neuronal cells, cardiomyocytes, hepatocytes or epithelial cells [ 14 , 15 , 16 , 17 ]. This property of stromal cells provides advantages in tissue regeneration. MSCs own a homing ability, which can recruit into the inflammation sites and tissue repair [ 18 , 19 , 20 , 21 ]. Moreover, MSCs have various biological roles such as multilineage differentiation, immunosuppression, and tissue-repair development [ 22 , 23 ]. Because of these benefits, MSCs have been broadly used in clinical studies [ 24 , 25 , 26 , 27 , 28 ], including spinal cord injuries, chronic obstructive pulmonary disease, renal failure, Parkinson’s disease, COVID19, and autoimmune diseases ( https://clinicaltrials.gov/ ).
Multiples studies have been reported that MSCs can also transfer to the tumor stroma and contribute to tumor microenvironment formation [ 29 , 30 , 31 ]. The results of studies have demonstrated that MSCs can change the tumor microenvironment depending on the requirements of the cancer cells for tumor growth directly by releasing growth factors or increasing tumor angiogenesis [ 32 , 33 , 34 ]. On the other hand, some studies indicated that MSCs may contribute in suppressing tumor cells [ 35 , 36 , 37 ]. Nevertheless, the precise mechanisms underlying these opposite effects remain unclear. A large number of studies have been conducted to investigate MSC-derived exosomes (MSCs-Exo) and revealed that MSCs-Exo have mechanisms similar to those of MSCs, such as regeneration tissue injury, repressing inflammatory responses, tumor progress, and stimulating angiogenesis [ 38 , 39 , 40 , 41 , 42 ].
In addition, MSCs-Exo involve in the influences of MSCs on tumor progress. Multiple investigations showing the effects of MSCs-Exo on tumor progress. Therefore, it is reasonable to hypothesize that MSCs-Exo transfer crucial MSCs-related molecules that alter the physiology of target cells in a particular manner. In recent years, MSCs-Exo have shown emerging role in cell-to-cell communication in the promotion of malignancies and cancers.
In this review, first we will describe the exosome’s biogenesis and component. Then we will highlight the effects of MSCs-Exo on breast cancer cell growth and progression.
Extracellular vesicles overview
In addition to the secretion of secretory vesicles by specific cells that facilitates the vesicular transport of cargos, such as hormones or neurotransmitters, most of cells are able to release several types of membrane vesicles, known as extracellular vesicles (EVs) [ 43 ]. At the beginning, it was thought that the release of EVs was a means of eliminating unnecessary compounds from the cell [ 44 ]. Nowadays, we know that EVs are more than just waste transporters, and the chief attention in this field is now focused on their capability to transport cargos between cells, such as DNA to lipids and proteins. EVs are important signaling vehicles in cell homeostatic processes or in pathological progression. Based on their origin, EVs can be mostly divided into two important classes: Exo and microvesicles (MVs) [ 10 , 45 ]. The term Exo was firstly used for membrane vesicles ranging from 30 to 100 nm in diameter secreted by reticulocytes during RBC maturation [ 44 , 46 , 47 ]. Basically, Exo is intraluminal vesicle (ILV) generated via the internal budding of endosome during development of multivesicular bodies (MVBs), which are intermediated into endosomal process, and released upon merging with the plasma membrane (PM) [ 48 , 49 ]. In the 1990s, Exo were stated to be released by immune cells with potential roles associated with immune regulation and were suggested for apply as vehicles in anti-tumoral immune responses [ 50 , 51 ]. The results of studies have demonstrated that various different cell types secrete Exo, and their effects in cell-to-cell communication in wide range of information related to normal and pathological conditions are now well documented [ 52 , 53 ].
In the beginning, MVs termed ‘platelet dust’, were initially stated as subcellular ingredients originating from thrombocytes in plasma and serum of healthy populations [ 54 ]. Subsequently, stimulated neutrophils displayed a process that released the vesicles via fusion with PM, called exocytosis [ 55 ]. In spite of the fact that MVs have been studied mostly for their function in blood coagulation, they participate in intracellular communication in many cell types, such as tumor cells, where they are mainly termed oncosomes [ 56 , 57 ]. MVs are generally larger than Exo (100–1000 nm in diameter) and generated by direct budding of the PM. This process is mediated via an elevation of intracellular cytosolic calcium that triggers calpain. Consequently, this cause restoration of the cytoskeleton, by cleaving the actin protein network and finally budding occur Fig. 1 [ 58 , 59 , 60 , 61 ].
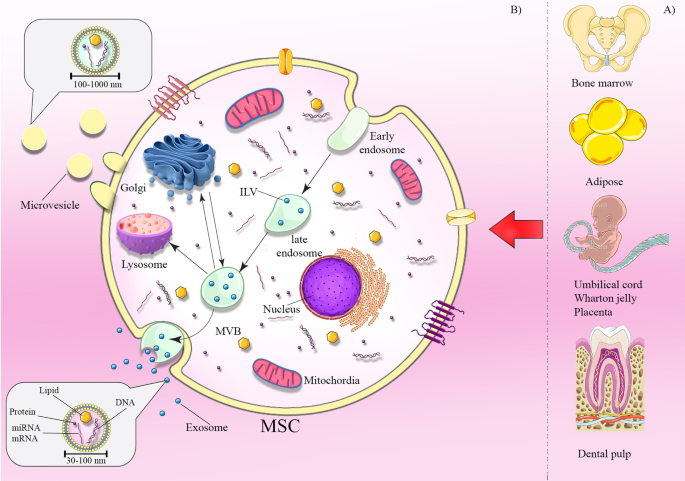
Sources of MSCs and exosome biogenesis. MSCs can be obtained from various sources including bone marrow, adipose, umbilical cord, Wharton jelly, placenta, and dental pulp ( a ). Mechanisms of exosome biogenesis and secretion ( b ). Exosome biogenesis initiates with the process of endocytosis. It includes internal budding of the cell membrane and embraces bioactive molecules, resulting to the formation of the endosome. Furthermore, these molecules are sorted in smaller vesicles which bud from membrane into endosome lumen forming multivesicular bodies (MVBs). The MVBs either fuse with lysosome for degradation or fuse with the plasma membrane to release exosomes
Exosomes biogenesis
Exo generate with internal budding of the PM to produce early endosome, in which early endosomes membrane invaginate and envelope surrounding lumina with cytoplasmic content cause the formation of ILVs within large MVBs [ 62 , 63 ]. Finally, MVBs get transferred to PM through cytoskeletal and microtubule network and fusion with the PM that the ILVs get released as Exo. In addition, MVBs have another fates, they were delivered to lysosomes for degradation of their components, or transported to the trans-Golgi system for endosome recycling [ 64 ]. The mechanisms that regulate the fate of MVBs are not absolutely clear. Two members of the Rab family, Rab27A and B, stimulate MVBs transportation to cell border then SNARE complex induces MVBs fusion with the cell membrane to secrete Exo [ 65 ]. Evidence has shown that endosomal-sorting complex required for transport (ESCRT) plays a central function in ILVs formation [ 66 ]. This complicated machinery composed of multiprotein complexes (ESCRT-0, I, II, and III) with associated proteins (Tsg101, ALIX, and VPS4) that work cooperatively to promote generation of MVB, vesicle formation, and protein cargo sorting [ 67 , 68 ]. During the biogenesis process, each complex has the function, ESCRT-0 recognizes and sequestrates the ubiquitinated cargos to specific domains of the endosomal membrane and trigger the pathway. ESCRT-I and II complexes initiate the deformation of membrane causing buds or stable membrane, the total complex will then merge with ESCRT-III, a subset of ESCRT that is implicate in stimulating the budding processes. Eventually, after cleaving the buds to generate ILVs, Vps4 is recruited to ESCRT-III in order to separate it from the cytoplasmic membrane [ 69 , 70 , 71 ]. Furthermore, exosomal protein Alix, that is related to some ESCRT (TSG101 and CHMP4) proteins, contributes to endosomal membrane budding, as well as exosomal components selection through interaction with syndecan [ 72 ].
Interestingly, last evidence showed an another route for Exo biogenesis and their cargo sorting into MVBs in an ESCRT-independent pathway, which contains lipids and related protein as tetraspanins [ 73 ].
Exosomes components
Exo typically contain luminal components, such as nucleic acids (DNA, RNA), lipid structures, proteins, peptides, and amino acids enriched in a lipid bilayer membrane (Fig. 2 ). Classically, Exo are highly enclosed in proteins with several roles, including tetraspanins (CD9, CD63, CD81, and CD82), which mediate cellular penetration, invasion, and fusion events, heat shock proteins (HSP70, HSP90), which participate in antigen processing and binding; MVB generation proteins that take part in Exo release (Alix, TSG101); as well as proteins responsible for membrane carrying and fusion (annexins and Rab) [ 74 , 75 , 76 ]. Exo also comprise of various forms of RNAs that can be incorporated into target cells. According to the evidences, microRNAs (miRNAs) are the most plentiful in exosomal RNA classes. There are other types of RNAs, such as ribosomal RNA, long non-coding RNA, transfer RNA, messenger RNA (mRNA), and small nuclear RNA [ 77 ]. When miRNAs transferred to Exo, they can experience unidirectional transport between cells, leading to the creation of a cell-to-cell trafficking network, which, in turn, causes transient or constant phenotypic modifications of target cells [ 78 ]. The miRNAs, such as miR-1, miR-320, miR-15, miR-214, miR-29a, miR-16, miR-151, miR-375, and lethal-7, have critical roles in angiogenesis, hematopoiesis, exocytosis, and tumorigenesis [ 79 , 80 , 81 ]. Intriguingly, evidence has been revealed that long RNA types, specifically long non-coding RNAs (lncRNAs) and circular RNAs (circRNAs) are expressed in Exo, and impact a range of biological processes such as tumor progression [ 82 ]. Several studies have been reported that expression of lncRNAs including lncRNA BCRT1, lncRNA HOXD-AS1, lncRNA UCA1, and lncRNA LNMAT2 were considerably increased in Exo derived from tumor cells, which was functionally implicated in promotion cancer cell growth, metastasis, and angiogenesis [ 83 , 84 , 85 , 86 ]. These studies revealed that Exo can mediate transfer of lncRNA as a significant mechanism in tumor progression and play an important role in modification of the tumor microenvironment through influencing main cellular pathways. The biological function of Exo base on not only in their proteins and nucleic acids, but in their lipid composition. Commonly, Exo are rich in phosphatidylserine (PS), phosphatidic acid (PA), cholesterol, sphingomyelin (SM), arachidonic acid and other fatty acids, prostaglandins, and leukotrienes, ensure their stability and rigidity [ 87 ].
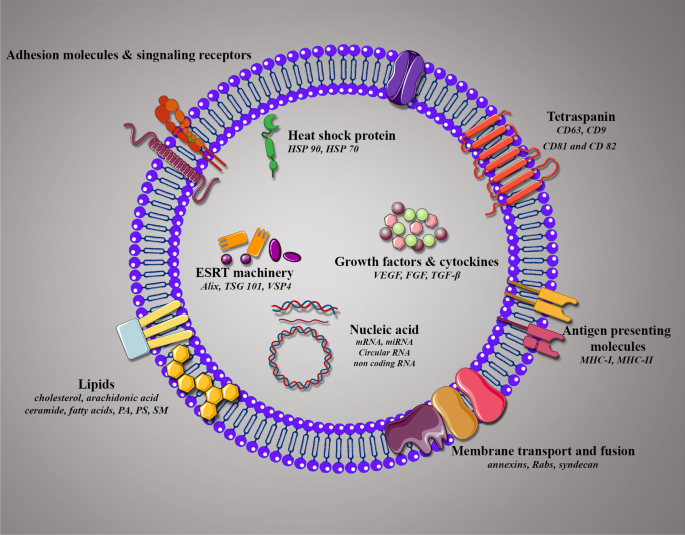
Composition and membrane orientation of exosomes. Schematic illustration of exosome composition containing several families of proteins, lipids, and nucleic acids with membrane orientation in exosomes
The roles of MSCs-Exo in tumors
The tumor microenvironment (TME) is a complicated and heterogeneous network incorporating both endothelial cells and immune cells, such as myeloid-derived suppressor cells (MDSCs), tumor-related stromal cells, cancer-associated fibroblasts (CAFs), and tumor-associated macrophages (TAMs). Generally, the TME is crucial for growth and progression of tumor cells.
The role of MSCs-Exo in development of tumor has been shown. According to the growing evidences, MSCs-Exo transport regulatory cargos to a variety of target cells to change the function of TME, such as tumor cell, CAFs, TAMs, MDSCs, and endothelial cells [ 88 ]. Studies have exhibited that MSCs-Exo can inhibit [ 42 ] and promote [ 89 ] tumor development in recipient cells by transferring components in diverse situations and various stages of cancer. Interestingly, MSCs-Exo obtained from diverse tissues evoke difference effects on cancers. For example, bone marrow MSCs-Exo (BM-MSCs-Exo) stimulate the development of cancer cell by transferring different microRNAs [ 90 , 91 , 92 ]. However, hUCMSC-derived Exo transferred miR-503-3p down-regulated MEST to inhibit human endometrial cancer cells progression [ 93 ], and ADMSC-derived Exo stimulate the differentiation of Th17 and T reg from naive CD4 + T cells to retard proliferation ability of tumor cells via transferring miR-10a [ 94 ]. Furthermore, approximately all researches on TA-MSCs-Exo proposed that they can elevate tumor progression [ 95 ]. The following parts focus on the capacity of MSCs-Exo in the pathogenesis of breast cancer.
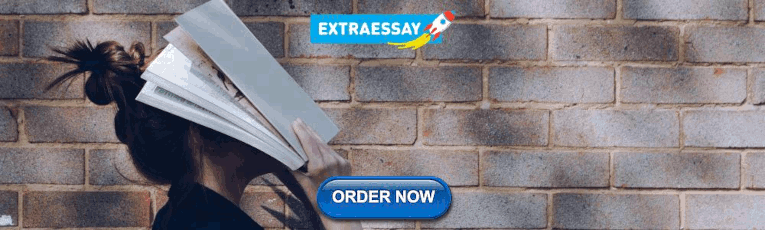
The roles of MSCs-Exo in breast cancer
According to the literature, MSCs-Exo can affect tumor development via various mechanisms (Fig. 3 , Table 1 ).
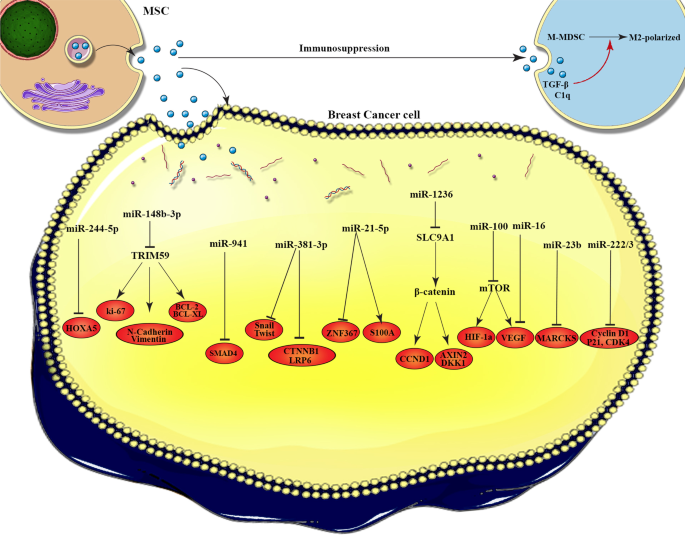
The molecular mechanisms of MSC-EVs cargos in breast cancer progression. This figure illustrates how the exosomes interact with the target cells and the molecular mechanisms by which cargos loaded in exosomes affect breast cancer cells. miR-224-5p increase autophagy in BC cells by down-regulation of HOXA5. miR-148b-3p promotes cell apoptosis via targeting TRIM59. miR-941 suppresses EMT and migration of BC cells by up-regulation of E-cadherin and down-regulation of vimentin, SMAD4, and SNAIL. miR-381 reduces migration, and invasion of BC cells by targeting Wnt signaling pathway and EMT transcription factors. miR-21-5p improves cell viability and drug resistance in the BC cells through induction of S100A6, moreover suppress the metastasis of tumor cell by targeting ZNF367. In addition, miR-1236 increases the sensitivity of BC cells to chemotherapy agent by silencing of SLC9A1 and inactivation of Wnt/β-catenin. Angiogenesis was suppressed in BC cells through miR-100 that modulate the mTOR/HIF-1α axis and miR-16 that down-regulate the expression of VEGF. BC dormancy was promoted by miR-23b that inhibit MARCKS. Quiescence and drug-resistance in tumor cell were induced by miR-222/223 that down-regulate the level of CDK4, Cyclin D1 and p21WAF1
Angiogenesis
Angiogenesis is the generation of new capillaries from pre-existing blood vessels that supplies nutrients for tumor cells, therefore has a crucial role in the BC progression [ 96 ]. Proliferation and metastasis of tumor cells based on a sufficient supply of oxygen and nutrients. Accumulated evidence has shown that MSCs-Exo contain angiogenic stimulatory factors such as fibroblast growth factor (FGF), vascular endothelial growth factor (VEGF), TGFβ1, and interleukin-8 (IL-8), which can promote angiogenesis [ 97 , 98 ]. VEGF acts as a major mediator of angiogenesis in malignancy, it is elevated by hypoxia, wide scale of growth factors, and oncogene expression, and can stimulate mitosis, cell migration, and elevate vascular permeability [ 99 , 100 ]. It has been documented that BC cells co-infused with BMSCs-Exo have higher angiogenesis capability [ 101 ]. However, according to the literature, MSCs-Exo can regulate BC angiogenesis by the targeting effects of some miRNAs on VEGF in BC. Lee and colleagues have reported that BMSCs-Exo contained miR-16 which reduce the expression of VEGF in 4T1 cells and thus suppress angiogenesis and tumor progression in vitro and in vivo [ 102 ]. Additionally, the transcription factor hypoxia-inducible factor-1alpha (HIF-1α) regulates VEGF via binding to the hypoxia response element within the VEGF gene promoter [ 103 ]. Rapamycin (mTOR) plays an important role in regulating cell proliferation and angiogenesis of endothelial cells [ 104 , 105 ], exerts a key function in the HIF-1α-mediated expression of VEGF in BC cells [ 106 ]. Pakravan et al. have been shown that BMSCs-Exo carrying miR-100 down-regulated the expression of VEGF by modulating the mTOR/HIF-1α axis, consequently suppressed angiogenesis in BC cells. In addition, they represented BMSCs-Exo transferring miR-100 decreased VEGF in a dose-dependent and time-dependent [ 107 ]. In summary, although some of studies have confirmed that MSCs-Exo have a promoting effect on BC angiogenesis, most of evidence have suggested their inhibitory effect.
Cell proliferation, apoptosis and autophagy
The role of MSCs-Exo in proliferation of cancer cells has been reported. Various studies have stated that MSCs-Exo can exert different effects on the growth of cancer cells [ 108 ]. Vallabhaneni and coworkers have been shown that BMSCs-Exo increase survival and proliferation of MCF7 cells through carrying tumor supportive proteins, miRNAs, lipids and metabolites [ 101 ]. Similarly, Wang et al. demonstrated that AD-MSCs-Exo promote proliferation and migration of BC cells in vitro and in vivo. They have also found the Hippo signaling pathway was responsible for the tumor progression effects of AD-MSCs-Exo, in which Exo exert their effects through activation of two key downstream effector proteins of Hippo, the YAP and TAZ. Hippo signaling pathway plays a key role in controlling tumorigenesis and regulating BC proliferation and metastasis [ 109 ]. However, their study has a limitation, the in vivo model was carried out using adipocytes treated with GW4869 whereas in vitro studies were done with pure Exo.
Furthermore, Wnt/β-catenin signaling involve in various cancer development, which is marked by nuclear accumulation of β-catenin [ 110 ]. It has been exhibited that AD-MSCs-Exo can enhance the proliferation of MCF7 cells, and up-regulate the expression of β-catenin and Wnt target genes, such as Axin2 and Dkk1 [ 111 ]. These observations suggested that AD-MSCs-Exo play a main role in BC cell proliferation via activation of Wnt signaling pathway. Recently, several studies have been indicated that HAND2-AS1 have an inhibitory effect on BC cell proliferation [ 112 , 113 ]. In a study conducted by Li. Xing et al. [ 114 ], in TUBC, the releasing of BMSCs-Exo carrying miR-106a-5p was inhibited by lncRNA HAND2-AS1. They also reported that HAND2-AS1 can perform as a sponge of miR-106a-5p, and HAND2-AS1 was reduced in TNBC cells. Moreover, up-regulation of HAND2-AS1 suppressed the secretion of BMSCs-Exo comprising miR-106a-5p, thereby inhibited TNBC growth both in vitro and in vivo [ 114 ]. Thus, we hypothesize that BMSCs-Exo carrying miR-106a-5p may support TNBC progression. However, a recent study by Yuan et al. demonstrated that exosomal miR-148b-3p from hUCMSCs suppressed proliferation of MDA-MB-231 cell by down-regulating of TRIM59 [ 115 ]. Likewise, dental pulp-derived MSCs-Exo with overexpressed miR-34a exerted a suppressive effect on BC cell proliferation [ 116 ]. A study conducted by Sheykhhasan et al. [ 117 ] has reported that AD-MSCs-Exo containing miR-145 can suppress the proliferation of BC cells through modulating ROCK1, MMP9, ERBB2, and TP53 gene expression [ 117 ].
Apoptosis is a process of programmed cell death that occurs to maintain physiologic tissue homeostasis. Apoptosis is activated when a cell is no longer needed or has sustained severe injury [ 118 , 119 ]. Autophagy is a self-degradative mechanism, in which cytoplasmic proteins or organelles encapsulated in double-membrane vesicles and fused with lysosomes for degradation or renewal [ 120 ]. Accumulating evidence exhibited that autophagy plays a key role in the removal of injured or unwanted proteins and cellular organelles, therefore preventing apoptosis and improving survival [ 121 ]. It is thought that autophagy inhibits tumor progression. Contrarily, when the tumor is established, autophagy can promote the tumor cell survival and growth [ 122 ]. An in vivo study conducted by Wang et al. [ 123 ] has been demonstrated that hUCMSCs-Exo carrying miR-224-5p can increase autophagy in BC cells through down-regulation of HOXA5, thereby elevate the proliferation and suppress apoptosis of BC cells. Nevertheless, MSCs-Exo can also exhibit an anti-tumor activity through suppression of apoptosis. Yuan et al. have been represented that hUCMSC-Exo carrying miR-148b-3p exhibited the ability to promote the MDA-MB-231 cell apoptosis via targeting tripartite motif 59 (TRIM59) [ 115 ]. The results of studies showed that TRIM59 regulates BC cell apoptosis [ 124 ].
In sum, MSCs-Exo can abnormally activate the signaling pathways or carry tumor supporters to increase proliferation of BC cells. However, they can transport tumor suppressors to exert an inhibitory effect on BC cell proliferation. In addition, MSCs-Exo exerted dual effects on autophagy and apoptosis of BC cells that is needed to be further investigate.
BC metastasis is one of the chief reasons for the high mortality rate of BC. Diagnosis of BC metastasis in the early stage is crucial for the administration and prediction of BC progression. Metastasis is a process that allows cancer cells to circulate in the bloodstream and lymphatic vessels, and then spread to distant parts of the body for colonization [ 125 , 126 ]. Recent evidence suggested that epithelial-to-mesenchymal transition (EMT) could increase metastasis of neighboring tumor cells [ 127 ]. The results of studies indicated that a complex set of signaling pathways regulate the metastasis [ 5 ]. In support of this view, some studies have showed that AD-MSCs-Exo stimulated BC cells migration through the activation of the Wnt signaling pathway [ 111 ]. Besides, Exo can promote metastasis through the regulation of other factors. According to the literature, MSC-exosomal-miR-106a-5p elevated both invasion and migration potential of TNBC cells, nonetheless lncRNA HAND2-AS1 can suppress this effect [ 114 ].
On the other hand, MSCs-Exo also have the capacity to inhibit the migration and metastasis of BC cells. For example, metastasis gene mucin 1 (MUC1), as a marker of tumorigenesis, overexpressed in BC cells and is related to metastasis. Meanwhile, it can be suppressed as a target of AD-MSCs-Exo containing miR-145 to inhibit the metastasis of T-47D cells [ 117 ]. Similarly, it has been revealed that hUCMSCs-Exo-carrying miR-148b-3p inhibit EMT and migration of MDA-MB-231 cells by downregulating the expression of TRIM59 both in vivo and in vitro [ 115 ]. In another study, it was found out that husMSC-Exo suppressed the metastasis of MCF-7 cells through delivering miR-21-5p and suppressing ZNF367 expression [ 128 ]. One study revealed that miR-381 loaded AD-MSC-Exo reduces migration, and invasion of TNBC cells by targeting Wnt signaling pathway (down-regulation of LRP6 and CTNNB1) and EMT transcription factors (Twist and Snail) [ 129 ]. Considerably, BMSCs-Exo with overexpressed miR-let-7f can reduce the proliferation and migration of 4T1 BC cells [ 130 ].
In conclusion, MSCs-Exo exert the dual role in BC cell migration and metastasis by regulation of signaling pathways such as wnt/ β-catenin, and miR‑21‑5p/ZNF367 pathway and delivering their cargos such as miRNAs to BC cells.
Tumor dormancy is a process that cells experience an arrest of cell cycle, termed quiescence. Dormancy is vital for tumor cells to obtain further mutations, to survive in a new environment, initiate metastasis, and to escape the immune system [ 131 ]. There are two types of tumor dormancy; cellular is characterized by three properties: (i) minimum proliferation; (ii) minimum death; and (iii) reversibility that referring to a reversible, non-proliferative, but viable cell status. Another type is tumor mass dormancy that is a situation in which there is a balance between the increase in tumor cell and the decrease in cell death [ 132 , 133 ]. Casson et al. have been reported that BM-MSCs-Exo initiate an epithelial cell phenotype with decrease proliferation and increase adhesion of MCF7 cells, that suggested dormancy of BC cells [ 134 ]. The drawback of this study was that the isolated exosomes contain larger microvesicles that is need to assess the effect of pure MSCs-Exo on BC dormancy. Another study revealed that BM-MSCs-Exo induce BC cell dormancy as cancer stem cells (CSCs). Once BC cells migrate to BM niche and encounter by MSCs, the cargo of BM-MSCs-Exo, alter the behavior of BC cells as CSCs, directly and indirectly by Exo secretion through Wnt/β-catenin pathway [ 135 ]. In addition, dormant BC cells can stimulate more cancer cells to enter a dormant state through stimulating MSCs to secret Exo-containing miRNAs. The results of investigations illuminated that miR-222/223 were up-regulated in Exo from BC-primed MSCs, and down-regulated the level of CDK4, Cyclin D1 and p21WAF1 to induce quiescence and drug-resistance within tumor cells [ 136 ]. In another study, it was exhibited that AD-MSCs-Exo carrying miR-941 significantly up-regulated the expression of E-cadherin and down-regulated vimentin, SMAD4, and SNAIL to suppress EMT and migration of BC cells following co-culture with MCF7-luminal and MDA-basal cells subtypes, which arrest the cell cycle into dormancy [ 137 ]. Furthermore, Ono et al. [ 138 ] reported that some miRNAs in BM-MSCs-Exo were enhanced following co-culture with BM-metastatic human BC cell line (BM2). The overexpression of miR-23b promoted BC dormancy by targeting MARCKS that encodes a protein that increase cell cycling and migration. Thus, we postulated that exosomal miR-23b and its inhibition of MARCKS is one of the ways participating in cell inhibition and dormancy in BCSCs. However, they reported that while Exo-treated BM2 cells showed significantly reduced tumor proliferation, BM2 cell overexpressing miR-23b did not show the same grade of growth suppression, recommending that other factors also participate in this effect. According to all studies above, MSCs-Exo own a promoting role in dormancy of BC.
Chemotherapy resistance
Chemotherapy is one of the main treatment approaches for cancer that have spread from the primary tumor site. Chemotherapy resistance is an important hindrance to achieve therapies in patients and is the crucial cause of death in most progressive stage cancers [ 139 , 140 ]. Drug resistance caused by several factors, including genetic mutations and/or epigenetic changes, and other cellular and molecular mechanisms [ 141 ]. Growing evidence has demonstrated that MSCs contribute to resistance of cancer cells to wide range of anti-cancer drugs [ 142 ]. Some studies have reported that hUCMSCs-Exo can participate in drug resistance of cancer via activation of various signaling cascade, the increase of multi-drug resistance-related proteins, and the inhibition of chemotherapy-induced apoptosis [ 143 ]. Several studies suggested that MSCs-Exo can affect chemotherapy agents in BC. Studies conducted in the last part of the last decade indicated that BM-MSCs-Exo promote resistance of BC cells to doxorubicin and the Exo released by doxorubicin-treated MSCs can give rise to a higher resistance of BC cells to doxorubicin than BM-MSCs-Exo. They also have found that doxorubicin treatment enhanced the expression of miR-21-5p in MSCs-Exo, causing the induction of S100A6 in the BC cells both in vitro and in vivo, and improving cell viability and drug resistance [ 144 ]. On the other hand, in a study conducted by Jia et al. AD-MSCs-Exo have shown that transferring miR-1236 increased the sensitivity of BC cells to cisplatin through down-regulation of SLC9A1 and inactivation of Wnt/β-catenin [ 145 ].
Briefly, these observations propose that MSCs-Exo play a contradictory approach in mediating chemoresistance in BC. More investigation is required to find out the role of MSCs-Exo in BC chemoresistance.
Immune evasion
Cancer immune surveillance is a key mechanism that immune system regulates the evolution and progression of tumors. It is characterized by three essential stages: elimination, equilibrium and escape [ 146 ]. Most tumor cells are recognized and eradicated by immune system before clinical presentation. Initially, in elimination phase, innate and adaptive immune responses, such as release of proinflammatory cytokines and infiltrating immune cells lead to antitumor immunity. In second phase, pro- and antitumor immunity fail to entirely eliminate cancer cells, but keep them under control. In the last phase, tumor cells absolutely escape from immune surveillance [ 147 , 148 ]. The studies revealed that BC cells can escape from immune system by regulation of gene expression likewise SOX4 [ 149 ]. Exo play a key role in immune regulation in BC by cargo expressed on their surface or transferred in the lumen [ 150 ]. Biswas et al. [ 151 ] have been unveiled that Exo released by tumor-educated MSCs can enhance development of BC through stimulating differentiation of MDSCs into highly immunosuppressive M2-polarized macrophages at tumor beds. Mechanistically, MSCs-Exo encapsulated in TGF-b, C1q, and semaphorins can stimulate myeloid tolerogenic activity not only through driving the overexpression of PD-L1 in immature myelomonocytic precursors and committed CD206 + macrophage but also through prompting differentiation of MHC class II + macrophages with increased L-Arginase activity and IL-10 production at tumor beds. The results suggested that both mechanisms can increase the BC progression. In brief, MSCs-Exo act as an inhibitory mediators of immune cells in BC.
In recent years, various studies have been revealed that MSCs play a vital role in tumor progression and tumor therapy. Exo are developing as important vehicles in the correlation between MSCs and cancer cells. MSCs-Exo has attracted the attention in therapeutic methods because of their relative abundance of sources and ease of isolation. In addition, other composition of Exo such as lipid and protein that increase Exo stability are other properties that make Exo as perfect transporters. Accumulating evidence has been indicated that MSCs-Exo can exert the promotive and inhibitory effects at different phases of BC development and their application as drug delivery vehicles in cancer therapy. For the first time, we reviewed the dual function of MSCs-Exo in cell proliferation, migration, metastasis, immune evasion, angiogenesis, chemotherapy, and dormancy of BC, thus suggest a more effective strategy for BC therapy. Nevertheless, the study of MSCs-Exo is facing with many questions that is needed to be addressed. For instance, MSCs-Exo from different sources may carry different contents, thereby affecting their effect on BC cells. HUC-MSCs-Exo can suppress development of BC, while AD-MSCs-Exo can induce BC progression. In addition, AD-MSCs-Exo enhanced the migration of MCF7 cells, while inhibited the proliferation of MDA-MB-231 cells, proposing that MSCs-Exo can play a contradictory role in different types of BC. Entirely, according to studies that we mentioned in this review, we can suppose that MSCs-Exo are multifaceted players of BC development and are affected through various factors.
Availability of data and materials
Not applicable.
Abbreviations
Adipose tissue
- Breast cancer
Bone marrow
Cancer-associated fibroblasts
Circular RNAs
Cancer stem cells
Epithelial-to-mesenchymal transition
- Extracellular vesicles
Endosomal-sorting complex required for transport
Fibroblast growth factor
Human umbilical cord
Hypoxia-inducible factor-1alpha
Intraluminal vesicle
Interleukin-8
Long non-coding RNAs
Mesenchymal stem cells
Metastasis gene mucin 1
Microvesicles
Multivesicular bodies
Messenger RNA
Mesenchymal stem cell-derived exosomes
Myeloid-derived suppressor cells
Plasma membrane
Triple-negative breast cancer
Tumor microenvironment
Tumor-associated macrophages
Targeting tripartite motif 59
Vascular endothelial growth factor
Gordon N, et al. Gene expression signatures of breast cancer stem and progenitor cells do not exhibit features of Warburg metabolism. Stem Cell Res Ther. 2015;6(1):157.
Article Google Scholar
Koellensperger E, et al. The impact of human adipose tissue-derived stem cells on breast cancer cells: implications for cell-assisted lipotransfers in breast reconstruction. Stem Cell Res Ther. 2017;8(1):121.
Marcotte R, et al. Functional genomic landscape of human breast cancer drivers, vulnerabilities, and resistance. Cell. 2016;164(1–2):293–309.
Article CAS Google Scholar
Fragomeni SM, Sciallis A, Jeruss JS. Molecular subtypes and local-regional control of breast cancer. Surg Oncol Clin N Am. 2018;27(1):95–120.
Song K, Farzaneh M. Signaling pathways governing breast cancer stem cells behavior. Stem Cell Res Ther. 2021;12(1):245.
Jiang Y, et al. Pluripotency of mesenchymal stem cells derived from adult marrow. Nature. 2002;418(6893):41–9.
Ding DC, Shyu WC, Lin SZ. Mesenchymal stem cells. Cell Transplant. 2011;20(1):5–14.
Zakrzewski W, et al. Stem cells: past, present, and future. Stem Cell Res Ther. 2019;10(1):68.
Meirelles LDS, Chagastelles PC, Nardi NB. Mesenchymal stem cells reside in virtually all post-natal organs and tissues. J Cell Sci. 2006;119(11):2204–13.
Ghorbani F, et al. Renoprotective effects of extracellular vesicles: a systematic review. Gene Reports. 2022;26: 101491.
Abbaszadeh H, et al. Regenerative potential of Wharton’s jelly-derived mesenchymal stem cells: a new horizon of stem cell therapy. J Cell Physiol. 2020;235(12):9230–40.
Tang Y, Zhou Y, Li H-J. Advances in mesenchymal stem cell exosomes: a review. Stem Cell Res Ther. 2021;12(1):71.
Dominici M, et al. Minimal criteria for defining multipotent mesenchymal stromal cells. Int Soc Cell Therapy Position Statement. 2006;8(4):315–7.
CAS Google Scholar
Lee KD, et al. In vitro hepatic differentiation of human mesenchymal stem cells. Hepatology. 2004;40(6):1275–84.
Păunescu V, et al. In vitro differentiation of human mesenchymal stem cells to epithelial lineage. J Cell Mol Med. 2007;11(3):502–8.
Pedersen TO, et al. Mesenchymal stem cells induce endothelial cell quiescence and promote capillary formation. Stem Cell Res Ther. 2014;5(1):23.
Abbaszadeh H, et al. Chronic obstructive pulmonary disease and asthma: mesenchymal stem cells and their extracellular vesicles as potential therapeutic tools. Stem Cell Res Ther. 2022;13(1):262.
Huang J, et al. Intrauterine infusion of clinically graded human umbilical cord-derived mesenchymal stem cells for the treatment of poor healing after uterine injury: a phase I clinical trial. Stem Cell Res Ther. 2022;13(1):85.
Ji JF, et al. Interactions of chemokines and chemokine receptors mediate the migration of mesenchymal stem cells to the impaired site in the brain after hypoglossal nerve injury. Stem Cells. 2004;22(3):415–27.
Pan L, et al. Human Wharton’s jelly-derived mesenchymal stem cells alleviate concanavalin A-induced fulminant hepatitis by repressing NF-κB signaling and glycolysis. Stem Cell Res Ther. 2021;12(1):496.
Arno AI, et al. Human Wharton’s jelly mesenchymal stem cells promote skin wound healing through paracrine signaling. Stem Cell Res Ther. 2014;5(1):28.
Jiang XX, et al. Human mesenchymal stem cells inhibit differentiation and function of monocyte-derived dendritic cells. Blood. 2005;105(10):4120–6.
Marinescu C-I, Preda MB, Burlacu A. A procedure for in vitro evaluation of the immunosuppressive effect of mouse mesenchymal stem cells on activated T cell proliferation. Stem Cell Res Ther. 2021;12(1):319.
Stessuk T, Ribeiro-Paes JT. Comment on “Allogeneic umbilical cord-derived mesenchymal stem cell transplantation for treating chronic obstructive pulmonary disease: a pilot clinical study.” Stem Cell Res Ther. 2020;11(1):340.
Eirin A, Lerman LO. Mesenchymal stem cell treatment for chronic renal failure. Stem Cell Res Ther. 2014;5(4):83.
Wang F, et al. Intravenous administration of mesenchymal stem cells exerts therapeutic effects on parkinsonian model of rats: focusing on neuroprotective effects of stromal cell-derived factor-1alpha. BMC Neurosci. 2010;11:52.
Saleh M, et al. Cell therapy in patients with COVID-19 using Wharton’s jelly mesenchymal stem cells: a phase 1 clinical trial. Stem Cell Res Ther. 2021;12(1):410.
Liang J, et al. Safety analysis in patients with autoimmune disease receiving allogeneic mesenchymal stem cells infusion: a long-term retrospective study. Stem Cell Res Ther. 2018;9(1):312.
Kidd S, et al. Direct evidence of mesenchymal stem cell tropism for tumor and wounding microenvironments using in vivo bioluminescent imaging. Stem Cells. 2009;27(10):2614–23.
Kidd S, et al. Origins of the tumor microenvironment: quantitative assessment of adipose-derived and bone marrow-derived stroma. PLoS ONE. 2012;7(2): e30563.
Roorda BD, et al. Bone marrow-derived cells and tumor growth: contribution of bone marrow-derived cells to tumor micro-environments with special focus on mesenchymal stem cells. Crit Rev Oncol Hematol. 2009;69(3):187–98.
Zhang T, et al. Bone marrow-derived mesenchymal stem cells promote growth and angiogenesis of breast and prostate tumors. Stem Cell Res Ther. 2013;4(3):70.
Djouad F, et al. Immunosuppressive effect of mesenchymal stem cells favors tumor growth in allogeneic animals. Blood. 2003;102(10):3837–44.
Zhu W, et al. Mesenchymal stem cells derived from bone marrow favor tumor cell growth in vivo. Exp Mol Pathol. 2006;80(3):267–74.
Pacioni S, et al. Human mesenchymal stromal cells inhibit tumor growth in orthotopic glioblastoma xenografts. Stem Cell Res Ther. 2017;8(1):53.
Atsuta I, et al. Mesenchymal stem cells inhibit multiple myeloma cells via the Fas/Fas ligand pathway. Stem Cell Res Ther. 2013;4(5):111.
Qiao L, et al. Suppression of tumorigenesis by human mesenchymal stem cells in a hepatoma model. Cell Res. 2008;18(4):500–7.
He L, et al. Bone marrow mesenchymal stem cell-derived exosomes protect cartilage damage and relieve knee osteoarthritis pain in a rat model of osteoarthritis. Stem Cell Res Ther. 2020;11(1):276.
Zhang Y, et al. Exosomes derived from human umbilical cord blood mesenchymal stem cells stimulate regenerative wound healing via transforming growth factor-β receptor inhibition. Stem Cell Res Ther. 2021;12(1):1–14.
Harrell CR, et al. Mesenchymal stem cell-derived exosomes and other extracellular vesicles as new remedies in the therapy of inflammatory diseases. Cells. 2019;8(12):1605.
Jia Y, et al. Mesenchymal stem cells-derived exosomal microRNA-139-5p restrains tumorigenesis in bladder cancer by targeting PRC1. Oncogene. 2021;40(2):246–61.
Li T, et al. Mesenchymal stem cell-derived exosomal microRNA-3940-5p inhibits colorectal cancer metastasis by targeting integrin α6. Dig Dis Sci. 2021;66(6):1916–27.
Schorey JS, et al. Exosomes and other extracellular vesicles in host-pathogen interactions. EMBO Rep. 2015;16(1):24–43.
Johnstone RM, et al. Vesicle formation during reticulocyte maturation. Association of plasma membrane activities with released vesicles (exosomes). J Biol Chem. 1987;262(19):9412–20.
Abbaszadeh H, et al. Human umbilical cord mesenchymal stem cell-derived extracellular vesicles: a novel therapeutic paradigm. J Cell Physiol. 2020;235(2):706–17.
Harding C, Heuser J, Stahl P. Receptor-mediated endocytosis of transferrin and recycling of the transferrin receptor in rat reticulocytes. J Cell Biol. 1983;97(2):329–39.
Pan B-T, Johnstone RMJC. Fate of the transferrin receptor during maturation of sheep reticulocytes in vitro: selective externalization of the receptor. Cell. 1983;33(3):967–78.
Harding C, Heuser J, Stahl P. Endocytosis and intracellular processing of transferrin and colloidal gold-transferrin in rat reticulocytes: demonstration of a pathway for receptor shedding. Eur J Cell Biol. 1984;35(2):256–63.
Pan B-T, et al. Electron microscopic evidence for externalization of the transferrin receptor in vesicular form in sheep reticulocytes. J Cell Biol. 1985;101(3):942–8.
Raposo G, et al. B lymphocytes secrete antigen-presenting vesicles. J Exp Med. 1996;183(3):1161–72.
Zitvogel L, et al. Eradication of established murine tumors using a novel cell-free vaccine: dendritic cell derived exosomes. Nat Med. 1998;4(5):594–600.
Gurung S, et al. The exosome journey: from biogenesis to uptake and intracellular signalling. Cell Commun Signal. 2021;19(1):47.
Colombo M, et al. Biogenesis, secretion, and intercellular interactions of exosomes and other extracellular vesicles. Annu Rev Cell Dev Biol. 2014;30:255–89.
Yáñez-Mó M, et al. Biological properties of extracellular vesicles and their physiological functions. J Extracell Vesicles. 2015;4(1):27066.
Stein JM, Luzio JP. Ectocytosis caused by sublytic autologous complement attack on human neutrophils. The sorting of endogenous plasma-membrane proteins and lipids into shed vesicles. Biochem J. 1991;274(2):381–6.
Sims PJ, et al. Complement proteins C5b-9 cause release of membrane vesicles from the platelet surface that are enriched in the membrane receptor for coagulation factor Va and express prothrombinase activity. J Biol Chem. 1988;263(34):18205–12.
Satta N, et al. Monocyte vesiculation is a possible mechanism for dissemination of membrane-associated procoagulant activities and adhesion molecules after stimulation by lipopolysaccharide. J Immunol. 1994;153(7):3245–55.
Pasquet JM, Dachary-Prigent J, Nurden AT. Calcium influx is a determining factor of calpain activation and microparticle formation in platelets. Eur J Biochem. 1996;239(3):647–54.
Ståhl A-L, et al. Exosomes and microvesicles in normal physiology, pathophysiology, and renal diseases. Pediatr Nephrol. 2019;34(1):11–30.
Abbaszadeh H, et al. The effect of Acellularized Wharton’s Jelly-derived exosomes on myeloid differentiation of umbilical cord blood-derived CD34+ hematopoietic stem cells. Gene Rep. 2021;25: 101298.
Tricarico C, Clancy J, D’Souza-Schorey C. Biology and biogenesis of shed microvesicles. Small GTPases. 2017;8(4):220–32.
McAndrews KM, Kalluri R. Mechanisms associated with biogenesis of exosomes in cancer. Mol Cancer. 2019;18(1):1–11.
Minciacchi VR, Freeman MR, Di Vizio D. Extracellular vesicles in cancer: exosomes, microvesicles and the emerging role of large oncosomes. Semin Cell Dev Biol. 2015;40:41–51.
Williams RL, Urbé S. The emerging shape of the ESCRT machinery. Nat Rev Mol Cell Biol. 2007;8(5):355–68.
Mashouri L, et al. Exosomes: composition, biogenesis, and mechanisms in cancer metastasis and drug resistance. Mol Cancer. 2019;18(1):1–14.
Kalluri R, LeBleu VS. The biology, function, and biomedical applications of exosomes. Science. 2020;367(6478):eaau6977.
Hurley JH. ESCRTs are everywhere. EMBO J. 2015;34(19):2398–407.
Henne WM, Buchkovich NJ, Emr SD. The ESCRT pathway. Dev Cell. 2011;21(1):77–91.
Juan T, Fürthauer M. Biogenesis and function of ESCRT-dependent extracellular vesicles. Semin Cell Dev Biol. 2018;74:66–77.
Juan T, Fürthauer M. Biogenesis and function of ESCRT-dependent extracellular vesicles. In: Seminars in cell & developmental biology. Elsevier. 2018.
Vild CJ, et al. A novel mechanism of regulating the ATPase VPS4 by its cofactor LIP5 and the endosomal sorting complex required for transport (ESCRT)-III protein CHMP5. J Biol Chem. 2015;290(11):7291–303.
Villarroya-Beltri C, et al. Sorting it out: regulation of exosome loading. Semin Cancer Biol. 2014;28:3–13.
Babst M. MVB vesicle formation: ESCRT-dependent, ESCRT-independent and everything in between. Curr Opin Cell Biol. 2011;23(4):452–7.
Vlassov AV, et al. Exosomes: current knowledge of their composition, biological functions, and diagnostic and therapeutic potentials. Biochim Biophys Acta. 2012;1820(7):940–8.
Kalra H, Drummen GP, Mathivanan S. Focus on extracellular vesicles: introducing the next small big thing. Int J Mol Sci. 2016;17(2):170.
Xu M-Y, et al. Differences in the cargos and functions of exosomes derived from six cardiac cell types: a systematic review. Stem Cell Res Ther. 2019;10(1):194.
Huang X, et al. Characterization of human plasma-derived exosomal RNAs by deep sequencing. BMC Genom. 2013;14(1):319.
Mittelbrunn M, et al. Unidirectional transfer of microRNA-loaded exosomes from T cells to antigen-presenting cells. Nat Commun. 2011;2(1):282.
Valadi H, et al. Exosome-mediated transfer of mRNAs and microRNAs is a novel mechanism of genetic exchange between cells. Nat Cell Biol. 2007;9(6):654–9.
Ohyashiki JH, Umezu T, Ohyashiki K. Exosomes promote bone marrow angiogenesis in hematologic neoplasia: the role of hypoxia. Curr Opin Hematol. 2016;23(3):268–73.
Waldenström A, Ronquist G. Role of exosomes in myocardial remodeling. Circ Res. 2014;114(2):315–24.
Hewson C, et al. Extracellular vesicle associated long non-coding RNAs functionally enhance cell viability. Noncoding RNA Res. 2016;1(1):3–11.
Chen C, et al. Exosomal long noncoding RNA LNMAT2 promotes lymphatic metastasis in bladder cancer. J Clin Investig. 2020;130(1):404–21.
Guo Z, et al. Hypoxic tumor-derived exosomal long noncoding RNA UCA1 promotes angiogenesis via miR-96-5p/AMOTL2 in pancreatic cancer. Mol Ther Nucleic Acids. 2020;22:179–95.
Jiang Y, et al. Exosomal long noncoding RNA HOXD-AS1 promotes prostate cancer metastasis via miR-361-5p/FOXM1 axis. Cell Death Dis. 2021;12(12):1129.
Liang Y, et al. LncRNA BCRT1 promotes breast cancer progression by targeting miR-1303/PTBP3 axis. Mol Cancer. 2020;19(1):85.
Serafini FL, et al. Diagnostic impact of radiological findings and extracellular vesicles: are we close to radiovesicolomics? Biology. 2021;10(12):1265.
Whiteside TL. Exosome and mesenchymal stem cell cross-talk in the tumor microenvironment. Semin Immunol. 2018;35:69–79.
Zhang X, et al. Hypoxic BMSC-derived exosomal miRNAs promote metastasis of lung cancer cells via STAT3-induced EMT. Mol Cancer. 2019;18(1):40.
Chang L, et al. Exosomes derived from miR-1228 overexpressing bone marrow-mesenchymal stem cells promote growth of gastric cancer cells. Aging (Albany NY). 2021;13(8):11808–21.
Zhang X, et al. Hypoxic BMSC-derived exosomal miRNAs promote metastasis of lung cancer cells via STAT3-induced EMT. Mol Cancer. 2019;18(1):1–15.
Qin F, et al. Bone marrow-derived mesenchymal stem cell-derived exosomal microRNA-208a promotes osteosarcoma cell proliferation, migration, and invasion. J Cell Physiol. 2020;235(5):4734–45.
Xie H, Wang J. MicroRNA-320a-containing exosomes from human umbilical cord mesenchymal stem cells curtail proliferation and metastasis in lung cancer by binding to SOX4. J Recept Signal Transduct Res. 2021. https://doi.org/10.1080/10799893.2021.1918166 .
Bolandi Z, et al. Adipose derived mesenchymal stem cell exosomes loaded with miR-10a promote the differentiation of Th17 and Treg from naive CD4(+) T cell. Life Sci. 2020;259: 118218.
Shi Y, et al. Tumour-associated mesenchymal stem/stromal cells: emerging therapeutic targets. Nat Rev Drug Discov. 2017;16(1):35–52.
Schneider BP, Miller KD. Angiogenesis of Breast Cancer. J Clin Oncol. 2005;23(8):1782–90.
Coultas L, Chawengsaksophak K, Rossant J. Endothelial cells and VEGF in vascular development. Nature. 2005;438(7070):937–45.
Olsson A-K, et al. VEGF receptor signalling? In control of vascular function. Nat Rev Mol Cell Biol. 2006;7(5):359–71.
Carmeliet P. VEGF as a key mediator of angiogenesis in cancer. Oncology. 2005;69(Suppl. 3):4–10.
Melincovici CS, et al. Vascular endothelial growth factor (VEGF)—key factor in normal and pathological angiogenesis. Rom J Morphol Embryol. 2018;59(2):455–67.
Google Scholar
Vallabhaneni KC, et al. Extracellular vesicles from bone marrow mesenchymal stem/stromal cells transport tumor regulatory microRNA, proteins, and metabolites. Oncotarget. 2015;6(7):4953–67.
Lee J-K, et al. Exosomes derived from mesenchymal stem cells suppress angiogenesis by down-regulating VEGF expression in breast cancer cells. PLoS ONE. 2014;8(12): e84256.
Forsythe JA, et al. Activation of vascular endothelial growth factor gene transcription by hypoxia-inducible factor 1. Mol Cell Biol. 1996;16(9):4604–13.
Humar R, et al. Hypoxia enhances vascular cell proliferation and angiogenesis in vitro via rapamycin (mTOR)-dependent signaling. Faseb j. 2002;16(8):771–80.
Wang S, et al. The mTOR/AP-1/VEGF signaling pathway regulates vascular endothelial cell growth. Oncotarget. 2016;7(33):53269–76.
Del Bufalo D, et al. Antiangiogenic potential of the mammalian target of rapamycin inhibitor temsirolimus. Can Res. 2006;66(11):5549–54.
Pakravan K, et al. MicroRNA-100 shuttled by mesenchymal stem cell-derived exosomes suppresses in vitro angiogenesis through modulating the mTOR/HIF-1α/VEGF signaling axis in breast cancer cells. Cell Oncol (Dordr). 2017;40(5):457–70.
Bruno S, et al. Effects of mesenchymal stromal cell-derived extracellular vesicles on tumor growth. Front Immunol. 2014;5:382–382.
Zhou X, et al. Estrogen regulates Hippo signaling via GPER in breast cancer. J Clin Investig. 2015;125(5):2123–35.
Taciak B, et al. Wnt signaling pathway in development and cancer. J Physiol Pharmacol. 2018;69(2):185–96.
Lin R, Wang S, Zhao RC. Exosomes from human adipose-derived mesenchymal stem cells promote migration through Wnt signaling pathway in a breast cancer cell model. Mol Cell Biochem. 2013;383(1):13–20.
Wang Y, Cai X. Long noncoding RNA HAND2-AS1 restrains proliferation and metastasis of breast cancer cells through sponging miR-1275 and promoting SOX7. Cancer Biomark. 2020;27(1):85–94.
Wei M, Liu L, Wang Z. Long non-coding RNA heart and neural crest derivatives expressed 2-antisense RNA 1 overexpression inhibits the proliferation of cancer cells by reducing RUNX2 expression in triple-negative breast cancer. Oncol Lett. 2019;18(6):6775–80.
Xing L, et al. LncRNA HAND2-AS1 suppressed the growth of triple negative breast cancer via reducing secretion of MSCs derived exosomal miR-106a-5p. Aging. 2020;13(1):424–36.
Yuan L, et al. Exosomes derived from MicroRNA-148b-3p-overexpressing human umbilical cord mesenchymal stem cells restrain breast cancer progression. Front Oncol. 2019;9:1076.
Vakhshiteh F, et al. Exosomes derived from miR-34a-overexpressing mesenchymal stem cells inhibit in vitro tumor growth: a new approach for drug delivery. Life Sci. 2021;266: 118871.
Sheykhhasan M, et al. Exosomes of mesenchymal stem cells as a proper vehicle for transfecting miR-145 into the breast cancer cell line and its effect on metastasis. Biomed Res Int. 2021;2021:5516078.
Li K, et al. High cholesterol induces apoptosis and autophagy through the ROS-activated AKT/FOXO1 pathway in tendon-derived stem cells. Stem Cell Res Ther. 2020;11(1):131.
Fleisher TA. Apoptosis. Ann Allergy Asthma Immunol. 1997;78(3):245–9 ( quiz 249-50 ).
Mathiassen SG, De Zio D, Cecconi F. Autophagy and the cell cycle: a complex landscape. Front Oncol. 2017;7:51.
Hewitt G, Korolchuk VI. Repair, reuse, recycle: the expanding role of autophagy in genome maintenance. Trends Cell Biol. 2017;27(5):340–51.
Levy JMM, Towers CG, Thorburn A. Targeting autophagy in cancer. Nat Rev Cancer. 2017;17(9):528–42.
Wang Y, et al. miR-224-5p carried by human umbilical cord mesenchymal stem cells-derived exosomes regulates autophagy in breast cancer cells via HOXA5. Front Cell Dev Biol. 2021;9:679185.
Tan P, et al. TRIM59 promotes breast cancer motility by suppressing p62-selective autophagic degradation of PDCD10. PLoS Biol. 2018;16(11): e3000051.
Pourakbari R, et al. Identification of genes and miRNAs associated with angiogenesis, metastasis, and apoptosis in colorectal cancer. Gene Rep. 2020;18: 100552.
Polacheck WJ, Zervantonakis IK, Kamm RD. Tumor cell migration in complex microenvironments. Cell Mol Life Sci. 2013;70(8):1335–56.
Kong D, et al. VEGF-C mediates tumor growth and metastasis through promoting EMT-epithelial breast cancer cell crosstalk. Oncogene. 2021;40(5):964–79.
Du L, Tao X, Shen X. Human umbilical cord mesenchymal stem cell-derived exosomes inhibit migration and invasion of breast cancer cells via miR-21-5p/ZNF367 pathway. Breast Cancer. 2021;28(4):829–37.
Shojaei S, et al. Delivery of miR-381-3p mimic by mesenchymal stem cell-derived exosomes inhibits triple negative breast cancer aggressiveness; an in vitro study. Stem Cell Rev Rep. 2021;17(3):1027–38.
Egea V, et al. Let-7f miRNA regulates SDF-1α- and hypoxia-promoted migration of mesenchymal stem cells and attenuates mammary tumor growth upon exosomal release. Cell Death Dis. 2021;12(6):516.
Recasens A, Munoz L. Targeting cancer cell dormancy. Trends Pharmacol Sci. 2019;40(2):128–41.
Endo H, Inoue M. Dormancy in cancer. Cancer Sci. 2019;110(2):474–80.
Gomatou G, et al. Tumor dormancy: implications for invasion and metastasis. Int J Mol Sci. 2021;22(9):4862.
Casson J, et al. Mesenchymal stem cell-derived extracellular vesicles may promote breast cancer cell dormancy. J Tissue Eng. 2018;9:2041731418810093.
Sandiford OA, et al. Mesenchymal stem cell-secreted extracellular vesicles instruct stepwise dedifferentiation of breast cancer cells into dormancy at the bone marrow perivascular region. Can Res. 2021;81(6):1567–82.
Bliss SA, et al. Mesenchymal stem cell-derived exosomes stimulate cycling quiescence and early breast cancer dormancy in bone marrow. Cancer Res. 2016;76(19):5832–44.
Mohd Ali N, et al. Adipose MSCs suppress MCF7 and MDA-MB-231 breast cancer metastasis and EMT pathways leading to dormancy via exosomal-miRNAs following co-culture interaction. Pharmaceuticals (Basel). 2020;14(1):8.
Ono M, et al. Exosomes from bone marrow mesenchymal stem cells contain a microRNA that promotes dormancy in metastatic breast cancer cells. Sci Signal. 2014;7(332):ra63.
Zargar A, et al. Overcoming the challenges of cancer drug resistance through bacterial-mediated therapy. Chronic Dis Transl Med. 2019;5(4):258–66.
Vasan N, Baselga J, Hyman DM. A view on drug resistance in cancer. Nature. 2019;575(7782):299–309.
Wang X, Zhang H, Chen X. Drug resistance and combating drug resistance in cancer. Cancer Drug Resist. 2019;2(2):141–60.
Roodhart JM, et al. Mesenchymal stem cells induce resistance to chemotherapy through the release of platinum-induced fatty acids. Cancer Cell. 2011;20(3):370–83.
Ji R, et al. Exosomes derived from human mesenchymal stem cells confer drug resistance in gastric cancer. Cell Cycle (Georget, Tex). 2015;14(15):2473–83.
Luo T, et al. Mesenchymal stem cell-secreted exosome promotes chemoresistance in breast cancer via enhancing miR-21-5p-mediated S100A6 expression. Mol Ther Oncolytics. 2020;19:283–93.
Jia Z, et al. Adipose mesenchymal stem cell-derived exosomal microRNA-1236 reduces resistance of breast cancer cells to cisplatin by suppressing SLC9A1 and the Wnt/β-catenin signaling. Cancer Manag Res. 2020;12:8733–44.
Schreiber RD, Old LJ, Smyth MJ. Cancer immunoediting: integrating immunity’s roles in cancer suppression and promotion. Science. 2011;331(6024):1565–70.
Bates JP, et al. Mechanisms of immune evasion in breast cancer. BMC Cancer. 2018;18(1):556.
Gil Del Alcazar CR, Alečković M, Polyak K. Immune escape during breast tumor progression. Cancer Immunol Res. 2020;8(4):422–7.
Bagati A, et al. Integrin αvβ6-TGFβ-SOX4 pathway drives immune evasion in triple-negative breast cancer. Cancer Cell. 2021;39(1):54-67.e9.
Yao X, et al. Endoplasmic reticulum stress-induced exosomal miR-27a-3p promotes immune escape in breast cancer via regulating PD-L1 expression in macrophages. J Cell Mol Med. 2020;24(17):9560–73.
Biswas S, et al. Exosomes produced by mesenchymal stem cells drive differentiation of myeloid cells into immunosuppressive M2-polarized macrophages in breast cancer. J Investig Med. 2019;203(12):3447–60.
Download references
Acknowledgements
The authors are grateful to Scientific Research Deanship at King Khalid University, Abha, Saudi Arabia for their financial support through the Large Research Group Project under grant number (RGP.02-87-43).
This work was financially supported by Scientific Research Deanship at King Khalid University, Abha, Saudi Arabia, through the Large Research Group Project under grant number (RGP.02-87-43). The funding bodies played no role in the design of the study and collection, analysis, and interpretation of data and in writing the manuscript.
Author information
Authors and affiliations.
Department of Radiological Techniques, Al-Mustaqbal University College, Babylon, Iraq
Ghaidaa Raheem Lateef Al-Awsi
College of Pharmacy, Prince Sattam Bin Abdulaziz University, Alkharj, Kingdom of Saudi Arabia
Fahad Alsaikhan
Department of Anatomy, Faculty of Medicine, Universitas Indonesia, Jakarta, Indonesia
Ria Margiana
Master’s Programme Biomedical Sciences, Faculty of Medicine, Universitas Indonesia, Jakarta, Indonesia
Andrology Program, Faculty of Medicine, Universitas Airlangga, Surabaya, Indonesia
Dr. Soetomo General Academic Hospital, Surabaya, Indonesia
Department of Clinical Laboratory Sciences, College of Applied Medical Sciences, King Khalid University, Abha, Saudi Arabia
Irfan Ahmad
Independent Researcher, Durgapur, West Bengal, India
Indrajit Patra
Pharmaceutical Chemistry Department, College of Pharmacy, Al-Ayen University, Thi-Qar, Iraq
Mazin A. A. Najm
Department of Botany, Bahauddin Zakariya University, Multan, Pakistan
Ghulam Yasin
Independent Researcher, “Kasmed” Private Medical Centre, Tashkent, Uzbekistan
Iroda Rasulova
Computer Engineering Techniques Department, Faculty of Information Technology, Imam Ja’afar Al-Sadiq University, Baghdad, Iraq
Ali Thaeer Hammid
Veterinary Medicine College, Al-Qasim Green University, Al-Qasim, Iraq
Hamzah H. Kzar
College of Medicine, University of Al-Ameed, Karbala, Iraq
Moaed E. Al-Gazally
Department of Immunology, Faculty of Medicine, Tabriz University of Medical Sciences, Tabriz, Iran
Homayoon Siahmansouri
You can also search for this author in PubMed Google Scholar
Contributions
GRLA, FA, RM and IA have drafted the work; IP, MAAN, GY, IR and ATH designed the figures and table and edited the manuscript; and HHK, MEA and HS designed the work. All authors read and approved the final manuscript.
Corresponding authors
Correspondence to Ria Margiana or Homayoon Siahmansouri .
Ethics declarations
Ethics approval and consent to participate, consent for publication, competing interests.
The authors indicated no potential conflicts of interest.
Additional information
Publisher's note.
Springer Nature remains neutral with regard to jurisdictional claims in published maps and institutional affiliations.
Rights and permissions
Open Access This article is licensed under a Creative Commons Attribution 4.0 International License, which permits use, sharing, adaptation, distribution and reproduction in any medium or format, as long as you give appropriate credit to the original author(s) and the source, provide a link to the Creative Commons licence, and indicate if changes were made. The images or other third party material in this article are included in the article's Creative Commons licence, unless indicated otherwise in a credit line to the material. If material is not included in the article's Creative Commons licence and your intended use is not permitted by statutory regulation or exceeds the permitted use, you will need to obtain permission directly from the copyright holder. To view a copy of this licence, visit http://creativecommons.org/licenses/by/4.0/ . The Creative Commons Public Domain Dedication waiver ( http://creativecommons.org/publicdomain/zero/1.0/ ) applies to the data made available in this article, unless otherwise stated in a credit line to the data.
Reprints and permissions
About this article
Cite this article.
Al-Awsi, G.R.L., Alsaikhan, F., Margiana, R. et al. Shining the light on mesenchymal stem cell-derived exosomes in breast cancer. Stem Cell Res Ther 14 , 21 (2023). https://doi.org/10.1186/s13287-023-03245-3
Download citation
Received : 26 April 2022
Accepted : 18 January 2023
Published : 08 February 2023
DOI : https://doi.org/10.1186/s13287-023-03245-3
Share this article
Anyone you share the following link with will be able to read this content:
Sorry, a shareable link is not currently available for this article.
Provided by the Springer Nature SharedIt content-sharing initiative
- Mesenchymal stem cell
Stem Cell Research & Therapy
ISSN: 1757-6512
- Submission enquiries: Access here and click Contact Us
- General enquiries: [email protected]
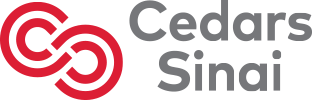
- See All Locations
- Primary Care
- Urgent Care Facilities
- Emergency Rooms
- Surgery Centers
- Medical Offices
- Imaging Facilities
- Browse All Specialties
- Diabetes & Endocrinology
- Digestive & Liver Diseases
- Ear, Nose & Throat
- General Surgery
- Neurology & Neurosurgery
- Obstetrics & Gynecology
- Orthopaedics
- Pain Medicine
- Pediatrics at Guerin Children’s
- Urgent Care
- Medical Records Request
- Insurance & Billing
- Pay Your Bill
- Advanced Healthcare Directive
- Initiate a Request
- Help Paying Your Bill

Stem Cell Discoveries Hold Potential to Improve Cancer Treatment
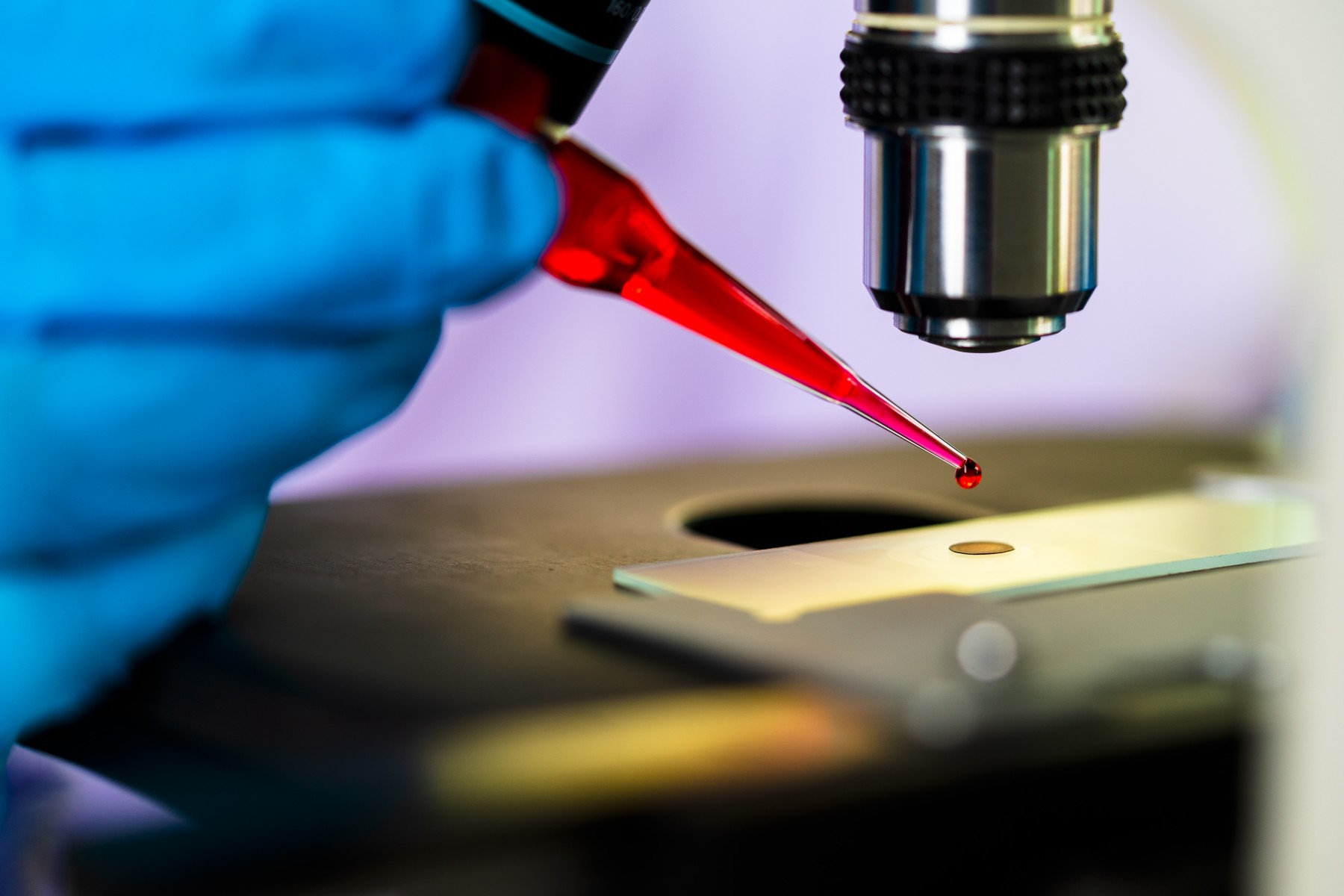
Findings Could Lead to New Ways to Fight Disease and Help Patients Recover Faster
Two recent discoveries by stem cell scientists at Cedars-Sinai may help make cancer treatment more efficient and shorten the time it takes for people to recover from radiation and chemotherapy.
In the first study, published in the journal Blood , investigators discovered a protein that is expressed by blood stem cells that could aid in identifying, studying and deploying the cells for treatments.
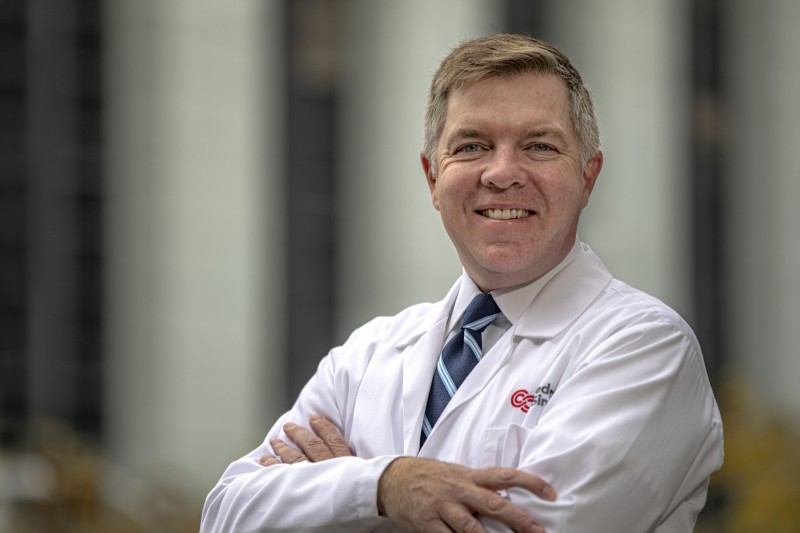
Blood stem cells are found in small quantities in the bone marrow and in peripheral blood—the type that travels through the heart, arteries, capillaries and veins. These stem cells are of interest to scientists because they produce all blood cells and immune cells in the body. They are used in the curative treatment of people with leukemia and lymphoma.
This approach faces a major challenge: Hematopoietic stem cells make up less than 0.01% of cells in the bone marrow and peripheral blood, and there is not yet a good way to separate them from other cells. This means that when people receive infusions of bone marrow and peripheral blood cells, they get a tiny number of stem cells that are therapeutic along with a lot of other cells that are not.
To study this phenomenon, investigators at the Chute laboratory led by first author Christina M. Termini, PhD, extracted bone marrow cells from adult mice and ran the samples through a device that can detect hundreds of different types of cells based on the proteins that live on their surfaces. This process revealed that hematopoietic stem cells have a high concentration of syndecan-2, which is part of a family of proteins called heparan sulfate proteoglycans, on the cell surface.
The researchers found this protein plays an important role in how hematopoietic stem cells reproduce. When stem cells that express syndecan-2 were transplanted into mice following irradiation, their cells repopulated. But when stem cells that lacked syndecan-2 were transplanted, the cells stopped replicating.
By transplanting only cells that express syndecan-2, it may be possible to make blood stem cell transplants more efficient and less toxic.
Second Discovery
The second discovery by Chute and his team—published in the journal Nature Communications —revealed a mechanism through which the blood vessels in the bone marrow respond to injury, such as from chemotherapy or radiation.
When people receive radiation or chemotherapy as part of their cancer treatment, their blood counts plummet. It typically takes several weeks for these counts to return to normal levels.
Chute and colleages found that when mice receive radiation treatment, the cells that line the inner walls of the blood vessels in the bone marrow produce a protein called semaphorin 3A. This protein tells another protein, called neuropilin 1, to kill damaged blood vessels in the bone marrow.
When the investigators blocked the ability of these blood vessel cells to produce neuropilin 1 or semaphorin 3A, or injected an antibody that blocks semaphorin 3A communication with neuropilin 1, the bone marrow vasculature regenerated following irradiation. In addition, blood counts increased dramatically after one week.
“We've discovered a mechanism that appears to control how blood vessels regenerate following injury,” said Chute, senior author of the paper. “Inhibiting this mechanism causes rapid recovery of the blood vessels and blood cells in bone marrow following chemotherapy or irradiation. In principle, targeting this mechanism could allow patients to recover following chemotherapy in one to two weeks, instead of three or four weeks as currently experienced.”
Termini, a post-doctoral scientist at the David Geffen School of Medicine at UCLA, was the first author on both studies.
Funding: The research reported in the Blood study was funded by the National Heart, Lung, and Blood Institute under award number HL086998, the National Institute of Allergy and Infectious Diseases under award numbers AI107333 and AI067769, the California Institute for Regenerative Medicine Leadership Award under award number LA1-08014, the National Institutes of Health under award numbers K01 1K01DK126989-01A1 and K08 1K08HL138305, the Damon Runyon Cancer Foundation under award number DRG-2327-18, the Burroughs Wellcome Fund Postdoctoral Diversity Enrichment Program under award number 1018686, and the University of California President’s Postdoctoral Fellowship.
Funding: The research reported in the Nature Communications study was funded by the National Heart, Lung, and Blood Institute under award number HL-086998, the National Institute of Allergy and Infectious Diseases under award numbers AI-067769 and AI-138331, the California Institute for Regenerative Medicine Leadership under award number LA1-08014, the National Institutes of Health under award numbers K01 1K01DK126989-01A1 and K08 1K08HL138305, the Damon Runyon Cancer Foundation under award number DRG-2327-18, the Burroughs Wellcome Fund Postdoctoral Diversity Enrichment Program under award number 1018686, the University of California President’s Postdoctoral Fellowship, and the Tower Cancer Research Foundation Career Development Grant.
Read more about the Chute Laboratory at Cedars-Sinai .
Related Stories
Cardiologists train large ai model to assess heart structure, function, smidt heart institute, cedars-sinai investigators train an echocardiography foundation model 10 times larger than models used in previous efforts.
Artificial intelligence experts at Cedars-Sinai and the Smidt Heart Institute created a dataset with more than 1 million echocardiograms, or cardiac ultrasound videos, and their corresponding clinical interpretations. Using this database, …
April Monthly Research Highlights Newsletter
A roundup of the latest medical discoveries and faculty news at cedars-sinai.
More than 100 faculty members who attended Cedars-Sinai’s April Research Town Hall—hosted by Jeffrey A. Golden, MD—learned how to best collaborate with …
Research Town Hall Spotlights New Human Microbiome Research Institute, Access to EIS Programs
Attendees at the quarterly event learned how to partner with cedars-sinai’s human microbiome research institute and research eis enterprise.
More than 100 faculty members who attended Cedars-Sinai’s April Research Town Hall—hosted by Jeffrey A. Golden, MD, executive vice dean of Research and Education and director of the Burns and Allen Research Institute at Cedars-Sinai—learned how …

Researchers develop a new way to safely boost immune cells to fight cancer
Cancer is the monster of our society. Last year alone, more than 600,000 people in the United States died from cancer, according to the American Cancer Society. The relentless pursuit of understanding this complex disease has shaped medical progress on developing treatment procedures that are less invasive while still highly effective.
Immunotherapy is on the rise as a possible solution. Immunotherapy involves harnessing the power of the body's immune system to fight against cancer cells. Researchers in the College of Engineering have found a way to revamp a treatment procedure into a groundbreaking practice.
Rong Tong, associate professor in chemical engineering, has teamed up with Wenjun "Rebecca" Cai, associate professor in materials science and engineering, to explore a cancer immunotherapy treatment that has long been of interest to researchers. In their newly published article in the journal Science Advances, Tong and Cai detailed their approach, which involves activating the immune cells in the body and reprogramming them to attack and destroy the cancer cells. This therapeutic method is frequently implemented with the protein cytokine. Cytokines are small protein molecules that act as intercellular biochemical messengers and are released by the body's immune cells to coordinate their response.
"Cytokines are potent and highly effective at stimulating the immune cells to eliminate cancer cells," Tong said. "The problem is they're so potent that if they roam freely throughout the body, they'll activate every immune cell they encounter, which can cause an overactive immune response and potentially fatal side effects."
Tong and Cai, in collaboration with chemical engineering and materials science and engineering graduate students, have developed an innovative approach to employ cytokine proteins as a potential immunotherapy treatment. Unlike previous methods, their technique ensures that the immune cell stimulating cytokines effectively localize within the tumors for weeks while preserving the cytokine's structure and reactivity levels.
Combining forces to take down cancer cells
Current cancer treatments, such as chemotherapy, cannot distinguish between healthy cells and cancer cells. When someone with cancer is treated with chemotherapy, the treatment attacks all of the cells in their body, which can lead to side effects such as hair loss and fatigue. Stimulating the body's immune system to attack tumors is a promising alternative to treat cancer. The delivery of cytokines can jump-start immune cells in the tumor, but overstimulating healthy cells can cause severe side effects.
"Scientists determined a while ago that cytokines can be used to activate and fight against tumors, but they didn't know how to localize them inside the tumor while not exposing toxicity to the rest of the body," said Tong. "Chemical engineers can look at this from an engineering approach and use their knowledge to help refine and elevate the effectiveness of the cytokines so they can work inside the body effectively."
The research team's goal is to find a balance between killing cancer cells in the body while sparing healthy cells.
To accomplish this goal, Tong and his students used their expertise to create specialized particles with distinctive sizes that help determine where the drug is going. These microparticles are designed to stay within the tumor environment after being injected into the body. Cai and her students worked on measuring these particles' surface properties.
"In the field of materials science and engineering, we study the surface chemistry and mechanical behavior of materials, such as the specialized particle created for this project," Cai said. "Surface engineering and characterization, along with particle size, play important roles in controlled drug delivery, ensuring prolonged drug presence and sustained therapeutic effectiveness."
To ensure successful drug delivery, Tong and his chemical engineering students designed a novel strategy that:
- Anchors cytokines to these new microparticles, limiting the harm of cytokines to healthy cells
- Allows the newly particle-anchored cytokines to jump-start immune systems and recruit immune cells to attack cancer cells
"Our strategy not only minimizes cytokine-induced harm to healthy cells, but also prolongs cytokine retention within the tumor," Tong said. "This helps facilitate the recruitment of immune cells for targeted tumor attack."
The next step in the process involves combining the new, localized cytokine therapy method with commercially available, Food and Drug Administration (FDA)-approved checkpoint blockade antibodies, which reactivate the tumor immune cells that have been silenced so they can fight back the cancer cells.
"When there is a tumor inside the body, the body's immune cells are being deactivated by the cancer cells," Tong explained. "The FDA-approved checkpoint blocking antibody helps "take off the brakes" that tumors put on immune cells, while the cytokine molecules "step on the gas" to jump-start the immune system and get an immune cell army to fight cancer cells. These two approaches work together to activate immune cells."
Combining the checkpoint antibodies with the particle-anchored cytokine proved to successfully eliminate many tumors in their study.
Engineering an impact on cancer treatment
Team members hope their impact on immunotherapy treatment is part of a greater movement toward cancer treatment approaches that are harmless to healthy cells. The new approach of attaching cytokines to particles also could be used in the future to deliver other types of immunostimulatory drugs, according to the team.
"Researchers are still looking for safer and more effective cancer treatments," said Tong. "This motivation is what drives us to develop new technologies in the field. The whole class of drugs that are employed to jump-start the immune system to fight cancer cells has largely not yet succeeded. Our goal is to create novel solutions that allow researchers to test these drugs with existing FDA-approved therapeutics, ensuring both safety and enhanced efficacy."
Cai said the nature of cancer treatment research requires expertise across engineering disciplines.
"I view this project as a perfect marriage between chemical engineering and materials science," Cai said. "The former focuses on the synthesis and drug delivery part, the latter on applying advanced materials characterization. This collaboration not only accelerates immunotherapy research, but also has the ability to transform cancer treatment."
- Immune System
- Brain Tumor
- Lung Cancer
- Prostate Cancer
- Colon Cancer
- Skin Cancer
- Immune system
- Chemotherapy
- Monoclonal antibody therapy
- Natural killer cell
- White blood cell
- Prostate cancer
- Endocrine system
Story Source:
Materials provided by Virginia Tech . Original written by Hailey Wade. Note: Content may be edited for style and length.
Journal Reference :
- Liqian Niu, Eungyo Jang, Ai Lin Chin, Ziyu Huo, Wenbo Wang, Wenjun Cai, Rong Tong. Noncovalently particle-anchored cytokines with prolonged tumor retention safely elicit potent antitumor immunity . Science Advances , 2024; 10 (16) DOI: 10.1126/sciadv.adk7695
Cite This Page :
Explore More
- Anticoagulant With an On-Off Switch
- Sleep Resets Brain Connections -- At First
- Far-Reaching Effects of Exercise
- Hidden Connections Between Brain and Body
- Novel Genetic Plant Regeneration Approach
- Early Human Occupation of China
- Journey of Inhaled Plastic Particle Pollution
- Earth-Like Environment On Ancient Mars
- A 'Cosmic Glitch' in Gravity
- Time Zones Strongly Influence NBA Results
Trending Topics
Strange & offbeat.
- Type 2 Diabetes
- Heart Disease
- Digestive Health
- Multiple Sclerosis
- Diet & Nutrition
- Supplements
- Health Insurance
- Public Health
- Patient Rights
- Caregivers & Loved Ones
- End of Life Concerns
- Health News
- Thyroid Test Analyzer
- Doctor Discussion Guides
- Hemoglobin A1c Test Analyzer
- Lipid Test Analyzer
- Complete Blood Count (CBC) Analyzer
- What to Buy
- Editorial Process
- Meet Our Medical Expert Board
What Are Cancer Stem Cells?
They influence how a tumor grows
Role in Cancer Growth
Resistance to therapy, importance of cancer stem cell research.
Cancer stem cells are a small subpopulation of cells found within tumors that are tumorigenic, meaning they can create a cancerous tumor. Self-renewal and the ability to differentiate into diverse cell types are hallmark features of cancer stem cells. They can reproduce themselves and sustain cancer in the body. They are therefore hypothesized to be the primary driver of cancer growth and metastasis . This is called the stem cell theory of cancer. Effective cancer treatment then must target and attack these cells. Doing so can improve the chances of cancer remission.
Cancer stem cells have been identified in brain , breast , colon , ovarian , pancreatic , and prostate tumors, as well as in melanoma , multiple myeloma , nonmelanoma skin cancer , and leukemia .
Cancer stem cell research is ongoing, and new studies are emerging frequently.
What Are Stem Cells?
Stem cells are undifferentiated (or only partly differentiated) human cells that can turn into different types of cells in the body, from nerve cells (neurons) to brain cells. They can also fix damaged tissues. They must possess two major qualities: self-renewal and the capacity to differentiate. Stem cell-based therapies are also being studied to treat serious illnesses such as paralysis and Alzheimer's disease .
There are two types of stem cells: embryonic and adult stem cells. Embryonic stem cells come from unused embryos and are created from an in vitro fertilization process. They are pluripotent, meaning they can turn into more than one cell type. Within adult stem cells, there are two different types: one type comes from fully developed tissues such as the brain, skin, and bone marrow, and the other is induced pluripotent stem cells, which have been changed in the lab to be more like embryonic stem cells.
luismmolina / Getty Images
The stem cell theory of cancer hypothesizes that cancer stem cells are thought to drive tumor initiation and may be responsible for therapeutic resistance and cancer recurrence.
Like many areas of biomedical research, cancer stem cells are an evolving field of study. Multiple studies have indicated that insufficient evidence exists to confirm the existence of cancer stem cells. A review of 1,000 Web of Science publications revealed that only 49% supported the cancer stem cell hypothesis.
Cell surface markers can be used to identify cancer stem cells, as has been done in research that supports the hypothesis that these stem cells do not respond to traditional therapies such as chemotherapy . This research also supports the idea that cancer stem cells are the source of cancer metastasis.
Like all stem cells, cancer cells must have the following characteristics:
- Self-renewal: When stem cells divide into more stem cells, this process is referred to as cell renewal.
- Cell differentiation: Cell differentiation is when a cell changes from a less differentiated to a more differentiated cell type.
Cancer stem cells use specific signaling pathways. It is hypothesized that cancer stem cells can also act as a reservoir of cancer cells, which may cause a relapse after surgery, radiation, or chemotherapy has eliminated all observable signs of cancer. Targeting these cells would thus highly improve the chances of a patient's remission if cancer stem cells are the origin of the tumor.
Cancer stem cells have the capacity to change into more specialized cell types, so they can potentially lead to tumor cell heterogeneity. Due to this quality, they are cited as a major factor of chemoresistance. Their highly resistant nature can lead to tumors metastasizing and tumor regrowth. As such, the developing research on cancer stem cells could dramatically change the prognosis of multiple cancer types.
Also, many new anticancer therapies are evaluated based on their ability to shrink tumors, but if the therapies are not killing the cancer stem cells, the tumor will soon grow back, often with resistance to the previously used therapy.
Cancer stem cell research is critical because it addresses the potential root cause of cancer proliferation and can lead to the development of more effective and safer treatments. Treatments targeting cancer stem cells will likely have fewer side effects compared with existing options because they will leave other kinds of cells untouched.
Understanding these cells can also help modify current treatments for maximum effect. Research has shown that cancer stem cells are resistant to the ionizing radiation used to treat cancer. Understanding this resistance may in the future help researchers find compounds that undermine this process and make cancer stem cells vulnerable to radiation damage.
A Word From Verywell
Cancer stem cell research offers promising hope for the continually evolving field of cancer therapeutics, but more research needs to be done to confirm the stem cell theory of cancer. Cancer stem cell research has the potential to generate better treatments for cancer with fewer side effects, as well as to improve the efficacy of current treatment options. If the theory is proven, therapies targeting cancer stem cells may even be able to lower the rate of cancer recurrence. While its existence is still up for debate, it represents an exciting opportunity to advance cancer care and improve cancer survival.
Yu Z, Pestell TG, Lisanti MP, Pestell RG. Cancer stem cells . Int J Biochem Cell Biol . 2012 Dec;44(12):2144-51. doi: 10.1016/j.biocel.2012.08.022
Ayob AZ, Ramasamy TS. Cancer stem cells as key drivers of tumour progression . J Biomed Sci . 2018 Mar 6;25(1):20. doi: 10.1186/s12929-018-0426-4
Stanford Children's Health. What are stem cells?
Walcher L, Kistenmacher AK, Suo H, Kitte R, Dluczek S, Strauß A, Blaudszun AR, Yevsa T, Fricke S, Kossatz-Boehlert U. Cancer stem cells-origins and biomarkers: perspectives for targeted personalized therapies . Front Immunol . 2020 Aug 7;11:1280. doi: 10.3389/fimmu.2020.01280
Bartram I, Jeschke JM. Do cancer stem cells exist? A pilot study combining a systematic review with the hierarchy-of-hypotheses approach . PLoS One . 2019 Dec 13;14(12):e0225898. doi: 10.1371/journal.pone.0225898
Dawood S, Austin L, Cristofanilli M. Cancer stem cells: implications for cancer therapy . Oncology (Williston Park) . 2014;28(12):1101-1110.
Stanford Medicine. The stem cell theory of cancer .
Nassar D, Blanpain C. Cancer stem cells: basic concepts and therapeutic implications. Annu Rev Pathol . 2016;11:47-76. doi:10.1146/annurev-pathol-012615-044438
Barbato L, Bocchetti M, Di Biase A, Regad T. Cancer stem cells and targeting strategies. Cells . 2019;8(8):926. doi:10.3390/cells8080926
Ayob AZ, Ramasamy TS. Cancer stem cells as key drivers of tumour progression. J Biomed Sci. 2018;25(1):20. doi.org/10.1186/s12929-018-0426-4
Stanford Medicine. What CSCs mean for cancer treatment .

Uncovering how skin stem cells survive the stressful environment of wounds
April 26, 2024
By Devon McPhee
When you get a cut or a scrape, the body floods the wounded area with immune cells such as neutrophils, monocytes, and macrophages to protect the area from infection and to clear it of dead cells and molecular debris. These immune cells not only secrete anti-microbial molecules, but also small proteins called cytokines that cause inflammation.
At the same time, the body recruits epithelial stem cells to repair the skin. These vulnerable cells must protect themselves from the harsh, inflammatory environment caused by the immune cells to complete their task of regenerating skin cells. The question is: how?
Researchers at the University of Chicago, in collaboration with the Howard Hughes Medical Institute and The Rockefeller University, have identified an answer – and it refutes a long-held belief of many in the field.
“It has long been thought that skin stem cells must operate in a specialized microenvironment known as a niche in order to survive the inflammatory environment of a wound,” said Yuxuan (Phoenix) Miao , assistant professor in the Ben May Department of Cancer Research at the University of Chicago and lead author of the paper. “What we discovered was that the stem cells are able to protect themselves by manipulating regulatory T-cells – specialized cells that suppress immune response – to create a barrier between themselves and the wounds’ harsh environment.”
Their findings were published online April 26 in Immunity and will also appear in the May print issue of the journal.
A mechanism for self-protection
To study this phenomenon, the researchers began by using fluorescence-activated cell sorting to identify the subset of skin stem cells that enter the wound. Then, through RNA sequencing and flow cytometry analysis, they identified genes present only in this subset.
Unexpectedly, they found high concentrations of molecules such as CD80 that are known to modulate immune response by regulating the body’s disease-fighting T-cells. The molecules enable the skin stem cells to weave a web of regulatory T-cells that envelop and protect them in the wound as they work to repair damaged skin. These molecules were not found in healthy skin’s stems cells nor in other non-immune cells within the wounded skin.
“When we delved into the mechanisms behind the skin stem cell’s ability to work in the wound environment, we were surprised to find that they co-opted a mechanism thought to be used only by professional immune cells,” said Jingyun Luan, a postdoctoral researcher in the Miao Lab and first author of the paper. “Namely, the activation of molecules that expand the expression of T-cells–particularly regulatory T-cells, which suppress inflammation.”
The researchers had previously identified the presence of CD80 molecules in cancer stem cells and hypothesized that they played a role in the mechanism that allows cancer stem cells to evade the immune system.
The team confirmed the role of CD80 during skin repair by removing it from the skin stem cells within the wounds of mice models. They observed a significant decrease in regulatory T-cells and a marked increase in neutrophils, which when present in large amounts can worsen inflammation. The result was wounds that healed much more slowly.
Faster wound healing, slower cancer growth
These findings have implications for treating the slow-healing wounds of diabetics and older individuals, whose skin stem cells may have a reduced ability to produce the protective CD80 molecules in a wound environment. Reverse strategies could be used to treat cancer by reducing or eliminating the ability of cancer stem cells to evade the immune system.
Miao’s team now plans to further explore CD80’s role in cancer stem cells and to understand the features that enable it to increase the regulatory T-cells in tumors. Ultimately, they hope this information will lead to strategies that manipulate the molecule for therapeutic purposes.
“We’re excited to better understand the mechanisms behind this function,” said Miao. “This intricate, stem cell-driven orchestration of immunity during wound repair has significant implications for how we think about pathogenic immune responses.”
Additional authors include Aleksandra Vuchkovska, Weijie Guo, Jennifer Good, Bijun Liu, and Audrey Gang of UChicago; Cynthia Truong, Nicole Infarinato, Katherine Stewart, Lisa Polak, Emma Andretta, Alexander Y. Rudensky, and Elaine Fuchs of the Howard Hughes Medical Institute; and Hilda Amalia Pasolli of The Rockefeller University.
Explore the Biological Sciences Division
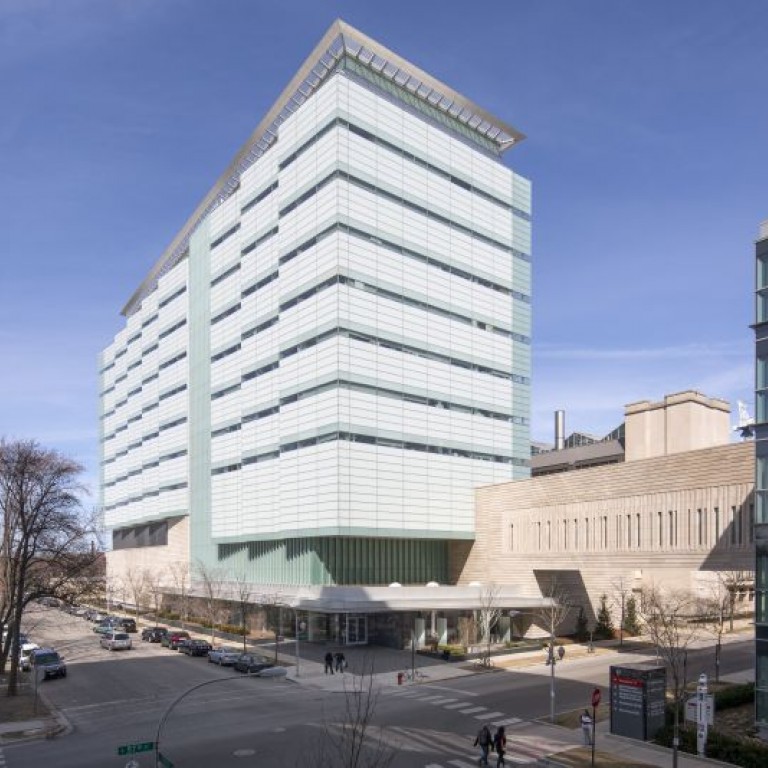
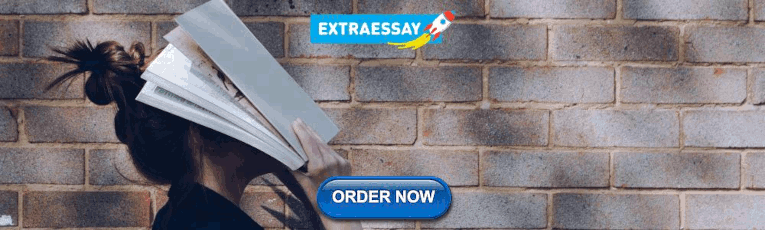
IMAGES
VIDEO
COMMENTS
Cancer stem cells are rare immortal cells within a tumour that can both self-renew by dividing and give rise to many cell types that constitute the tumour, and can therefore form tumours. Such ...
Cancer stem cells (CSCs) represent specific type of rare cells found in the broad majority of tumors, possessing self-renewal and differentiation capacities. ... and differentiation, in order to maintain the stem cell properties, offers new avenues for cancer treatments. Therefore, targeting the essential pathways, such as Notch, Wnt ...
Since cancer stem cells (CSCs) were first identified in leukemia in 1994, they have been considered promising therapeutic targets for cancer therapy. These cells have self-renewal capacity and ...
1. Introduction. Cancer is the most dangerous disease by causing millions of deaths worldwide [].Despite of rapid advancement in research of diagnostics and therapeutics, the death rate by cancer only declined ~1.5% annually in the period of 2006-2015 in United States [].Comprehensive knowledge about cancer biology would allow scientists to design better therapeutic systems.
FULL STORY. Two recent discoveries by stem cell scientists at Cedars-Sinai may help make cancer treatment more efficient and shorten the time it takes for people to recover from radiation and ...
Other tissues and organs in which cancer commonly develops, such as the skin, mammary glands, gut, prostate gland, liver, and even the brain, also contain stem cells. 7-12 The stem cells in each ...
Stanford researchers are currently searching for stem cells that underlie cancers of the blood, breast, ovaries, lung, brain and bladder, among others -- making the institute the global epicenter of the cancer stem cell hunt. Learning how cancer stem cells self-renew is the first step toward drugs that throw a wrench in the cancer propagation ...
November 28, 2023 at 6:00 a.m. EST. Scientists have discovered what they call vertebral skeletal stem cells in the spine, a finding that might have implications for treating cancer and improving ...
The test for the cancer stem cell (CSC) hypothesis is to find a target expressed on all, and only CSCs in a patient tumor, then eliminate all cells with that target that eliminates the cancer. ... Memorial Sloan Kettering Cancer Center, New York, New York. ... Ludwig Center for Cancer Stem Cell Research, Stanford University, Stanford ...
Recent advancements in stem cell technology open a new door for patients suffering from diseases and disorders that have yet to be treated. Stem cell-based therapy, including human pluripotent ...
Matosevic is also on the faculty of the Purdue Institute for Cancer Research and the Purdue Institute for ... So we eliminated the need for blood and instead differentiated stem cells into immune cells, or natural killer cells, and then genetically engineered those." ... "We are also open to and always seeking new collaborations and ...
Stem cells articles from across Nature Portfolio. ... Cancer stem cells; Embryonic germ cells; ... Research Highlights 29 Apr 2024 Cell Research. P: 1-2.
Over the last 2 decades, induced pluripotent stem cells (iPSCs) have had various potential applications in various medical research areas, from personalized medicine to disease treatment. Different cellular resources are accessible for iPSC generation, such as keratinocytes, skin fibroblasts, and blood or urine cells. However, all these sources are somatic cells, and we must make several ...
Stem cells survive much longer than ordinary cells, increasing the chance that they might accumulate genetic mutations. It might take only a few mutations for one cell to lose control over its self-renewal and growth and become the source of cancer. The idea that the remnants of our embryonic past could lead to our demise through cancer is ...
After CAR T-cell therapy, all 10 patients had complete remission with incomplete hematologic recovery and grade 4 pancytopenia. After haploidentical HSCT, 1 patient died on day 13 of septic shock ...
The work was supported by funding from the Ludwig Foundation for Cancer Research, the Emerson Collective Cancer Research Fund, the New York Stem Cell Foundation, the Stinehart-Reed Foundation, the Leukemia and Lymphoma Society, the J. Benjamin Eckenhoff Fund, the Blavatnik Family Fellowship, the Deutsche Forschungsgemainshaft, the Knut and ...
In women, breast cancer (BC) is the second most frequently diagnosed cancer and the leading cause of cancer death. Mesenchymal stem cells (MSCs) are a subgroup of heterogeneous non-hematopoietic fibroblast-like cells that have the ability to differentiate into multiple cell types. Recent studies stated that MSCs can migrate into the tumor sites and exert various effect on tumor growth and ...
The cancer stem cell (CSC) model, also called the deterministic model, emerged in the late 1990s when researchers began to address the possible relationship between hematopoietic stem cells and human leukemia [9,10]. It proposes that the growth and progression of numerous cancers are driven by small subpopulations of stem-like cells with self ...
Rockefeller University. "Cancer stem cells are fueled through dialogue with their environments." ScienceDaily. ScienceDaily, 30 November 2022. <www.sciencedaily.com / releases / 2022 / 11 ...
These cancer stem cells are believed to be capable of self-renewal and repopulation of tumor cells, resulting in the recurrence of cancer. The target of the new drug is an enzyme in cancer stem cells and tumor cells called Polo-like kinase 4, which was selected because blocking it negatively affects cell fate decisions associated with cancer ...
Two recent discoveries by stem cell scientists at Cedars-Sinai may help make cancer treatment more efficient and shorten the time it takes for people to recover from radiation and chemotherapy. In the first study, published in the journal Blood, investigators discovered a protein that is expressed by blood stem cells that could aid in ...
Many cancer therapies, in addition to producing numerous side effects, fail to achieve complete tumour remission, partly due to the presence of cancer stem cells, which are difficult to eradicate.
Isolation of cancer stem cells of the central nervous system (CNS) has been achieved by means of antigenic markers and by exploiting in vitro culture conditions developed for normal neural stem ...
July 26, 2021 — New research has identified potential treatment that could improve the human immune system's ability to search out and destroy cancer cells within the body. Scientists have ...
Like many areas of biomedical research, cancer stem cells are an evolving field of study. Multiple studies have indicated that insufficient evidence exists to confirm the existence of cancer stem cells. A review of 1,000 Web of Science publications revealed that only 49% supported the cancer stem cell hypothesis.
City of Hope to Present New Research at the American Society of Clinical Oncology (ASCO) Annual Meeting 2024, Highlighting Promising Data on Stem Cell Transplantation, Blood Cancers and Supportive ...
City of Hope to present new research at the American Society of Clinical Oncology (ASCO) Annual Meeting 2024, highlighting promising data on stem cell transplantation, blood cancers and supportive care oncology interventions. By. Zen Logsdon. May. 02, 2024. ... one of the largest cancer research and treatment organizations in the United States, ...
(SACRAMENTO) Neuroblastoma is the leading cause of cancer death in children under the age of five. Thanks to an award from The Hartwell Foundation, UC Davis pediatric surgeon Erin Brown will develop a stem cell treatment to target this difficult-to-treat disease.. Brown is one of only ten scientists selected to receive the 2024 Individual Biomedical Research Award.
The researchers had previously identified the presence of CD80 molecules in cancer stem cells and hypothesized that they played a role in the mechanism that allows cancer stem cells to evade the immune system. The team confirmed the role of CD80 during skin repair by removing it from the skin stem cells within the wounds of mice models.
Stem cell technologies may open new doors for cancer therapy. Stem cells migrate to solid tumors and micrometastatic lesions, facilitating site-specific anti-tumor agent delivery. ... However, conquering stem cell therapy limitations will require additional research to better illuminate relationships between normal and cancer stem cells. A ...