
- April 12, 2024 | Quantum Control Unlocked: Creating Resistance-Free Electron Channels
- April 12, 2024 | Shadow Play: NASA’s Best Photos of the Total Solar Eclipse
- April 12, 2024 | New Columbia Study Links Pregnancy With Accelerated Aging
- April 12, 2024 | Researchers Develop Simple Way To Harvest More “Blue Energy” From Waves
- April 12, 2024 | World-First Study Reveals That There Is up to 100x More Plastic on the Ocean Floor Than the Surface
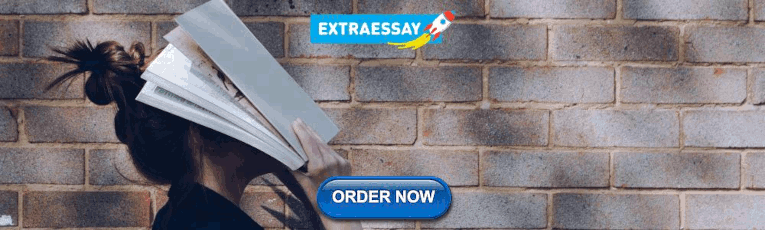
Rethinking H2O: Water Molecule Discovery Contradicts Textbook Models
By University of Cambridge January 15, 2024
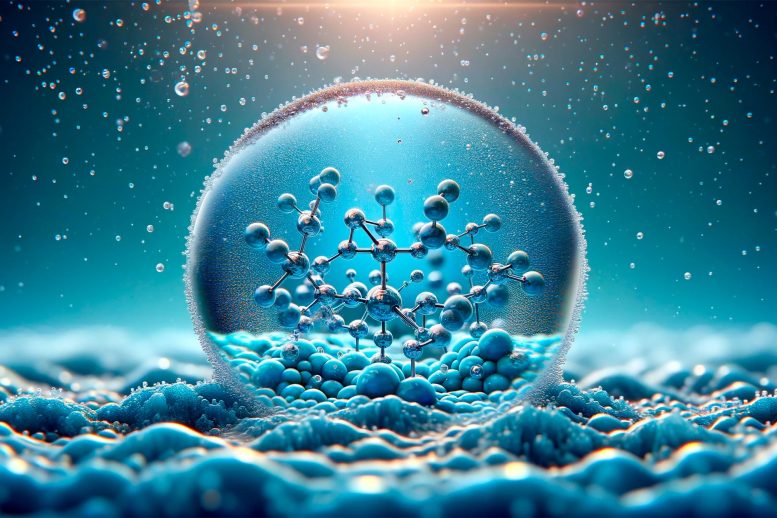
Researchers have overturned traditional models of how water molecules behave at the surface of saltwater, revealing new insights into ion distribution and orientation. This breakthrough, achieved through advanced techniques, has significant implications for climate science and technology. Credit: SciTechDaily.com
Groundbreaking research shows that water molecules at the saltwater surface behave differently than previously thought, offering new perspectives for environmental science and technology.
Textbook models will need to be re-drawn after a team of researchers found that water molecules at the surface of salt water are organized differently than previously thought.
Many important reactions related to climate and environmental processes take place where water molecules interface with air. For example, the evaporation of ocean water plays an important role in atmospheric chemistry and climate science. Understanding these reactions is crucial to efforts to mitigate the human effect on our planet.
The distribution of ions at the interface of air and water can affect atmospheric processes. However, a precise understanding of the microscopic reactions at these important interfaces has so far been intensely debated.
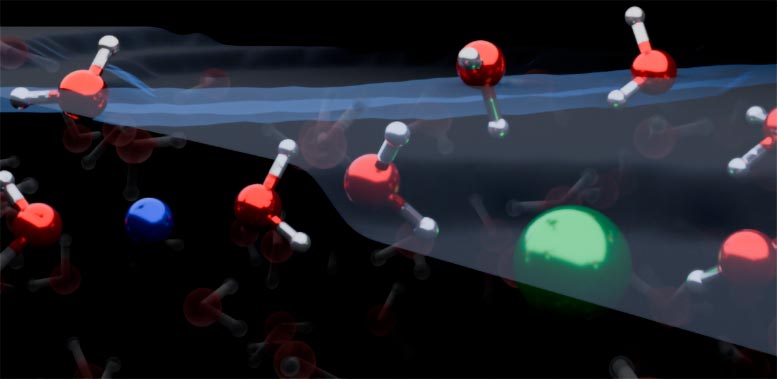
Graphic representation of the liquid/air interface in a sodium chloride solution. Credit: Yair Litman
Innovative Research Techniques
In a paper published today (January 15) in the journal Nature Chemistry , researchers from the University of Cambridge and the Max Planck Institute for Polymer Research in Germany show that ions and water molecules at the surface of most salt-water solutions, known as electrolyte solutions, are organized in a completely different way than traditionally understood. This could lead to better atmospheric chemistry models and other applications.
The researchers set out to study how water molecules are affected by the distribution of ions at the exact point where air and water meet. Traditionally, this has been done with a technique called vibrational sum-frequency generation (VSFG). With this laser radiation technique, it is possible to measure molecular vibrations directly at these key interfaces. However, although the strength of the signals can be measured, the technique does not measure whether the signals are positive or negative, which has made it difficult to interpret findings in the past. Additionally, using experimental data alone can give ambiguous results.
The team overcame these challenges by utilizing a more sophisticated form of VSFG, called heterodyne-detected (HD)-VSFG, to study different electrolyte solutions. They then developed advanced computer models to simulate the interfaces in different scenarios.
Revolutionizing Traditional Models
The combined results showed that both positively charged ions, called cations, and negatively charged ions, called anions, are depleted from the water/air interface. The cations and anions of simple electrolytes orient water molecules in both up- and down-orientation. This is a reversal of textbook models, which teach that ions form an electrical double layer and orient water molecules in only one direction.
Co-first author Dr Yair Litman, from the Yusuf Hamied Department of Chemistry, said: “Our work demonstrates that the surface of simple electrolyte solutions has a different ion distribution than previously thought and that the ion-enriched subsurface determines how the interface is organized: at the very top there are a few layers of pure water, then an ion-rich layer, then finally the bulk salt solution.”
Co-first author Dr Kuo-Yang Chiang of the Max Planck Institute said: “This paper shows that combining high-level HD-VSFG with simulations is an invaluable tool that will contribute to the molecular-level understanding of liquid interfaces.”
Professor Mischa Bonn, who heads the Molecular Spectroscopy department of the Max Planck Institute, added: “These types of interfaces occur everywhere on the planet, so studying them not only helps our fundamental understanding but can also lead to better devices and technologies. We are applying these same methods to study solid/liquid interfaces, which could have potential applications in batteries and energy storage.”
Reference: “Surface stratification determines the interfacial water structure of simple electrolyte solutions” by Yair Litman, Kuo-Yang Chiang, Takakazu Seki, Yuki Nagata and Mischa Bonn, 15 January 2024, Nature Chemistry . DOI: 10.1038/s41557-023-01416-6
More on SciTechDaily
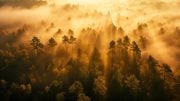
Carbon Sinks in Crisis: Climate Change Threatens Global Forest CO2 Sequestration
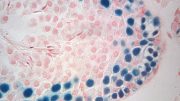
Frozen Testicular Tissue Can Be Reimplanted After 20 Years and Go On To Make Viable Sperm
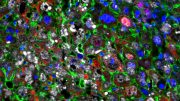
Damaged Liver Tissue Restored Faster Than Ever With Cellular Regeneration Therapy
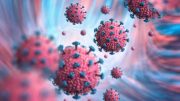
Beyond the Virus: The Devastating Long-Term Financial Toll of COVID-19
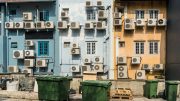
Cooling Homes Without Warming the Planet – New Technology for More Efficient AC
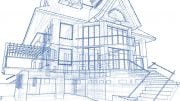
Just by Listening to Echoes, A Drone Can Determine the Shape of a Room
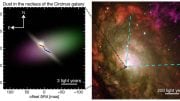
Astronomers View Dense Disk and Bright Funnel at the Center of the Circinus Galaxy
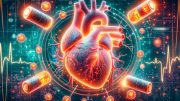
Why Your Heart Might Soon Heal Itself After a Heart Attack
2 comments on "rethinking h2o: water molecule discovery contradicts textbook models".
Different from … better than …
Hello, dears .Rethinking from the original, Rethinking then discussed shortly than Rethinking and decided that with the best possible solution afterwards open the lighting if people were interested to this there eyes will be lighting up and all of our will be not needs at this point
Leave a comment Cancel reply
Email address is optional. If provided, your email will not be published or shared.
Save my name, email, and website in this browser for the next time I comment.
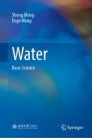
Water pp 21–40 Cite as
Understanding the Structure and Function of Water at the Molecular Scale
- Sheng Meng 3 &
- Enge Wang 4
- First Online: 20 June 2023
298 Accesses
If you had to choose “what is the most important substance in nature”, I’m afraid most people’s answer would be: water! This is probably one of the beliefs that have remained constant throughout the history of mankind. We have to ask: What is it about water and why is it so magical?
This is a preview of subscription content, log in via an institution .
Buying options
- Available as PDF
- Read on any device
- Instant download
- Own it forever
- Available as EPUB and PDF
- Durable hardcover edition
- Dispatched in 3 to 5 business days
- Free shipping worldwide - see info
Tax calculation will be finalised at checkout
Purchases are for personal use only
J.W. Döbereiner, Schweigg. J. 39 , 1 (1823)
Google Scholar
J.J. Berzelius, Jber. Chem. 15 , 237 (1837)
U. Raviv, P. Laurat, J. Klein, Fluidity of water confined to sub nanometer films. Nature 413 , 51 (2001)
Article ADS Google Scholar
D.D. Klug, Dense ice in detail. Nature 420 , 749 (2002)
O. Mishima, Y. Suzuki, Propagation of the polyamorphic transition of ice and the liquid-liquid critical point. Nature 419 , 599 (2002)
C.A. Tulk, C.J. Benmore, J. Urquidi, D.D. Klug, J. Neuefeind, B. Tomberli, P.A. Egelstaff, Structural studies of several distinct metastable forms of amorphous ice. Science 297 , 1320 (2002)
J. Li, D.K. Ross, Evidence for two kinds of hydrogen bond in ice. Nature 365 , 327 (1993)
W.L. Jorgensen, L. Salem, The Organic Chemists’ Book of Orbitals (Academic Press, New York, 1973)
T.R. Dyke, K.M. Mack, J.S. Muenter, The structure of water dimer from molecular beam electric resonance spectroscopy. J. Chem. Phys. 66 , 498 (1977)
N. Pugliano, R.J. Saykally, Measurement of quantum tunneling between chiral isomers of the cyclic water trimer. Science 257 , 193 (1992)
Article Google Scholar
J.D. Cruzan, L.B. Braly, K. Liu, M.G. Brown, J.G. Loeser, R.J. Saykally, Quantifying hydrogen bond cooperativity in water: VRT spectroscopy of the water tetramer. Science 271 , 59 (1996)
K. Liu, M.G. Brown, J.D. Cruzan, R.J. Saykally, Vibration-rotation tunneling spectra of the water pentamer: structure and dynamics. Science 271 , 62 (1996)
K. Liu, M.G. Brown, C. Carter, R.J. Saykally, J.K. Gregory, D.C. Clary, Characterization of a cage form of the water hexamer. Nature 381 , 501 (1996)
K. Liu, J.D. Cruzan, R.J. Saykally, Water clusters. Science 271 , 929 (1996)
J.K. Gregory, D.C. Clary, K. Liu, M.G. Brown, R.J. Saykally, The water dipole moment in water clusters. Science 275 , 814 (1997)
J.D. Bernal, R.H. Fowler, A theory of water and ionic solution, with particular reference to hydrogen and hydroxyl ions. J. Chem. Phys. 1 , 515 (1933)
L. Pauling, The structure and entropy of ice and of other crystals with some randomness of atomic arrangement. J. Am. Chem. Soc. 57 , 2680 (1935)
K. Modig, B.G. Pfrommer, B. Halle, Temperature-dependent hydrogen-bond geometry in liquid water. Phys. Rev. Lett. 90 , 075502 (2003)
M. Boero, K. Terakura, T. Ikeshoji, C.C. Liew, M. Parrinello, Hydrogen bonding and dipole moment of water at supercritical conditions: a first-principles molecular dynamics study. Phys. Rev. Lett. 85 , 3245 (2000)
E. Schwegler, G. Galli, F. Gygi, R.Q. Hood, Dissociation of water under pressure. Phys. Rev. Lett. 87 , 265501 (2001)
M.F. Kropman, H.J. Bakker, Dynamics of water molecules in aqueous solvation shells. Science 291 , 2118 (2001)
D. Marx, M.E. Tuckerman, J. Hutter, M. Parrinello, The nature of the hydrated excess proton in water. Nature 397 , 601 (1999)
M.E. Tuckerman, D. Marx, M. Parrinello, The nature and transport mechanism of hydrated hydroxide ions in aqueous solution. Nature 417 , 925 (2002)
J. Alper, Water splitting goes Au naturel. Science 299 , 1686 (2003)
F.L. Gervasio, P. Carloni, M. Parrinello, Electronic structure of wet DNA. Phys. Rev. Lett. 89 , 108102 (2002)
K. Laasonen, M. Sprik, M. Parrinello, “Ab initio” liquid water. J. Chem. Phys. 99 , 9080 (1993)
M.E. Tuckerman, D. Marx, M.L. Klein, M. Parrinello, On the quantum nature of the shared proton in hydrogen bonds. Science 275 , 817 (1997)
M. Benoit, D. Marx, M. Parrinello, Quantum effects on phase transitions in high-pressure ice. Comput. Mater. Sci. 10 , 88 (1998)
L.E. Firment, G.A. Somorjai, Low-energy electron diffraction studies of molecular crystals: the surface structures of vapor-grown ice and naphthalene. J. Chem. Phys. 63 , 1037 (1975)
L.E. Firment, G.A. Somorjai, Low energy electron diffraction studies of the surfaces of molecular crystals (ice, ammonia, naphthalene, benzene). Surf. Sci. 84 , 275 (1979)
B.A. Sexton, Vibrational spectra of water chemisorbed on Platinum (111). Surf. Sci. 94 , 435 (1980)
G.B. Fisher, J.L. Gland, The interaction of water with the Pt(111) surface. Surf. Sci. 94 , 446 (1980)
D. Doering, T.E. Madey, The adsorption of water on clean and oxygen-dosed Ru(001). Surf. Sci. 123 , 305 (1982)
P.A. Thiel, R.A. DePaola, F.M. Hoffmann, The vibrational spectra of chemisorbed molecular clusters: H 2 O on Ru(001). J. Chem. Phys. 80 , 5326 (1984)
S. Andersson, C. Nyberg, C.G. Tengstål, Adsorption of water monomers on Cu(100) and Pd(100) at low temperatures. Chem. Phys. Lett. 104 , 305 (1984)
F.T. Wagner, T.E. Moylan, A comparison between water adsorbed on Rh(111) and Pt(111), with and without predosed oxygen. Surf. Sci. 191 , 121 (1987)
W. Ranke, Low temperature adsorption and condensation of O 2 , H 2 O, and NO on Pt(111), studied by core level and valence band photoemission. Surf. Sci. 209 , 57 (1989)
A.F. Carley, P.R. Davies, M.W. Roberts, K.K. Thomas, Hydroxylation of molecularly adsorbed water at Ag(111) and Cu(100) surfaces by dioxygen: photoelectron and vibrational spectroscopic studies. Surf. Sci. 238 , L467 (1990)
H. Ogasawara, J. Yoshinobu, M. Kawai, Water adsorption on Pt(111): from isolated molecule to three-dimensional cluster. Chem. Phys. Lett. 231 , 188 (1994)
G. Held, D. Menzel, The structure of the p(√3×√3)R30° bilayer of D 2 O on Ru(001). Surf. Sci. 316 , 92 (1994)
M. Morgenstern, T. Michely, G. Comsa, Anisotropy in the adsorption of H 2 O at low coordination sites on Pt(111). Phys. Rev. Lett. 77 , 703 (1996)
M. Morgenstern, J. Muller, T. Michely, G. Comsa, The ice bilayer on Pt(111): nucleation, structure and melting. Z. Phys. Chem. 198 , 43 (1997)
I. Villegas, M.J. Weaver, Infrared spectroscopy of model electrochemical interfaces in ultrahigh vacuum: evidence for coupled cation-anion hydration in the Pt(111)/K + Cl − system. J. Phys. Chem. 100 , 19502 (1996)
S. Smith, C. Huang, E.K.L. Wong, B.D. Kay, Desorption and crystallization kinetics in nanoscale thin films of amorphous water ice. Surf. Sci. 367 , L13 (1996)
P. Löfgren, P. Ahlström, D.V. Chakarov, J. Lausmaa, B. Kasemo, Substrate dependent sublimation kinetics of mesoscopic ice films. Surf. Sci. 367 , L19 (1996)
A. Glebov, A.P. Graham, A. Menzel, J.P. Toennies, Orientational ordering of two-dimensional ice on Pt(111). J. Chem. Phys. 106 , 9382 (1997)
G. Pirug, H.P. Bonzel, UHV simulation of the electrochemical double layer: adsorption of HClO 4 /H 2 O on Au(111). Surf. Sci. 405 , 87 (1998)
X. Su, L. Lianos, Y.R. Shen, G.A. Somorjai, Surface-induced ferroelectric ice on Pt(111). Phys. Rev. Lett. 80 , 1533 (1998)
M.S. Yeganeh, S.M. Dougal, H.S. Pink, Vibrational spectroscopy of water at liquid/solid interfaces: crossing the isoelectric point of a solid surface. Phys. Rev. Lett. 83 , 1179 (1999)
H. Ogasawara, J. Yoshinobu, M. Kawai, Clustering behavior of water (D 2 O) on Pt(111). J. Chem. Phys. 111 , 7003 (1999)
M. Nakamura, Y. Shingaya, M. Ito, The vibrational spectra of water cluster molecules on Pt(111) surface at 20 K. Chem. Phys. Lett. 309 , 123 (1999)
A.L. Glebov, A.P. Graham, A. Menzel, Vibrational spectroscopy of water molecules on Pt(111) at submonolayer coverages. Surf. Sci. 427 , 22 (1999)
M. Nakamura, M. Ito, Monomer and tetramer water clusters adsorbed on Ru(0001). Chem. Phys. Lett. 325 , 293 (2000)
K. Jacobi, K. Bedürftig, Y. Wang, G. Ertl, From monomers to ice ─ new vibrational characteristics of H 2 O adsorbed on Pt(111). Surf. Sci. 472 , 9 (2001)
S. Haq, J. Harnett, A. Hodgson, Growth of thin crystalline ice films on Pt(111). Surf. Sci. 505 , 171 (2002)
T. Mitsui, M.K. Rose, E. Fomin, D.F. Ogletree, M. Salmeron, Water diffusion and clustering on Pd(111). Science 297 , 1850 (2002)
K. Morgenstern, J. Nieminen, Intermolecular bond length of ice on Ag(111). Phys. Rev. Lett. 88 , 066102 (2002)
H. Ogasawara, B. Brena, D. Nordlund, M. Nyberg, A. Pelmenschikov, L.G.M. Pettersson, A. Nilsson, Structure and bonding of water on Pt(111). Phys. Rev. Lett. 89 , 276102 (2002)
D.N. Denzler, C. Hess, R. Dudek, S. Wagner, C. Frischkorn, M. Wolf, G. Ertl, Interfacial structure of water on Ru(0001) investigated by vibrational spectroscopy. Chem. Phys. Lett. 376 , 618 (2003)
S.K. Jo, J. Kiss, J.A. Polanco, J.M. White, Identification of second layer adsorbates: water and chloroethane on Pt(111). Surf. Sci. 253 , 233 (1991)
P.J. Feibelman, Partial dissociation of water on Ru(0001). Science 295 , 99 (2002)
D.J. Elliot, Integrated Circuit Fabrication Technology (McGraw-Hill, New York, 1982)
W. Ranke, D. Schmeisser, Y.R. Xing, Orientation dependence of H 2 O adsorption on a cylindrical Si single crystal. Surf. Sci. 152–153 , 1103 (1985)
Y.J. Chabal, S.B. Christman, Evidence of dissociation of water on the Si(100)2×1 surface. Phys. Rev. B 29 , 6974 (1984)
R.A. Rosenberg, P.J. Love, V. Rehn, I. Owen, G. Thornton, The bonding of hydrogen on water-dosed Si(111). J. Vacuum Sci. Technol. A 4 , 1451 (1986)
F. Meyer, M.J. Sparnaay, The chemical and physical properties of clean Germanium and Silicon surfaces, in Surface physics of phosphors and semiconductors , ed. by C.G. Scott, C.E. Reed (Academic Press, London, 1975), chap. 6, pp. 321
R.H. Williams, I.T. McGovern, Adsorption on semiconductors, in the chemical physics of solid surfaces and heterogeneous catalysis, vol. 3, ed. by D.A. King, D.P. Woodruff (Elsevier, Amsterdam, 1984), chap. 6, pp. 267
W. Langel, M. Parrinello, Hydrolysis at stepped MgO surfaces. Phys. Rev. Lett. 73 , 504 (1994)
L. Giordano, J. Goniakowski, J. Suzanne, Partial dissociation of water molecules in the (3×2) water monolayer deposited on the MgO(100) surface. Phys. Rev. Lett. 81 , 1271 (1998)
M. Odelius, Mixed molecular and dissociative water adsorption on MgO[100]. Phys. Rev. Lett. 82 , 3919 (1999)
K.C. Hass, W.F. Schneider, A. Curioni, W. Andreoni, The chemistry of water on alumina surfaces: reaction dynamics from first principles. Science 282 , 265 (1998)
P.J.D. Lindan, N.M. Harrison, Mixed dissociative and molecular adsorption of water on the rutile (110) surface. Phys. Rev. Lett. 80 , 762 (1998)
A. Vittadini, A. Selloni, F.P. Rotzinger, M. Gratzel, Structure and energetics of water adsorbed at TiO 2 anatase (101) and (001) surfaces. Phys. Rev. Lett. 81 , 2954 (1998)
R. Schaub et al., Oxygen vacancies as active sites for water dissociation on rutile TiO 2 (110). Phys. Rev. Lett. 87 , 266104 (2001)
Y.S. Chu, T.E. Lister, W.G. Cullen, H. You, Z. Nagy, Commensurate water monolayer at the RuO 2 (110) water interface. Phys. Rev. Lett. 86 , 3364 (2001)
M. Odelius, M. Bernasconi, M. Parrinello, Two dimensional ice adsorbed on mica surface. Phys. Rev. Lett. 78 , 2855 (1997)
L. Cheng, P. Fenter, K.L. Nagy, M.L. Schlegel, N.C. Sturchio, Molecular-scale density oscillations in water adjacent to a mica surface. Phys. Rev. Lett. 87 , 156103 (2001)
S. Fölsch, M. Henzler, Water adsorption on the NaCl surface. Surf. Sci. 247 , 269 (1991)
S. Fölsch, A. Stock, M. Henzler, Two-dimensional water condensation on the NaCl(100) surface. Surf. Sci. 264 , 65 (1992)
L.W. Bruch, A. Glebov, J.P. Toennies, H. Weiss, A helium atom scattering study of water adsorption on the NaCl(100) single crystal surface. J. Chem. Phys. 103 , 5109 (1995)
M. Foster, G.E. Ewing, An infrared spectroscopic study of water thin films on NaCl(100). Surf. Sci. 427 , 102 (1999)
A. Allouche, Water adsorption on NaCl(100): a quantum ab-initio cluster calculation. Surf. Sci. 406 , 279 (1998)
H. Meyer, P. Entel, J. Hafner, Physisorption of water on salt surfaces. Surf. Sci. 488 , 177 (2001)
M.F. Reedijk, J. Arsic, F.F.A. Hollander, S.A. de Vries, E. Vlieg, Liquid order at the interface of KDP crystals with water: evidence for icelike layers. Phys. Rev. Lett. 90 , 066103 (2003)
D. Chakarov, B. Kasemo, Photoinduced crystallization of amorphous ice films on graphite. Phys. Rev. Lett. 81 , 5181 (1998)
T. Werder, J.H. Walther, R.L. Jaffe, T. Halicioglu, P. Koumoutsakos, On the water–carbon interaction for use in molecular dynamics simulations of graphite. J. Phys. Chem. B 107 , 1345 (2003)
L.F. Scatena, M.G. Brown, G.L. Richmond, Water at hydrophobic surfaces: weak hydrogen bonding and strong orientation effects. Science 292 , 908 (2001)
T.R. Jensen, M.O. Jensen, N. Reitzel, K. Balashev, G.H. Peters, K. Kjaer, T. Bjornholm, Water in contact with extended hydrophobic surfaces: direct evidence of weak dewetting. Phys. Rev. Lett. 90 , 086101 (2003)
L. Yang, H.W. Huang, Observation of a memberane fusion intermediate structure. Science 297 , 1877 (2002)
L. Guidoni, V. Torre, P. Carloni, Potassium and sodium binding to the outer mouth of the K + channel. Biochemistry 38 , 8599 (1999)
K. Murata et al., Structural determinants of water permeation through aquaporin-1. Nature 407 , 599 (2000)
P.A. Thiel, T.E. Madey, The interation of water with solid surfaces: fundamental aspects. Surf. Sci. Rep. 7 , 211 (1987)
M.A. Henderson, The interation of water with solid surfaces: fundamental aspects revisited. Surf. Sci. Rep. 46 , 1 (2002)
A. Hodgson, S. Haq, Water adsorption and the wetting of metal surfaces. Surf. Sci. Rep. 64 , 381 (2009)
A. Verdaguer, G.M. Sacha, H. Bluhm, M. Salmeron, Molecular structure of water at interfaces: wetting at the nanometer scale. Chem. Rev. 106 , 1478 (2006)
N.E. Levinger, Water in confinement. Science 298 , 1722 (2002)
P. Ball, How to keep dry in water. Nature 423 , 25 (2003)
J. Teixeira, J.M. Zanotti, M.C. BellissentFunel, S.H. Chen, Water in confined geometries. Phys. B 234 , 370 (1997)
R. Bergman, J. Swenson, Dynamics of supercooled water in confined geometry. Nature 403 , 283 (2000)
K. Koga, G.T. Gao, H. Tanaka, X.C. Zeng, Formation of ordered ice nanotubes inside carbon nanotubes. Nature 412 , 802 (2001)
G. Hummer, J.C. Rasaiah, J.P. Noworyta, Water conduction through the hydrophobic channel of a carbon nanotube. Nature 414 , 188 (2001)
M.S.P. Sansom, P.C. Biggin, Water at the nanoscale. Nature 414 , 156 (2001)
C. Dellago, M.M. Naor, G. Hummer, Proton transport through water-filled carbon nanotubes. Phys. Rev. Lett. 90 , 105902 (2003)
D.J. Mann, M.D. Halls, Water alignment and proton conduction inside carbon nanotubes. Phys. Rev. Lett. 90 , 195503 (2003)
R.P. Feynman, There is plenty of room at the bottom, in Annual Meeting of the American Physical Society . 29th Dec, 1959. http://www.zyvex.com/nanotech/feynman.html
Download references
Author information
Authors and affiliations.
Institute of Physics, Chinese Academy of Sciences, Beijing, China
Peking University, Beijing, China
You can also search for this author in PubMed Google Scholar
Corresponding author
Correspondence to Sheng Meng .
Rights and permissions
Reprints and permissions
Copyright information
© 2023 Peking University Press
About this chapter
Cite this chapter.
Meng, S., Wang, E. (2023). Understanding the Structure and Function of Water at the Molecular Scale. In: Water. Springer, Singapore. https://doi.org/10.1007/978-981-99-1541-5_2
Download citation
DOI : https://doi.org/10.1007/978-981-99-1541-5_2
Published : 20 June 2023
Publisher Name : Springer, Singapore
Print ISBN : 978-981-99-1540-8
Online ISBN : 978-981-99-1541-5
eBook Packages : Physics and Astronomy Physics and Astronomy (R0)
Share this chapter
Anyone you share the following link with will be able to read this content:
Sorry, a shareable link is not currently available for this article.
Provided by the Springer Nature SharedIt content-sharing initiative
- Publish with us
Policies and ethics
- Find a journal
- Track your research

An official website of the United States government
The .gov means it’s official. Federal government websites often end in .gov or .mil. Before sharing sensitive information, make sure you’re on a federal government site.
The site is secure. The https:// ensures that you are connecting to the official website and that any information you provide is encrypted and transmitted securely.
- Publications
- Account settings
Preview improvements coming to the PMC website in October 2024. Learn More or Try it out now .
- Advanced Search
- Journal List

A Novel Tool for Visualization of Water Molecular Structure and Its Changes, Expressed on the Scale of Temperature Influence
Zoltan kovacs.
1 Department of Physics and Control, Faculty of Food Science, Szent István University, H-1118 Budapest, Hungary
Bernhard Pollner
2 Department for Hygiene and Medical Microbiology, Medical University of Innsbruck, A-6020 Innsbruck, Austria; [email protected]
George Bazar
3 Department of Nutritional Science and Production Technology, Faculty of Agricultural and Environmental Sciences, Kaposvar University, H-7400 Kaposvar, Hungary; uh.balirga@razab
Jelena Muncan
4 Biomeasurement Technology Laboratory, Graduate School of Agricultural Science, Kobe University, Kobe 657-8501, Japan; pj.ca.u-ebok.elpoep@nacnumj
Roumiana Tsenkova
Aquaphotomics utilizes water-light interaction for in-depth exploration of water, its structure and role in aqueous and biologic systems. The aquagram, a major analytical tool of aquaphotomics, allows comparison of water molecular structures of different samples by comparing their respective absorbance spectral patterns. Temperature is the strongest perturbation of water changing almost all water species. To better interpret and understand spectral patterns, the objective of this work was to develop a novel, temperature-scaled aquagram that provides standardized information about changes in water molecular structure caused by solutes, with its effects translated to those which would have been caused by respective temperature changes. NIR spectra of Milli-Q water in the temperature range of 20–70 °C and aqueous solutions of potassium chloride in concentration range of 1 to 1000 mM were recorded to demonstrate the applicability of the proposed novel tool. The obtained results presented the influence of salt on the water molecular structure expressed as the equivalent effect of temperature in degrees of Celsius. The temperature-based aquagrams showed the well-known structure breaking and structure making effects of salts on water spectral pattern, for the first time presented in the terms of temperature influence on pure water. This new method enables comparison of spectral patterns providing a universal tool for evaluation of various bio-aqueous systems which can provide better insight into the system’s functionality.
1. Introduction
Recently, water has become more and more important subject of studies, as evidenced by the increasing number of water-related publications in various fields of science [ 1 , 2 , 3 , 4 ]. Water is fundamental to life—it is one of the essential and widely distributed components of biologic systems, which can be considered as a biomolecule in its own right [ 5 , 6 ]. Different scientific fields have been studying water from different aspects, trying to reach better understanding of its properties, structure and functions, yet still, our picture of water as a substance is rather incomplete. Spectroscopy methods, which are based on interaction of light and matter, have contributed a lot to our understanding of water. Recently, established, scientific discipline of aquaphotomics aims to integrate the knowledge these methods acquired about water based on its interaction with light, into one “omics” discipline which relates the structure of water with its functionality, and whose ultimate objective is better understanding of water as a matrix of aqueous and biologic systems [ 7 ]. In this regard, aquaphotomics have made significant novel discoveries, and utilized the properties of water in various application fields ranging from water and food quality monitoring, microbiology, to biomeasurements, biodiagnostics and biomonitoring [ 8 , 9 , 10 ]. Owing to the wide range of aqueous systems and biologic systems aquaphotomics studied, some novel, surprising insights have been discovered, which place focus on importance of water structure, hydrogen bonding and temperature.
The effect of temperature has always been one of the most studied phenomena in spectroscopy with respect to its influence on water structure. The very terms “structure-makers” and “structure-breakers” are coined to describe substances which induce changes in the water structure and consequently its spectrum, comparable to decrease in temperature and increase of temperature, respectively. Effects of salts or sugars are commonly described using those terms [ 11 , 12 , 13 ]. The known influence of temperature on hydrogen bonding in water is something that can be used as an etalon for better understanding of how substances affect the structure of water in various solutions. Among many others, principal component analysis and two-dimensional correlation spectroscopy [ 14 ] or multivariate curve resolution-alternating least squares technique [ 15 ] were used to describe the effects of temperature perturbations on the NIR spectra of water in terms of hydrogen bonding either alone or in comparison to salt perturbation.
Aquaphotomics studies made some further steps uncovering that various phenomena, related to biomolecules or functionality of living organisms and aqueous systems can be described as related to specific water molecular structure and presented using spectral pattern.
For example, in one work, concerned with the detection of UV-induced damage on DNA structure, it was found that the aqueous solutions of UVC-damaged DNA caused increase in hydrogen bonded water—i.e., that damaged DNA was a structure-making element causing changes of water similar to low temperature [ 16 ]. In another study, which explored cold tolerance ability of different soybean cultivars, it was found that the water structure in the leaves of those cultivars with higher cold tolerance ability even at low temperatures preserved the water in less-hydrogen bonded state compared to those cultivars who are more susceptible, as if the environmental temperature would have been in fact higher [ 17 ]. What these novel insights into the water structure and analogy with temperature influence provided is a novel knowledge and better understanding of the water functionality at different levels of organization of biologic systems.
One of the main visualization and analytical tools of aquaphotomics, is the so-called aquagram [ 8 , 18 ] which provides a comprehensible demonstration of the ratios of different water species present in a sample. Aquagrams have been found very useful in many applications, such as diagnosis of estrus in giant pandas [ 19 ], orangutans [ 20 ] and cows [ 21 ] and showing the different water spectral patterns of probiotic and non-probiotic bacteria strains [ 22 ], or for example, revealing different types of water in the soft contact lenses, in a completely nondestructive manner [ 23 , 24 , 25 ]. The cited references demonstrated the usefulness of the presentation of the water spectral patterns in aquagrams.
In order to unify the relation between water species depicted by aquagrams and related water functionalities we propose to use temperature as a common denominator to express changes of light absorbance at each of the water vibrational frequencies as changes caused by the most influential perturbation for water—temperature. Considering the advantages of better understanding of the functionality of water structure if the analogy is made with the influence of temperature, the objective of this work was to develop a novel method and a visualization tool, so called temperature-based aquagram which translates the effects of any type of perturbation of water structure in aqueous or biologic systems to the equivalent effects that would have been caused by the temperature changes and expressed in temperature units. The need to introduce the temperature-based aquagrams arose from the experiments on more complex systems, such as previously described, where it was observed that certain phenomena, (caused by solutes for example) have effects on the water molecular structure of the system and contribute to its functionality in the way analog to the changes in temperature.
In a study concerned with classification of bacteria based on the probiotic strength it was found that the strong probiotic bacteria, compared to the moderately strong strains or non-probiotic strains, create less hydrogen-bonded water species ( Figure 1 ) [ 22 ]. On the other hand, the spectra of pure water at different temperatures show shift of the main band towards the shorter wavelengths with increasing temperature [ 14 ], i.e., showing that increase in temperature results in breaking of the hydrogen bonds. If the two cases are compared, it can be seen that probiotic bacteria affect surrounding water similarly to the influence of temperature increase, as if they are a structure-breaking element [ 22 ]. This conclusion supports the novel insight into molecular mechanisms of how probiotics work–they increase solubility of substances in water [ 26 ], just like the increase in temperature of the water would contribute to better solubilization.

Water spectral pattern presented on aquagrams shows different water structure in different bacteria strains (Reprinted with permission from Slavchev, A., Kovacs, Z., Koshiba, H., Nagai, A., Bázár, G., Krastanov, A., Kubota, Y. and Tsenkova, R. 2015. [ 22 ]).
From this example, it can be seen that comparisons with effect of temperature can be useful and contribute to better intuitive understanding of the functionality of the studied system. Recalling the soybean cultivars example, the role of many substances that plants accumulate in response to cold stress, becomes more obvious—their function is to provide a certain water molecular structure in the leaves as if the temperature of the environment is different [ 17 , 27 ].
The concept of the novel visualization tool—a temperature-based aquagram—was developed similarly to the one Bernal and Fowler introduced long ago—the so called “structural temperature” concept [ 28 ]. The “structural temperature” is the respective temperature at which pure water would have effectively the same molecular structure as the water of the aqueous system under study. At the time of introduction, no adequate method that could describe that structure was found; there were propositions that it can be estimated based on the measurements of viscosity, Raman spectra or X-ray diffraction. In addition, the intended purpose was mainly concerned with the applications in analysis of electrolyte solutions, and since the concept did not offer the possibility to separate effects of the individual ions, the entire idea was more or less abandoned [ 29 ]. However, the structural temperature concept fits well into the framework of aquaphotomics, which places the water spectral pattern and the respective water functionality of the system in the central place. In contrast to “reductionistic omics methods” which are focused on isolating the biomolecules and separation of elements of the system, the aquaphotomics views each aqueous or biologic system as a whole, where all the components of the system exert their influence on the water matrix, whose structure is directly related to the function of the system [ 9 , 10 ]. Just like the temperature is the macroscopic, measurable characteristic, arising from the molecular structure of the system, so is the light absorbance at each water specific vibrational frequency, and one can benefit from expressing one in the terms of another.
The purpose of this study is to introduce a novel visualization tool, which expresses the effects of any type of perturbation of water molecular structure in aqueous or biologic systems to the equivalent effects that would have been caused by the temperature and expressed in temperature units. To illustrate this, we have chosen a simple salt solution and a concentration as a major perturbation. Salt is chosen as it is not near infrared active substance, hence the changes in absorbance of the solutions are only due to the changes in water molecular structure [ 15 , 30 ]. Following the steps provided in the study, one can easily replicate the experiment, develop the temperature-based aquagrams and use it further for specific purposes. While in this study, the analysis and the results are presented for only 1st overtone of water region, the same methodology is applicable for any region of the water absorbance spectra. This new method provides numerical results on a clearly defined scale with confidence intervals. It enables the comparison of results across time and different experiments and provides information about the statistical significance of the found differences.
2. Results and Discussion
The spectral data in the wavelength interval of 1300 to 1600 nm for the two experiments were separately subjected to principal component analysis (PCA). The PCA score plots demonstrated the multidimensional patterns of the spectral data. Specifically, the highest variations displayed in the first principal components (PC1) were related to temperature and concentration of potassium chloride, representing 99.3% and 99.1% of the spectral variation in case of the temperature and the potassium chloride experiments, respectively. The PCA results did not show outliers either in the temperature or the potassium chloride datasets. The raw and 2nd derivative spectra of the two experiments were analyzed separately to discover the wavelengths exhibiting the largest changes caused by the temperature and salt perturbations.
2.1. Results of Temperature Experiment
The raw and 2nd derivative absorbance (logT −1 ) spectra in the spectral range of 1300 to 1600 nm of Milli-Q water in the temperature range of 20 to 70 °C are shown in Figure 2 . The main feature of the NIR spectrum of water is a broad peak around 1450 nm, comprised of several overlapping bands, described mainly as the 1st overtone of the OH stretching vibration [ 31 ]. This observation is confirmed by the second derivative spectra which indicated very intense bands at 1412 and 1462 nm. These bands are well known as bands associated with free water molecules [ 32 , 33 , 34 ] and strongly hydrogen-bonded water [ 14 , 35 ], respectively.

Raw and 2nd derivative (calculated with Savitzky–Golay filter using 2nd order polynomial and 21 points) absorbance (logT −1 ) spectra in the spectral range of 1300–1600 nm (OH first overtone) of Milli-Q water in the temperature range of 20–70 °C ( n = 78).
From a spectroscopic point of view, an increase in temperature has been interpreted as a decrease of number of hydrogen bonds [ 14 , 34 ], while others explain it via weakening of hydrogen bonds [ 36 ]. There is agreement, regardless of one’s preferred theory, that the change of temperature causes alteration in hydrogen-bonding configurations of water as described in more details previously [ 37 ].
Figure 2 shows a “blue shift”, i.e., movement of the main band towards the shorter wavelengths with increasing temperature and an isosbestic (temperature invariant) point. These phenomena are well studied in scientific literature [ 14 , 34 , 38 ]. Evidence clearly suggest that less hydrogen-bonded water is predominant at higher temperature, while spectra acquired at low temperature are represented mostly in the more hydrogen-bonded area where water molecules form species with one (S1), two (S2), three (S3) and four (S4) hydrogen bonds [ 39 ].
Despite the competing theories about the water structure, aquaphotomics identifies water-specific absorbance bands with higher variations caused by respective perturbation for the system of interest and uses them to depict the unique spectral pattern as an integrated marker of the system–perturbation interaction. The presentation of the 12 specific water coordinates [ 7 ] of the spectra of Milli-Q water acquired in the temperature range of 20 to 70 °C together with the 95% confidence intervals is given in Figure 3 a,b, calculated with the temperature-based aquagram calculation method. In the case of working with more complex system, it would be advisable to follow the protocol of aquaphotomics analysis which can provide more thorough examination of activated water absorbance bands and not necessarily limit the presentation to only the 12 coordinates as is chosen here. The aquagram provides an easy-to-comprehend presentation of the phenomena described above, i.e., the change of the strongly and weakly hydrogen-bonded patterns, based on Figure 2 . The movement of the higher absorbance towards shorter wavelengths with increasing temperature is convincing. The scales of the aquagrams ( Figure 3 a,b) express the effect of perturbation occurring at the 12 coordinates (i.e., in the defined wavelength ranges) in degrees Celsius equivalent. Therefore, in this specific example the radial values show the temperatures corresponding to the signal acquisitions (20 to 70 °C). The plotted dashed and dotted lines represent the upper and lower confidence levels of the aquagram values, respectively, for each single analyzed temperature step. There was no overlapping of the confidence intervals (95%) of the Milli-Q samples measured at different temperature observed, meaning that 2 °C temperature changes caused statistically significant effects on the individual coordinates (i.e., in the defined wavelength ranges). The stability of the temperature-based aquagram calculation is presented in Figure 3 b, by depicting only three selected temperature levels (20, 30 and 40 °C). Though the spectral dataset used to calculate the temperature-based aquagram of Figure 3 b is only a subset of the dataset used to calculate Figure 3 a, the shape and the range are the same in both cases. This type of stability was not possible with the “classic” aquagram ( Figure 3 c,d). These examples present the applicability and the additional benefits of the newly developed temperature-based aquagram for the evaluation and presentation of the water species in the 12 coordinates (representing defined wavelength ranges) through the evaluation of a well-known perturbation, i.e., the effect of temperature on the water spectral changes.

Aquagrams of Milli-Q water in the temperature range of 20–70 °C, ( a , b ) with 95% confidence intervals calculated with temperature-based aquagram calculation method, ( c , d ) calculated with the classic calculation method, ( a , c ) all the 26 temperature steps ( n = 78) and ( b , d ) on three selected temperature steps ( n = 9) to show the stability of the methods (UCL—upper confidence level, LCL—lower confidence level).
2.2. Results of Potassium Chloride Experiment
The raw and 2nd derivative absorbance (logT −1 ) spectra of the aqueous solutions of 0.001 to 1 M potassium chloride salt in the 1300–1600 nm wavelength range (OH first overtone) are presented in Figure 4 . The main component of the aqueous solution, other than water, is KCl, which has no absorption in the NIR region; thus, it is not surprising that the spectra show a broad peak around 1450 nm. The second derivative spectra also provide similar patterns to those of the Milli-Q water acquired at different temperature, indicating bands at 1412 and 1462 nm. The trend of the shift in the peak position was similar to that observed in the temperature-perturbed pure water (i.e., the peak moves towards lower wavelengths as temperature is increased), but the actual peak locations were different from those for the pure water. The effect of low concentrations of salts diluted in water has been illustrated by the changes in the OH bonding of water molecular systems in many experiments [ 15 , 40 , 41 , 42 ]. As salts do not absorb NIR light, accurate measurement of even low concentration of salts, published in the above-mentioned papers, means salts change the surrounding water molecular structure according to the number of the solvent molecules in the solution which still can restructure the water molecular system.

Raw and 2nd derivative (calculated with Savitzky–Golay filter using 2nd order polynomial and 21 points) absorbance (logT −1 ) spectra in the range of 1300–1600 nm (OH first overtone) of 0.001–1 M KCl solutions ( n = 180).
This phenomena is not new; Bernal and Fowler [ 28 ] showed that the addition of electrolytes changes the spectrum of water in the infrared overtone region. Lin and Brown [ 43 ] also analyzed the effects of different salts on water spectra, and the authors were able to build accurate regression models using the spectral range of 1490 to 1610 nm for salt content prediction.
The spectra shown in Figure 4 imply that the increasing concentration of KCl causes “blue shift”, i.e., a shift towards the spectral range referring to the band of less hydrogen-bonded water molecules. Our findings, hence show a good agreement with the results of previous research [ 15 , 40 , 41 , 42 ].
A more detailed evaluation of the proposed 12 specific water coordinates [ 7 ] of the spectra of the aqueous solutions of potassium chloride compared to the spectra of water is provided in Figure 5 . The plots show the aquagrams of the single concentration levels together with the respective 95% confidence intervals calculated with the “classic” method ( Figure 5 a) and the new temperature-based aquagram calculation method ( Figure 5 b). The relative position of the patterns of the single concentration levels shows some similarity for the two methods. Furthermore, in both methods, spectral patterns of the highest concentration range (100–1000 mM) show the highest difference from the spectra of the lower ranges and Milli-Q samples (the latter one in black). This pattern explains the same phenomena which were found based on the raw spectra, i.e., a higher concentration of salt create water with less hydrogen bonds and decreases the number of water species to more hydrogen bonds. For the higher concentration ranges the aquagrams of the individual concentrations do not overlap, i.e., addition of 0.01 or 0.1 M KCl caused statistically significant change at the individual coordinates.

Aquagrams with 95% confidence intervals of Milli-Q water ( n = 150) and 0.001–1 M KCl solutions ( n = 180) calculated with the classic ( a ) or the temperature based aquagram ( b ) calculation methods on the individual concentrations (UCL—upper confidence level, LCL—lower confidence level).
However, the results also show an increasing tendency of the C7 coordinate (1432–1444 nm) with increasing concentration of KCl, which can be assigned to water molecules with one hydrogen bond (S1), meaning that increase in concentration of salt leads to increase in the number of these molecules [ 39 ].
The dominantly higher effect of the higher concentrations on the spectral pattern hides the pattern of the lower concentrations; therefore, the calculation of the aquagrams were performed using the spectral data set for the two lowest concentration ranges only ( Figure 6 ). The phenomena of the higher concentrations on the water spectral pattern, namely the higher concentration being more dominantly a structure breaker, can be observed in case of the concentration of samples at the concentration range of 10 to 100 mM. It is interesting to note the similarities to the pattern of the aquagrams calculated on all the three ranges ( Figure 5 b).

Aquagrams with 95% confidence intervals of Milli-Q water ( n = 150) and 0.001–0.1 M KCl solutions ( n = 120) calculated with the temperature based aquagram calculation method on the individual concentrations (UCL—upper confidence level, LCL—lower confidence level).
The aquagrams of the higher concentrations (10–100 mM) show consistency with the previous findings regarding the structure-breaking characteristic as displayed in Figure 6 . More specifically, the higher concentration samples present higher absorbance values in the range between 1342 and 1374 nm, i.e., C01-C03 that refer to the 1st overtone of free OH stretch (OH–(H 2 O) n , n = 1…4) [ 44 , 45 ] and 1440 and 1452 nm, i.e., C07-C08 that are known as the bands of water hydration [ 35 ] and water molecule connected to another water molecule (S 1 ) [ 14 , 46 ] and the symmetric and asymmetric stretching of first overtone of water [ 35 , 46 , 47 ]. However, in the range between 1476 and 1512 nm, i.e., C10-C12, the higher concentration samples show lower values and these wavelengths are usually assigned to strongly hydrogen bonded water [ 14 , 35 ] and aqueous protons ([H + •(H 2 O) 6 ]–H 2 O in H 5 O 2 + symmetric stretch) [ 48 ]. These findings also mean decreasing concentration of salt causes a shift towards longer wavelengths, i.e., the range referring to the band of more hydrogen bonded water molecules.
Using the additional benefit of the temperature-based aquagram calculation method, further results can be achieved from Figure 6 . The addition of, for instance, 0.1 M potassium chloride to Milli-Q water results in water structural changes equivalent to changes caused by temperature of about 0.65, 0.6, 0.3, 0.1, 0.2 0.6, 1.8, 1.2, 0.2, −0.1, −0.3 and −0.6 °C at C01, C02, C03, C04, C05, C06, C07, C08, C09, C10, C11 and C12 coordinates, respectively. Furthermore, having calculated the confidence intervals, the statistical significance of the differences is also available. For example, calculations showed that addition of 0.02 M KCl to Milli-Q caused change equivalent to the change due to 0.33 and 0.23 °C temperature increase at coordinates C07 and C08 when compared to pure Milli-Q, which was found significant ( p = 0.05), too.
The aquagrams of the lowest concentration range (1–10 mM) calculated with the temperature-based aquagram method is shown in Figure 7 to further evaluate the findings of the effects of decreasing concentrations of salt. The results suggest that we can observe concentration levels of 5 to 10 mM that cause a shift towards longer wavelengths, i.e., the range referring to the band of more hydrogen-bonded water molecules compared to the spectrum of Milli-Q water. These findings imply that salts can also have structure-breaker and structure-maker effects on water structure similar to the behavior of sugars [ 13 ]. However, the aquagrams of the even lower concentrations (1 to 5 mM) also show alteration with nearly the same deviation, but towards shorter wavelengths. This may suggest that changing between structure-breaker and structure-maker properties of salt exist at such a low concentration, but the discernment of these minor changes would require very accurate measurements.

Aquagrams with 95% confidence intervals of Milli-Q water ( n = 150) and 0.001–0.01 M KCl solutions ( n = 60) calculated with the temperature based aquagram calculation method on the individual concentrations (UCL—upper confidence level, LCL—lower confidence level).
3. Materials and Methods
3.1. samples.
Two experiments with different sources of perturbations were conducted to demonstrate the procedure of temperature-based aquagram development and show the advantages in comparison to the representation of spectral data using classic aquagrams. In both experiments, pure Milli-Q water was used as a sample (Milli-Q purification system (Millipore, Molsheim, France, resistance = 18 MΩ) and in the first experiment perturbation of the sample was caused by changes in temperature, while in the second one, by changes in the concentration of salt.
3.2. The Temperature Experiment
The effect of temperature perturbation on water near-infrared spectra has been well-studied and is thoroughly described in the literature [ 14 , 34 , 49 ]. Therefore, the experiment was performed on Milli-Q water in the temperature range of 20 to 70 °C to acquire spectra which could be used for the evaluation of the aquagram methods. The Milli-Q water was produced by a Milli-Q purification system (Millipore, Molsheim, France, resistance = 18 MΩ). The spectral acquisition was performed at 2 °C increments, resulting in 26 temperature steps in the range of 20 to 70 °C.
3.3. The Potassium Chloride Experiment
The addition of salt to water at different concentrations is also an often evaluated perturbation [ 42 , 50 ]. Therefore, an experiment was performed with different concentrations of aqueous solutions of potassium chloride. Potassium chloride (KCl, M = 74.56 g mol −1 , purity min. 99.0% mass/mass) was purchased from Wako Pure Chemical Industries, Ltd. (Kobe, Japan). Aqueous solutions were prepared in different concentrations of KCl, in the range of 1 to 1000 mM. Three concentration ranges were prepared: Range A, from 100 to 1000 mM concentration, in steps of 100 mM; Range B from 10 to 100 mM, in steps of 10 mM and finally, Range C, in 1 to 10 mM in 1 mM concentration steps. Each dilution was prepared by serial dilution from the stock samples with the highest concentration in a given range (A, B or C) and prepared in two replicates, resulting in two independently prepared sets of samples. Stock solutions were prepared and further serially diluted with added Milli-Q water step-by-step to reach the appropriate concentrations—a solution created in each step was further diluted to prepare the next lower concentration.
3.4. NIR Spectral Acquisition
A FOSS-XDS spectrometer (FOSS NIRSystems, Inc., Hoganas, Sweden) equipped with a Rapid Liquid Analyzer module including a temperature-controlled 1 mm pathlength cuvette holder was used to measure transmittance spectra (logT −1 ) of the Milli-Q samples for the temperature experiment and of the aqueous solutions for the KCl experiment. Spectral acquisition was performed by saving three consecutive spectra in the range of 400–2500 nm at 0.5 nm spectral steps. Each saved spectrum was the average of 32 successive scans.
A thermal bath with continuous water circulation was attached to the Rapid Liquid Analyzer module to ensure the required temperature of the sample during scanning in the range of 20 to 70 °C at 2 °C increments.
The same apparatus was used to provide a constant temperature of 28 °C where each aqueous solution of potassium chloride was incubated for 90 s to equilibrate to the required temperature before scanning. Milli-Q water samples were measured as every fifth sample during the KCl experiment to provide environmental controls.
The total number of spectra for the temperature experiment was 78 (26 temperature steps × 3 consecutive scans) and for the KCl experiment was 330 (30 concentrations × 2 repeats × 3 consecutive scans + 150 Milli-Q control scans) ( Appendix A Dataset).
The FOSS-XDS instrument was operated using VISION 3.5 software (FOSS NIRSystems, Inc., Hoganas, Sweden). In both experiments, reference spectra were recorded before every sample.
3.5. Statistical Data Analysis
Only the wavelength interval of 1300 to 1600 nm, corresponding to the first overtone of the O–H stretching band [ 51 ] was used for the evaluations. For the purpose of explaining methodology of how to develop temperature-based aquagrams, in this study, the focus is placed on this particular part of the water absorbance spectra, because it is best understood so far in the terms of the water molecular species whose absorbance bands are well-resolved and their assignments known [ 7 ]. In the first overtone of water as a result of systematization of experimental work done on many different systems, not only different aqueous solutions, but also a great variety of biologic systems under different perturbations 12 water absorbance bands termed WAMACs (Water Matrix Coordinates)–each range from 6 to 12 nm width, were discovered [ 7 ]. The great body of evidences in scientific literature provided the meaning to these ranges—i.e., it was possible to connect each of these 12 coordinates to specific water molecular species. In aquaphotomics studies the 12 WAMACs are called, coordinates because they represent windows in the spectra through which water structure of the system under study can be observed. In this study, the 12 WAMACs will be used to develop aquagrams, but it should be noted that the methodology explained is applicable for any region of the water absorbance spectra; it is not necessarily limited to the coordinates chosen here. Here, we chose for the reason of simplicity and because they are well-understood to use only those. However, depending on the system under study and the range of the spectra used, the reader is advised to follow the aquaphotomics protocol of analysis for extraction of the “activated water absorbance bands–WAMACs [ 8 ] and then follow the further instructions to develop temperature-based aquagrams as described below.
Before the development of aquagrams, exploratory analysis was performed. First, Principal component analysis (PCA) [ 52 ] was used to describe multidimensional patterns in the spectral data and to discover outliers. The raw and 2nd derivative spectra were plotted to visualize the spectral changes induced by temperature perturbation and by the perturbation of salt concentration on the spectra of Milli-Q water and aqueous solutions of potassium chloride, respectively. The 2nd derivative spectra were calculated using a Savitzky–Golay filter [ 53 ] using the 2nd order polynomial and 21 points.
3.5.1. Calculation Protocol of “Classic” Aquagram
The absorbance values at specific water matrix coordinates (WAMACs) [ 7 ] define the water spectral pattern (WASP), which is different for different perturbations. The WASP can be visualized by a chart called the aquagram [ 18 , 19 ]. This representation of the WAMACs was first introduced by Tsenkova [ 18 ]. This aquagram (from now on called the “classic” aquagram) displays the multiplicative-scatter-corrected (MSC) (or standard-normal-variate (SNV)) transformed, normalized and averaged absorbance values of different samples or sample groups at 12 specific characteristic wavelengths. As, mentioned, these specific wavelengths were experimentally discovered as absorbance bands of specific water molecular species in previous studies and are later confirmed by overtone calculations of already reported water absorbance bands in the infrared range [ 7 ]. The aforementioned water absorbance bands cover various form of water molecular species and are thus useful to depict characteristic spectral patterns in the first overtone region of water. This calculation can be summarized by Equation (1).
where, A λ ′ —value on aquagram for a given wavelength; A λ —absorbance after MSC applied on 1st overtone region of OH (i.e., 1300–1600 nm); μ λ —mean of all spectra for the examined group at a given wavelength (after MSC applied); σ λ —SD of all spectra for the examined group at a given wavelength (after MSC applied); λ —12 wavelengths (1342, 1364, 1374, 1384, 1412, 1426, 1440, 1452, 1462, 1476, 1488, 1512 nm) [ 7 ].
The classic aquagram shows the relative fingerprint, i.e., the WASPs, in the context of all spectra in the examined group [ 18 , 19 , 21 , 54 ].
Recently, this aquagram calculation method was extended by adding the possibility to observe the statistical significance of the differences presented on the aquagrams. Therefore, besides the average spectra of the individual groups used to plot the aquagrams, the respective confidence intervals are also calculated using the so-called Bootstrap method [ 55 ]. This improvement makes it possible to plot the aquagrams together with their upper and lower 95% confidence interval limits.
3.5.2. Calculation Protocol for Newly Developed (Temperature-Based) Aquagram
The newly developed temperature-based aquagram calculation algorithm presents the respective water matrix coordinates in units equivalent to change in temperature and includes the respective confidence intervals. Therefore, it gives rise to the possibility to compare the WAMACs across time and also across different experiments and it provides information about the statistical significance of the differences.
The new calculation method is based on the comparison of the areas (under the ranges of the above-mentioned 12 specific water coordinates [ 7 ]) of the respective test sample spectra and the spectra of Milli-Q water. This method aims to express the spectral pattern changes in units equivalent to the change of temperature that would cause the observed change. The calculation of this aquagram concept (from now called the temperature-based aquagram) can be summarized in the following steps. Note that the calculation steps are explained for one coordinate (C01 representing spectral range between 1336 and 1348 nm, as an example) out of the 12 coordinates (C01-C12, i.e., defined wavelength ranges) to give an easily understandable description, but the same steps have to be repeated for each of the 12 coordinates. The main calculation steps are summarized in a chart to provide an overview of the developed method ( Appendix B Figure A1 ).
The dataset of the experiment of interest is defined as the experimental dataset.
The average spectra of the groups of interest (in this case, salt concentration levels) are calculated in the experimental dataset together with their respective confidence intervals using the Bootstrap method [ 55 ], yielding as many single, unique spectra as there are groups are in the experimental dataset (plus their upper and lower 95% confidence interval limits).

Scheme of the calculation for Area under the curve (AUC) aquagram method. Spectrum of pure water with highlighted subranges of the 12 specific water matrix coordinates (WAMACs).
- Step 4. The ratio of the area under the curve for each single coordinate is calculated with respect to the full area under the curve for the first overtone OH region (i.e., the area of C01 is divided by the full area under the spectrum in the range of 1300 to 1600 nm). This is done for every single average spectrum, in the reference dataset and the experimental dataset (together with the respective confidence interval limits for the experimental dataset). This calculation step provides normalized values and avoids possible differences due to scattering and/or pathlength effects.
- Step 5. Based on the reference dataset, a continuous array of values for the relative area of C01 (as calculated in Step 1) is calculated for a continuous temperature range from 20 to 70 °C using local polynomial regression. This is an essential step in order to accommodate the data from an experiment performed at specific temperature—see Step 6.
- Step 6. The basic principle of the temperature-based aquagram method is to compare the effect of the perturbation used on the system under study which resulted in a certain water spectral pattern to the effect the temperature changes would induce in pure water. Thus, any perturbation can be expressed as an equivalent temperature effect on a Milli-Q water sample. It is necessary to perform a “local calibration” with the reference dataset around the temperature of the experimental dataset. Therefore, in this step, the temperature calibration range is defined. This range is used to express the effect of perturbation in degrees Celsius equivalent. For this, a symmetrical scale is defined from the reference dataset (calculated at Step 5) using two degrees, plus and minus around the temperature of the experiment (hence, a span of 4 °C). For example, if the experiment was performed at 25.0 °C, then the calibration range of 23.0 to 27.0 °C would be used.
- Step 7. The temperature calibration equation, the relationship between the change of the temperature and change of the area of C01 at the temperature of the experiment, is determined based on the calculation performed in Step 5 on the reference dataset. (It is known how the area of C01 changes as a function of temperature described by a linear function). Therefore, it is easy to compare the changes for areas for C01 for the experimental dataset (calculated at Step 3) to the changes of the area of C01 caused by temperature, i.e., to express the changes in C01 in units of temperature (degrees Celsius) equivalent.
- Step 8. The calculated temperature (degrees Celsius) equivalent value for every group of the experimental dataset is finally visualized together with the respective 95% confidence intervals in a radar chart, where the units of the axes are in degrees Celsius.
- Step 9. The calculation and visualization of the results were performed using the R programing language [ 56 , 57 ].
4. Conclusions
Recently, aquaphotomics has been introduced to focus on water as a key component for providing information about the function of the entire system. Non-destructive NIR spectroscopy and aquaphotomics have been applied to explain new phenomena in the field of life sciences. In contrast to reductionistic methods in which biomolecules and other elements are analyzed separately from the system, aquaphotomics studies the aqueous systems through its water matrix.
In the present study, a newly developed temperature-based aquagram calculation method is presented as an additional tool to express the changes of water molecular structure in aqueous systems which are caused by perturbations different from temperature. Although the need to introduce temperature-based aquagrams originated from experiments on complex systems, the successful application of the new aquagram calculation method was demonstrated through the evaluation of the results of well-known phenomena. The results of temperature and salt perturbations on Milli-Q water are demonstrated in the present study.
The effect of temperature on the spectral pattern of Milli-Q water acquired in the temperature range of 20 to 70 °C is presented with the temperature-based aquagram calculation method. The method provides the presentation of the phenomena demonstrating blue shift in the first OH overtone range with increasing temperature. Furthermore, the scale of the aquagram expressed the effect of perturbation in degrees Celsius equivalent.
Aqueous solutions of potassium chloride were chosen for the experiment as KCl has no absorption in the NIR region. Thus, its effect on water spectral patterns can be clearly evaluated and presented as caused by temperature changes at respective wavelengths. Furthermore, it demonstrates that the method is invariably applicable for evaluation the effects of all types of solutes. The new temperature-based aquagram calculation method provided further information about the magnitude of the change. In other words, the results were displayed on a degree-Celsius scale that showed how much a given sample would have needed to have been warmed up or cooled down in each of the single coordinates (C01 to C12) to achieve the same results as the actual measurement, while all measurements were performed at precisely the same temperature.
Adding 0.1-M potassium chloride to Milli-Q water resulted in structural changes equivalent to an approximately 0.6 °C temperature increase in the less hydrogen-bonded, and 0.3 °C temperature decrease in the more hydrogen-bonded areas of the OH first overtone spectral region.
The examples presented here confirm the applicability and the additional benefits of the temperature-based aquagram calculation method. They provide a demonstration of the ratio of the different water species existing in different aqueous and biologic systems. Additionally, this new type of aquagram calculation displayed the spectral patterns in a meaningful scale and stable pattern independent of any modification of the evaluated dataset, which gives rise to the opportunity to compare results not only within a single chart, but also across time and different experiments.
This newly developed tool is especially suitable for visualizing water structure evolution and phase transitions, for example, in the food preservation industry, pharmaceutical development, material science and related applications.
The presented new chemometric tool, developed in R-Project, is freely accessible as an R-package from GitHub repository [ 58 ] and can be used in various fields of NIR spectroscopy and water research.
Acknowledgments
Authors are grateful to Professor David Funk for his help to revise the manuscript from language editing and from scientific point of view.
Dataset. Raw spectral data used for the calculations presented in this study. Both data of temperature experiment and potassium chloride experiment is included in this dataset where the column experiment describes which spectra belong to which experiment.

Workflow of the calculation protocol of temperature-based aquagram.
Author Contributions
All authors contributed to this research. The design of the experiments was performed by Z.K., B.P. and R.T., Z.K. and B.P. did the recording and the processing of the NIRS data, as well as the result of the evaluation and implementing the concept in R-project. The manuscript was written by Z.K., B.P., G.B. and J.M., R.T. assisted in writing the study and revised it. Z.K., R.T., G.B. and J.M. contributed to designing the research and revise the manuscript. The work presented in the study was conceived within research projects led by R.T. and Z.K. All authors have read and agreed to the published version of the manuscript.
Authors acknowledge the financial support of the ÚNKP-19–4 New National Excellence Program of the Ministry for Innovation and Technology (Z.K.), János Bolyai Research Scholarship of the Hungarian Academy of Sciences (Z.K.) and by the European Union and co-financed by the European Social Fund through project No. EFOP-3.6.3-VEKOP-16–2017–00005. JM gratefully acknowledges the financial support provided by Japanese Society for Promotion of Science ( {"type":"entrez-protein","attrs":{"text":"P17406","term_id":"114259","term_text":"P17406"}} P17406 ).
Conflicts of Interest
The authors declare no conflict of interest.
Sample Availability: Samples of the compounds KCl are available from the authors.
- Search Menu
- Browse content in Arts and Humanities
- Browse content in Archaeology
- Anglo-Saxon and Medieval Archaeology
- Archaeological Methodology and Techniques
- Archaeology by Region
- Archaeology of Religion
- Archaeology of Trade and Exchange
- Biblical Archaeology
- Contemporary and Public Archaeology
- Environmental Archaeology
- Historical Archaeology
- History and Theory of Archaeology
- Industrial Archaeology
- Landscape Archaeology
- Mortuary Archaeology
- Prehistoric Archaeology
- Underwater Archaeology
- Urban Archaeology
- Zooarchaeology
- Browse content in Architecture
- Architectural Structure and Design
- History of Architecture
- Residential and Domestic Buildings
- Theory of Architecture
- Browse content in Art
- Art Subjects and Themes
- History of Art
- Industrial and Commercial Art
- Theory of Art
- Biographical Studies
- Byzantine Studies
- Browse content in Classical Studies
- Classical Literature
- Classical Reception
- Classical History
- Classical Philosophy
- Classical Mythology
- Classical Art and Architecture
- Classical Oratory and Rhetoric
- Greek and Roman Archaeology
- Greek and Roman Epigraphy
- Greek and Roman Law
- Greek and Roman Papyrology
- Late Antiquity
- Religion in the Ancient World
- Digital Humanities
- Browse content in History
- Colonialism and Imperialism
- Diplomatic History
- Environmental History
- Genealogy, Heraldry, Names, and Honours
- Genocide and Ethnic Cleansing
- Historical Geography
- History by Period
- History of Agriculture
- History of Education
- History of Emotions
- History of Gender and Sexuality
- Industrial History
- Intellectual History
- International History
- Labour History
- Legal and Constitutional History
- Local and Family History
- Maritime History
- Military History
- National Liberation and Post-Colonialism
- Oral History
- Political History
- Public History
- Regional and National History
- Revolutions and Rebellions
- Slavery and Abolition of Slavery
- Social and Cultural History
- Theory, Methods, and Historiography
- Urban History
- World History
- Browse content in Language Teaching and Learning
- Language Learning (Specific Skills)
- Language Teaching Theory and Methods
- Browse content in Linguistics
- Applied Linguistics
- Cognitive Linguistics
- Computational Linguistics
- Forensic Linguistics
- Grammar, Syntax and Morphology
- Historical and Diachronic Linguistics
- History of English
- Language Variation
- Language Families
- Language Acquisition
- Language Evolution
- Language Reference
- Lexicography
- Linguistic Theories
- Linguistic Typology
- Linguistic Anthropology
- Phonetics and Phonology
- Psycholinguistics
- Sociolinguistics
- Translation and Interpretation
- Writing Systems
- Browse content in Literature
- Bibliography
- Children's Literature Studies
- Literary Studies (Modernism)
- Literary Studies (Asian)
- Literary Studies (European)
- Literary Studies (Eco-criticism)
- Literary Studies (Romanticism)
- Literary Studies (American)
- Literary Studies - World
- Literary Studies (1500 to 1800)
- Literary Studies (19th Century)
- Literary Studies (20th Century onwards)
- Literary Studies (African American Literature)
- Literary Studies (British and Irish)
- Literary Studies (Early and Medieval)
- Literary Studies (Fiction, Novelists, and Prose Writers)
- Literary Studies (Gender Studies)
- Literary Studies (Graphic Novels)
- Literary Studies (History of the Book)
- Literary Studies (Plays and Playwrights)
- Literary Studies (Poetry and Poets)
- Literary Studies (Postcolonial Literature)
- Literary Studies (Queer Studies)
- Literary Studies (Science Fiction)
- Literary Studies (Travel Literature)
- Literary Studies (War Literature)
- Literary Studies (Women's Writing)
- Literary Theory and Cultural Studies
- Mythology and Folklore
- Shakespeare Studies and Criticism
- Browse content in Media Studies
- Browse content in Music
- Applied Music
- Dance and Music
- Ethics in Music
- Ethnomusicology
- Gender and Sexuality in Music
- Medicine and Music
- Music Cultures
- Music and Culture
- Music and Religion
- Music and Media
- Music Education and Pedagogy
- Music Theory and Analysis
- Musical Scores, Lyrics, and Libretti
- Musical Structures, Styles, and Techniques
- Musicology and Music History
- Performance Practice and Studies
- Race and Ethnicity in Music
- Sound Studies
- Browse content in Performing Arts
- Browse content in Philosophy
- Aesthetics and Philosophy of Art
- Epistemology
- Feminist Philosophy
- History of Western Philosophy
- Metaphysics
- Moral Philosophy
- Non-Western Philosophy
- Philosophy of Action
- Philosophy of Law
- Philosophy of Religion
- Philosophy of Science
- Philosophy of Language
- Philosophy of Mind
- Philosophy of Perception
- Philosophy of Mathematics and Logic
- Practical Ethics
- Social and Political Philosophy
- Browse content in Religion
- Biblical Studies
- Christianity
- East Asian Religions
- History of Religion
- Judaism and Jewish Studies
- Qumran Studies
- Religion and Education
- Religion and Health
- Religion and Politics
- Religion and Science
- Religion and Law
- Religion and Art, Literature, and Music
- Religious Studies
- Browse content in Society and Culture
- Cookery, Food, and Drink
- Cultural Studies
- Customs and Traditions
- Ethical Issues and Debates
- Hobbies, Games, Arts and Crafts
- Lifestyle, Home, and Garden
- Natural world, Country Life, and Pets
- Popular Beliefs and Controversial Knowledge
- Sports and Outdoor Recreation
- Technology and Society
- Travel and Holiday
- Visual Culture
- Browse content in Law
- Arbitration
- Browse content in Company and Commercial Law
- Commercial Law
- Company Law
- Browse content in Comparative Law
- Systems of Law
- Competition Law
- Browse content in Constitutional and Administrative Law
- Government Powers
- Judicial Review
- Local Government Law
- Military and Defence Law
- Parliamentary and Legislative Practice
- Construction Law
- Contract Law
- Browse content in Criminal Law
- Criminal Procedure
- Criminal Evidence Law
- Sentencing and Punishment
- Employment and Labour Law
- Environment and Energy Law
- Browse content in Financial Law
- Banking Law
- Insolvency Law
- History of Law
- Human Rights and Immigration
- Intellectual Property Law
- Browse content in International Law
- Private International Law and Conflict of Laws
- Public International Law
- IT and Communications Law
- Jurisprudence and Philosophy of Law
- Law and Society
- Law and Politics
- Browse content in Legal System and Practice
- Courts and Procedure
- Legal Skills and Practice
- Primary Sources of Law
- Regulation of Legal Profession
- Medical and Healthcare Law
- Browse content in Policing
- Criminal Investigation and Detection
- Police and Security Services
- Police Procedure and Law
- Police Regional Planning
- Browse content in Property Law
- Personal Property Law
- Study and Revision
- Terrorism and National Security Law
- Browse content in Trusts Law
- Wills and Probate or Succession
- Browse content in Medicine and Health
- Browse content in Allied Health Professions
- Arts Therapies
- Clinical Science
- Dietetics and Nutrition
- Occupational Therapy
- Operating Department Practice
- Physiotherapy
- Radiography
- Speech and Language Therapy
- Browse content in Anaesthetics
- General Anaesthesia
- Neuroanaesthesia
- Browse content in Clinical Medicine
- Acute Medicine
- Cardiovascular Medicine
- Clinical Genetics
- Clinical Pharmacology and Therapeutics
- Dermatology
- Endocrinology and Diabetes
- Gastroenterology
- Genito-urinary Medicine
- Geriatric Medicine
- Infectious Diseases
- Medical Oncology
- Medical Toxicology
- Pain Medicine
- Palliative Medicine
- Rehabilitation Medicine
- Respiratory Medicine and Pulmonology
- Rheumatology
- Sleep Medicine
- Sports and Exercise Medicine
- Clinical Neuroscience
- Community Medical Services
- Critical Care
- Emergency Medicine
- Forensic Medicine
- Haematology
- History of Medicine
- Medical Ethics
- Browse content in Medical Dentistry
- Oral and Maxillofacial Surgery
- Paediatric Dentistry
- Restorative Dentistry and Orthodontics
- Surgical Dentistry
- Browse content in Medical Skills
- Clinical Skills
- Communication Skills
- Nursing Skills
- Surgical Skills
- Medical Statistics and Methodology
- Browse content in Neurology
- Clinical Neurophysiology
- Neuropathology
- Nursing Studies
- Browse content in Obstetrics and Gynaecology
- Gynaecology
- Occupational Medicine
- Ophthalmology
- Otolaryngology (ENT)
- Browse content in Paediatrics
- Neonatology
- Browse content in Pathology
- Chemical Pathology
- Clinical Cytogenetics and Molecular Genetics
- Histopathology
- Medical Microbiology and Virology
- Patient Education and Information
- Browse content in Pharmacology
- Psychopharmacology
- Browse content in Popular Health
- Caring for Others
- Complementary and Alternative Medicine
- Self-help and Personal Development
- Browse content in Preclinical Medicine
- Cell Biology
- Molecular Biology and Genetics
- Reproduction, Growth and Development
- Primary Care
- Professional Development in Medicine
- Browse content in Psychiatry
- Addiction Medicine
- Child and Adolescent Psychiatry
- Forensic Psychiatry
- Learning Disabilities
- Old Age Psychiatry
- Psychotherapy
- Browse content in Public Health and Epidemiology
- Epidemiology
- Public Health
- Browse content in Radiology
- Clinical Radiology
- Interventional Radiology
- Nuclear Medicine
- Radiation Oncology
- Reproductive Medicine
- Browse content in Surgery
- Cardiothoracic Surgery
- Gastro-intestinal and Colorectal Surgery
- General Surgery
- Neurosurgery
- Paediatric Surgery
- Peri-operative Care
- Plastic and Reconstructive Surgery
- Surgical Oncology
- Transplant Surgery
- Trauma and Orthopaedic Surgery
- Vascular Surgery
- Browse content in Science and Mathematics
- Browse content in Biological Sciences
- Aquatic Biology
- Biochemistry
- Bioinformatics and Computational Biology
- Developmental Biology
- Ecology and Conservation
- Evolutionary Biology
- Genetics and Genomics
- Microbiology
- Molecular and Cell Biology
- Natural History
- Plant Sciences and Forestry
- Research Methods in Life Sciences
- Structural Biology
- Systems Biology
- Zoology and Animal Sciences
- Browse content in Chemistry
- Analytical Chemistry
- Computational Chemistry
- Crystallography
- Environmental Chemistry
- Industrial Chemistry
- Inorganic Chemistry
- Materials Chemistry
- Medicinal Chemistry
- Mineralogy and Gems
- Organic Chemistry
- Physical Chemistry
- Polymer Chemistry
- Study and Communication Skills in Chemistry
- Theoretical Chemistry
- Browse content in Computer Science
- Artificial Intelligence
- Computer Architecture and Logic Design
- Game Studies
- Human-Computer Interaction
- Mathematical Theory of Computation
- Programming Languages
- Software Engineering
- Systems Analysis and Design
- Virtual Reality
- Browse content in Computing
- Business Applications
- Computer Games
- Computer Security
- Computer Networking and Communications
- Digital Lifestyle
- Graphical and Digital Media Applications
- Operating Systems
- Browse content in Earth Sciences and Geography
- Atmospheric Sciences
- Environmental Geography
- Geology and the Lithosphere
- Maps and Map-making
- Meteorology and Climatology
- Oceanography and Hydrology
- Palaeontology
- Physical Geography and Topography
- Regional Geography
- Soil Science
- Urban Geography
- Browse content in Engineering and Technology
- Agriculture and Farming
- Biological Engineering
- Civil Engineering, Surveying, and Building
- Electronics and Communications Engineering
- Energy Technology
- Engineering (General)
- Environmental Science, Engineering, and Technology
- History of Engineering and Technology
- Mechanical Engineering and Materials
- Technology of Industrial Chemistry
- Transport Technology and Trades
- Browse content in Environmental Science
- Applied Ecology (Environmental Science)
- Conservation of the Environment (Environmental Science)
- Environmental Sustainability
- Environmentalist Thought and Ideology (Environmental Science)
- Management of Land and Natural Resources (Environmental Science)
- Natural Disasters (Environmental Science)
- Nuclear Issues (Environmental Science)
- Pollution and Threats to the Environment (Environmental Science)
- Social Impact of Environmental Issues (Environmental Science)
- History of Science and Technology
- Browse content in Materials Science
- Ceramics and Glasses
- Composite Materials
- Metals, Alloying, and Corrosion
- Nanotechnology
- Browse content in Mathematics
- Applied Mathematics
- Biomathematics and Statistics
- History of Mathematics
- Mathematical Education
- Mathematical Finance
- Mathematical Analysis
- Numerical and Computational Mathematics
- Probability and Statistics
- Pure Mathematics
- Browse content in Neuroscience
- Cognition and Behavioural Neuroscience
- Development of the Nervous System
- Disorders of the Nervous System
- History of Neuroscience
- Invertebrate Neurobiology
- Molecular and Cellular Systems
- Neuroendocrinology and Autonomic Nervous System
- Neuroscientific Techniques
- Sensory and Motor Systems
- Browse content in Physics
- Astronomy and Astrophysics
- Atomic, Molecular, and Optical Physics
- Biological and Medical Physics
- Classical Mechanics
- Computational Physics
- Condensed Matter Physics
- Electromagnetism, Optics, and Acoustics
- History of Physics
- Mathematical and Statistical Physics
- Measurement Science
- Nuclear Physics
- Particles and Fields
- Plasma Physics
- Quantum Physics
- Relativity and Gravitation
- Semiconductor and Mesoscopic Physics
- Browse content in Psychology
- Affective Sciences
- Clinical Psychology
- Cognitive Neuroscience
- Cognitive Psychology
- Criminal and Forensic Psychology
- Developmental Psychology
- Educational Psychology
- Evolutionary Psychology
- Health Psychology
- History and Systems in Psychology
- Music Psychology
- Neuropsychology
- Organizational Psychology
- Psychological Assessment and Testing
- Psychology of Human-Technology Interaction
- Psychology Professional Development and Training
- Research Methods in Psychology
- Social Psychology
- Browse content in Social Sciences
- Browse content in Anthropology
- Anthropology of Religion
- Human Evolution
- Medical Anthropology
- Physical Anthropology
- Regional Anthropology
- Social and Cultural Anthropology
- Theory and Practice of Anthropology
- Browse content in Business and Management
- Business History
- Business Strategy
- Business Ethics
- Business and Government
- Business and Technology
- Business and the Environment
- Comparative Management
- Corporate Governance
- Corporate Social Responsibility
- Entrepreneurship
- Health Management
- Human Resource Management
- Industrial and Employment Relations
- Industry Studies
- Information and Communication Technologies
- International Business
- Knowledge Management
- Management and Management Techniques
- Operations Management
- Organizational Theory and Behaviour
- Pensions and Pension Management
- Public and Nonprofit Management
- Strategic Management
- Supply Chain Management
- Browse content in Criminology and Criminal Justice
- Criminal Justice
- Criminology
- Forms of Crime
- International and Comparative Criminology
- Youth Violence and Juvenile Justice
- Development Studies
- Browse content in Economics
- Agricultural, Environmental, and Natural Resource Economics
- Asian Economics
- Behavioural Finance
- Behavioural Economics and Neuroeconomics
- Econometrics and Mathematical Economics
- Economic Methodology
- Economic Systems
- Economic History
- Economic Development and Growth
- Financial Markets
- Financial Institutions and Services
- General Economics and Teaching
- Health, Education, and Welfare
- History of Economic Thought
- International Economics
- Labour and Demographic Economics
- Law and Economics
- Macroeconomics and Monetary Economics
- Microeconomics
- Public Economics
- Urban, Rural, and Regional Economics
- Welfare Economics
- Browse content in Education
- Adult Education and Continuous Learning
- Care and Counselling of Students
- Early Childhood and Elementary Education
- Educational Equipment and Technology
- Educational Strategies and Policy
- Higher and Further Education
- Organization and Management of Education
- Philosophy and Theory of Education
- Schools Studies
- Secondary Education
- Teaching of a Specific Subject
- Teaching of Specific Groups and Special Educational Needs
- Teaching Skills and Techniques
- Browse content in Environment
- Applied Ecology (Social Science)
- Climate Change
- Conservation of the Environment (Social Science)
- Environmentalist Thought and Ideology (Social Science)
- Natural Disasters (Environment)
- Social Impact of Environmental Issues (Social Science)
- Browse content in Human Geography
- Cultural Geography
- Economic Geography
- Political Geography
- Browse content in Interdisciplinary Studies
- Communication Studies
- Museums, Libraries, and Information Sciences
- Browse content in Politics
- African Politics
- Asian Politics
- Chinese Politics
- Comparative Politics
- Conflict Politics
- Elections and Electoral Studies
- Environmental Politics
- European Union
- Foreign Policy
- Gender and Politics
- Human Rights and Politics
- Indian Politics
- International Relations
- International Organization (Politics)
- International Political Economy
- Irish Politics
- Latin American Politics
- Middle Eastern Politics
- Political Theory
- Political Methodology
- Political Communication
- Political Philosophy
- Political Sociology
- Political Behaviour
- Political Economy
- Political Institutions
- Politics and Law
- Public Administration
- Public Policy
- Quantitative Political Methodology
- Regional Political Studies
- Russian Politics
- Security Studies
- State and Local Government
- UK Politics
- US Politics
- Browse content in Regional and Area Studies
- African Studies
- Asian Studies
- East Asian Studies
- Japanese Studies
- Latin American Studies
- Middle Eastern Studies
- Native American Studies
- Scottish Studies
- Browse content in Research and Information
- Research Methods
- Browse content in Social Work
- Addictions and Substance Misuse
- Adoption and Fostering
- Care of the Elderly
- Child and Adolescent Social Work
- Couple and Family Social Work
- Developmental and Physical Disabilities Social Work
- Direct Practice and Clinical Social Work
- Emergency Services
- Human Behaviour and the Social Environment
- International and Global Issues in Social Work
- Mental and Behavioural Health
- Social Justice and Human Rights
- Social Policy and Advocacy
- Social Work and Crime and Justice
- Social Work Macro Practice
- Social Work Practice Settings
- Social Work Research and Evidence-based Practice
- Welfare and Benefit Systems
- Browse content in Sociology
- Childhood Studies
- Community Development
- Comparative and Historical Sociology
- Economic Sociology
- Gender and Sexuality
- Gerontology and Ageing
- Health, Illness, and Medicine
- Marriage and the Family
- Migration Studies
- Occupations, Professions, and Work
- Organizations
- Population and Demography
- Race and Ethnicity
- Social Theory
- Social Movements and Social Change
- Social Research and Statistics
- Social Stratification, Inequality, and Mobility
- Sociology of Religion
- Sociology of Education
- Sport and Leisure
- Urban and Rural Studies
- Browse content in Warfare and Defence
- Defence Strategy, Planning, and Research
- Land Forces and Warfare
- Military Administration
- Military Life and Institutions
- Naval Forces and Warfare
- Other Warfare and Defence Issues
- Peace Studies and Conflict Resolution
- Weapons and Equipment

- < Previous chapter
- Next chapter >
2 (page 14) p. 14 The water molecule and its interactions
- Published: August 2015
- Cite Icon Cite
- Permissions Icon Permissions
‘The water molecule and its interactions’ discusses the structural and electrical properties of the water molecule. A water molecule is made up of two hydrogen atoms connected by covalent bonds to one oxygen atom. Water molecules interact with each other through a type of interaction called hydrogen bonding. A tetrahedral arrangement of four water molecules around a central one is the key to understanding water. It helps to explain the structure of water in its various states, its properties, and how it interacts with other kinds of molecules, allowing exploration of the properties and behaviour of the wide range of chemical, physical, and biological systems in which water is involved.
Signed in as
Institutional accounts.
- Google Scholar Indexing
- GoogleCrawler [DO NOT DELETE]
Personal account
- Sign in with email/username & password
- Get email alerts
- Save searches
- Purchase content
- Activate your purchase/trial code
Institutional access
- Sign in with a library card Sign in with username/password Recommend to your librarian
- Institutional account management
- Get help with access
Access to content on Oxford Academic is often provided through institutional subscriptions and purchases. If you are a member of an institution with an active account, you may be able to access content in one of the following ways:
IP based access
Typically, access is provided across an institutional network to a range of IP addresses. This authentication occurs automatically, and it is not possible to sign out of an IP authenticated account.
Sign in through your institution
Choose this option to get remote access when outside your institution. Shibboleth/Open Athens technology is used to provide single sign-on between your institution’s website and Oxford Academic.
- Click Sign in through your institution.
- Select your institution from the list provided, which will take you to your institution's website to sign in.
- When on the institution site, please use the credentials provided by your institution. Do not use an Oxford Academic personal account.
- Following successful sign in, you will be returned to Oxford Academic.
If your institution is not listed or you cannot sign in to your institution’s website, please contact your librarian or administrator.
Sign in with a library card
Enter your library card number to sign in. If you cannot sign in, please contact your librarian.
Society Members
Society member access to a journal is achieved in one of the following ways:
Sign in through society site
Many societies offer single sign-on between the society website and Oxford Academic. If you see ‘Sign in through society site’ in the sign in pane within a journal:
- Click Sign in through society site.
- When on the society site, please use the credentials provided by that society. Do not use an Oxford Academic personal account.
If you do not have a society account or have forgotten your username or password, please contact your society.
Sign in using a personal account
Some societies use Oxford Academic personal accounts to provide access to their members. See below.
A personal account can be used to get email alerts, save searches, purchase content, and activate subscriptions.
Some societies use Oxford Academic personal accounts to provide access to their members.
Viewing your signed in accounts
Click the account icon in the top right to:
- View your signed in personal account and access account management features.
- View the institutional accounts that are providing access.
Signed in but can't access content
Oxford Academic is home to a wide variety of products. The institutional subscription may not cover the content that you are trying to access. If you believe you should have access to that content, please contact your librarian.
For librarians and administrators, your personal account also provides access to institutional account management. Here you will find options to view and activate subscriptions, manage institutional settings and access options, access usage statistics, and more.
Our books are available by subscription or purchase to libraries and institutions.
- About Oxford Academic
- Publish journals with us
- University press partners
- What we publish
- New features
- Open access
- Rights and permissions
- Accessibility
- Advertising
- Media enquiries
- Oxford University Press
- Oxford Languages
- University of Oxford
Oxford University Press is a department of the University of Oxford. It furthers the University's objective of excellence in research, scholarship, and education by publishing worldwide
- Copyright © 2024 Oxford University Press
- Cookie settings
- Cookie policy
- Privacy policy
- Legal notice
This Feature Is Available To Subscribers Only
Sign In or Create an Account
This PDF is available to Subscribers Only
For full access to this pdf, sign in to an existing account, or purchase an annual subscription.
If you're seeing this message, it means we're having trouble loading external resources on our website.
If you're behind a web filter, please make sure that the domains *.kastatic.org and *.kasandbox.org are unblocked.
To log in and use all the features of Khan Academy, please enable JavaScript in your browser.
AP®︎/College Biology
Course: ap®︎/college biology > unit 1.
- Hydrogen bonding in water
Hydrogen bonds in water
- Capillary action and why we see a meniscus
- Surface tension
- Cohesion and adhesion of water
- Water as a solvent
- Specific heat, heat of vaporization, and density of water
- Importance of water for life
- Lesson summary: Water and life
- Structure of water and hydrogen bonding
Introduction to the properties of water
- Solvent properties of water : Learn how and why water dissolves many polar and charged molecules.
- Cohesion and adhesion of water : Water can stick to itself (cohesion) and other molecules (adhesion).
- Specific heat, heat of vaporization, and density of water : Water has a high heat capacity and heat of vaporization, and ice—solid water—is less dense than liquid water.
Polarity of water molecules
Hydrogen bonding of water molecules, attribution:, works cited.
- "Bacterial cell structure." Wikipedia. June 24, 2015. Accessed July 5, 2015. https://en.wikipedia.org/?title=Bacterial_cell_structure .
- "Properties of water." Wikipedia. May 27, 2016. Accessed May 28, 2016. https://en.wikipedia.org/wiki/Properties_of_water .
- Reece, J. B., L. A. Urry, M. L. Cain, S. A. Wasserman, P. V. Minorsky, and R. B. Jackson. "Polar covalent bonds in water molecules result in hydrogen bonding." In Campbell Biology , 45. 10th ed. San Francisco, CA: Pearson, 2011.
Additional references
Want to join the conversation.
- Upvote Button navigates to signup page
- Downvote Button navigates to signup page
- Flag Button navigates to signup page

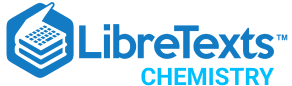
- school Campus Bookshelves
- menu_book Bookshelves
- perm_media Learning Objects
- login Login
- how_to_reg Request Instructor Account
- hub Instructor Commons
- Download Page (PDF)
- Download Full Book (PDF)
- Periodic Table
- Physics Constants
- Scientific Calculator
- Reference & Cite
- Tools expand_more
- Readability
selected template will load here
This action is not available.
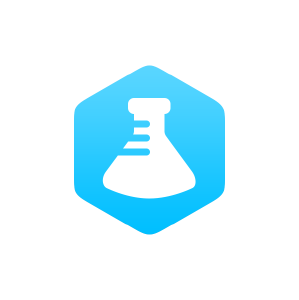
13.5: The Structure and Properties of Water
- Last updated
- Save as PDF
- Page ID 41392
With 70% of our earth being ocean water and 65% of our bodies being water, it is hard to not be aware of how important it is in our lives. There are 3 different forms of water, or H 2 O: solid (ice), liquid (water), and gas (steam). Because water seems so ubiquitous, many people are unaware of the unusual and unique properties of water, including:
Boiling Point and Freezing Point
Surface tension, heat of vaporization, and vapor pressure.
- Viscosity and Cohesion
- Solid State
- Liquid State
If you look at the periodic table and locate tellurium (atomic number: 52), you find that the boiling points of hydrides decrease as molecule size decreases. So the hydride for tellurium: H 2 Te (hydrogen telluride) has a boiling point of -4°C . Moving up, the next hydride would be H 2 Se (hydrogen selenide) with a boiling point of -42°C . One more up and you find that H 2 S (hydrogen sulfide) has a boiling point at -62°C . The next hydride would be H 2 O (WATER!) . And we all know that the boiling point of water is 100°C . So despite its small molecular weight, water has an incredibly big boiling point. This is because water requires more energy to break its hydrogen bonds before it can then begin to boil. The same concept is applied to freezing point as well, as seen in the table below. The boiling and freezing points of water enable the molecules to be very slow to boil or freeze, this is important to the ecosystems living in water. If water was very easy to freeze or boil, drastic changes in the environment and so in oceans or lakes would cause all the organisms living in water to die. This is also why sweat is able to cool our bodies.
Besides mercury, water has the highest surface tension for all liquids. Water's high surface tension is due to the hydrogen bonding in water molecules. Water also has an exceptionally high heat of vaporization . Vaporization occurs when a liquid changes to a gas, which makes it an endothermic reaction. Water's heat of vaporization is 41 kJ/mol. Vapor pressure is inversely related to intermolecular forces, so those with stronger intermolecular forces have a lower vapor pressure. Water has very strong intermolecular forces, hence the low vapor pressure, but it's even lower compared to larger molecules with low vapor pressures.
- Viscosity is the property of fluid having high resistance to flow. We normally think of liquids like honey or motor oil being viscous, but when compared to other substances with like structures, water is viscous. Liquids with stronger intermolecular interactions are usually more viscous than liquids with weak intermolecular interactions.
- Cohesion is intermolecular forces between like molecules; this is why water molecules are able to hold themselves together in a drop. Water molecules are very cohesive because of the molecule's polarity. This is why you can fill a glass of water just barely above the rim without it spilling.
Solid State (Ice)
All substances, including water, become less dense when they are heated and more dense when they are cooled. So if water is cooled, it becomes more dense and forms ice. Water is one of the few substances whose solid state can float on its liquid state! Why? Water continues to become more dense until it reaches 4°C. After it reaches 4°C, it becomes LESS dense. When freezing, molecules within water begin to move around more slowly, making it easier for them to form hydrogen bonds and eventually arrange themselves into an open crystalline, hexagonal structure. Because of this open structure as the water molecules are being held further apart, the volume of water increases about 9%. So molecules are more tightly packed in water's liquid state than its solid state. This is why a can of soda can explode in the freezer.
Liquid State (Liquid Water)
It is very rare to find a compound that lacks carbon to be a liquid at standard temperatures and pressures. So it is unusual for water to be a liquid at room temperature! Water is liquid at room temperature so it's able to move around quicker than it is as solid, enabling the molecules to form fewer hydrogen bonds resulting in the molecules being packed more closely together. Each water molecule links to four others creating a tetrahedral arrangement, however they are able to move freely and slide past each other, while ice forms a solid, larger hexagonal structure.
Gas State (Steam)
As water boils, its hydrogen bonds are broken. Steam particles move very far apart and fast, so barely any hydrogen bonds have the time to form. So, less and less hydrogen bonds are present as the particles reach the critical point above steam. The lack of hydrogen bonds explains why steam causes much worse burns that water. Steam contains all the energy used to break the hydrogen bonds in water, so when steam hits your face you first absorb the energy the steam has taken up from breaking the hydrogen bonds it its liquid state. Then, in an exothermic reaction, steam is converted into liquid water and heat is released. This heat adds to the heat of boiling water as the steam condenses on your skin.
Water as the "Universal Solvent"
Because of water's polarity, it is able to dissolve or dissociate many particles. Oxygen has a slightly negative charge, while the two hydrogens have a slightly positive charge. The slightly negative particles of a compound will be attracted to water's hydrogen atoms, while the slightly positive particles will be attracted to water's oxygen molecule; this causes the compound to dissociate. Besides the explanations above, we can look to some attributes of a water molecule to provide some more reasons of water's uniqueness:
- Forgetting fluorine, oxygen is the most electronegative non-noble gas element, so while forming a bond, the electrons are pulled towards the oxygen atom rather than the hydrogen. This creates 2 polar bonds, which make the water molecule more polar than the bonds in the other hydrides in the group.
- A 104.5° bond angle creates a very strong dipole.
- Water has hydrogen bonding which probably is a vital aspect in waters strong intermolecular interaction
Why is this important for the real world?
The properties of water make it suitable for organisms to survive in during differing weather conditions. Ice freezes as it expands, which explains why ice is able to float on liquid water. During the winter when lakes begin to freeze, the surface of the water freezes and then moves down toward deeper water; this explains why people can ice skate on or fall through a frozen lake. If ice was not able to float, the lake would freeze from the bottom up killing all ecosystems living in the lake. However ice floats, so the fish are able to survive under the surface of the ice during the winter. The surface of ice above a lake also shields lakes from the cold temperature outside and insulates the water beneath it, allowing the lake under the frozen ice to stay liquid and maintain a temperature adequate for the ecosystems living in the lake to survive.
- Cracolice, Mark S. and Edward Peters I. Basics of Introductory Chemistry . Thompson, Brooks/Cole Publishing Company. 2006
- Petrucci, et al. General Chemistry: Principles & Modern Applications: AIE (Hardcover). Upper Saddle River: Pearson/Prentice Hall, 2007.
Contributors and Attributions
- Corinne Yee (UCD), Desiree Rozzi (UCD)
Free Shipping for US orders over $72 and International orders over $250!
Great news for structured water enthusiasts in australia.
Fellow Australians! Our heart goes out to you for all you’ve been through over the last year. To support faster access to products, we now have many products from our shop available for you locally . Keep raising the vibration! We love you!
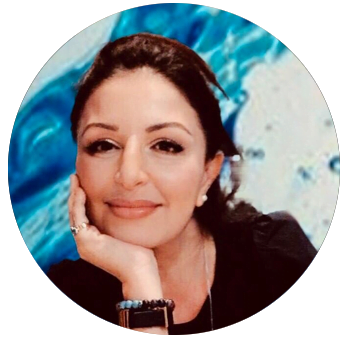
You can contact our local rep, Jules Serhan , and she will get your questions and order sorted!
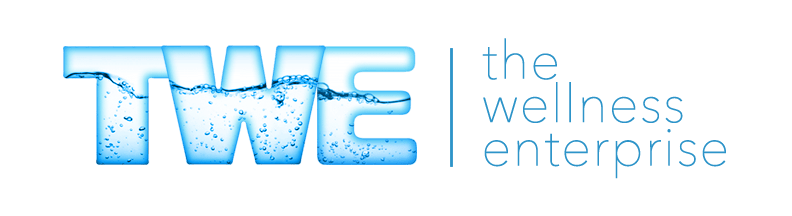
- Cosmic Tower
- How Aqua Energizers Work
- Testing and Certification
- Quality Materials
- Choose Your Aqua Energizer
- Shop Aqua Energizers
- Nano Soma Nutritional Immune System Support
- Metasomer Topical Gel
- Metasomer Natural Skin Cream
- The Best Water Pitcher Ever
- Best Water Filter Ever
- Structured Water
- What is Structured Water?
- Structured Water FAQ
- Is Structured Water Real? – The Evidence is in Nature
- How Long Does Structured Water Stay Structured?
- Read all Structured Water >
Water and Plants
- Structured Water for Agriculture: Water Quality Like Rainwater 100% of the Season
- Grow a Thriving Garden with Structured Water
- Regenerative Agriculture
Water Science
- What Scientists Say About Structured Water
Dr. Masaru Emoto and Water Consciousness
- Dr. Gerald Pollack
- Dr. Thomas Cowan on the Importance of Structured Water
- Read all Water Science >
Water Lifestyle
- The Plastic Crisis Solution
- Save Money with Structured Water Devices
- How Hydration Can Help With Anxiety
- Water: Elixir of Life
- Read all Water Lifestyle >
Water and Health
- Doctors Say Structured Water is Best for Hydration
- Top 5 Benefits of Structured Water That Improve Your Health
- Doctors Prescription to Hydrate Now Course
- How to Protect Yourself from EMFs
- Read all Water and Health >
Wellness Brands
- AQUA ENERGIZER™
Water Filtration
- The Best Water to Drink
- Why Filter Water
- Should I Add Minerals to My Water?
- Read all Water Filtration >
Water Library
- Water and the Cell
- Beyond Alkaline Water
The Hidden Messages in Water
- The Fourth Phase of Water
- View All the Books >
Shop By Category
- Structured Water Discounts
- Trade In Program
- Water Filters & Replacements
- Water Mineralization
- Water Accessories
- Water for Plants
Shop By Brand
- Best Water Ever
Shop By Use
- Whole House
- Refrigerator
Top Sellers
- AQUA ENERGIZER™ Whole House
- AQUA ENERGIZER™ Portable
- Jivara® Mountain Spring
- Nano Soma Spray
- Nano Soma Metasomer Gel
- Best Water Pitcher Ever
- March 23, 2017
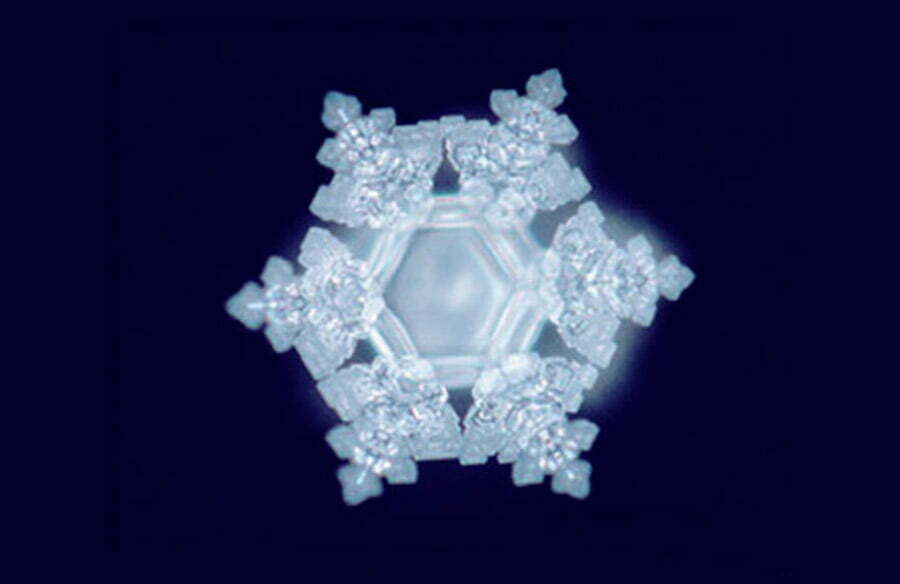
Blog Content Navigation
Who was dr. masaru emoto.
Dr. Masaru Emoto, the Japanese scientist who revolutionized the idea that our thoughts and intentions impact the physical realm, is one of the most important water researchers the world has known. For over 20 years until he passed away in 2014, he studied the scientific evidence of how the molecular structure in water transforms when it is exposed to human words, thoughts, sounds and intentions.
The extraordinary life work of Dr. Emoto is documented in the New York Times Bestseller, The Hidden Messages in Water . In his book, Dr. Emoto demonstrates how water exposed to loving, benevolent, and compassionate human intention results in aesthetically pleasing physical molecular formations in the water while water exposed to fearful and discordant human intentions results in disconnected, disfigured, and “unpleasant” physical molecular formations. He did this through Magnetic Resonance Analysis technology and high-speed photographs.
See the following water crystal photographs from Dr. Emoto’s work. Each water crystal you see was exposed to the word it has written next to it prior to being photographed:
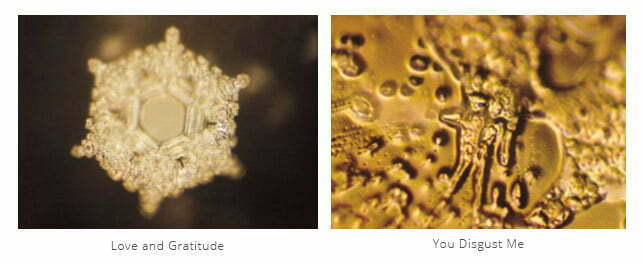
His research also showed us how polluted and toxic water, when exposed to to prayer and intention can be altered and restored to beautifully formed geometric crystals found in clean, healthy water. The following photos are images of photographs of the the water in the Fujiwara Dam before and after the Reverend Kato Hoki, chief priest of Jyuhouin Temple, offered an hour long prayer over it.
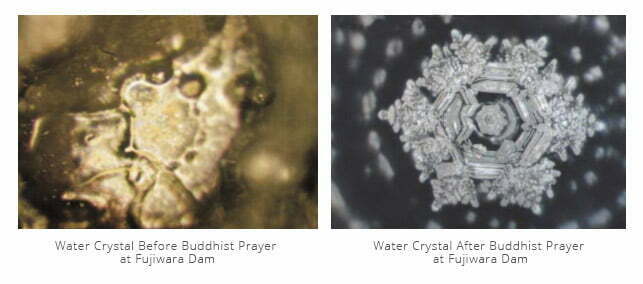
How Music Affects Water?
Dr. Emoto also studied how sound affects water. The Emoto music studies demonstrate how certain types of sound, like classical music, generate beautiful crystalline patterns, while heavy metal music, generate ugly and distorted crystalline formations. In the images below you see the crystalline formation resulting from water being exposed to Mozart’s Symphony No. 40 and then in contrast what the water crystal image looks like after listening to heavy metal music.
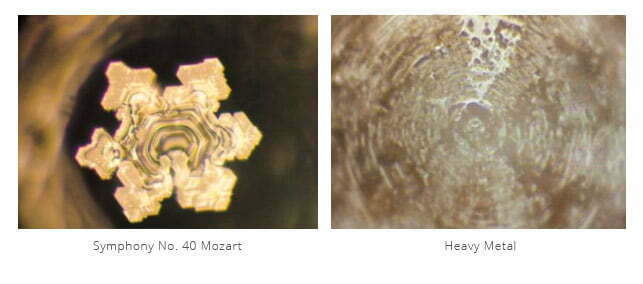
Dr. Masaru Emoto put Water as a Living Consciousness on the map for the scientific world. He showed us how water is an energy capable of more than we ever imagined. The power human thoughts, sounds and intentions has to strengthen and disempower is one of the greatest discoveries of our time.
His work has us question, if water is affected by the words, intentions, and energies, what about human beings, who are made of mostly water? If we transform the water and thoughts we are made of, what else is possible?
There are legions of scientists who have built upon the breakthroughs of Dr. Emoto and are offering practical technologies for everyday life. One such example who we find particularly inspiring is the Austrian Engineer Bernard Ratheiser. Since 1994, he has developed devices that restructure water molecules and assist water in being its highest vibration as living energy.
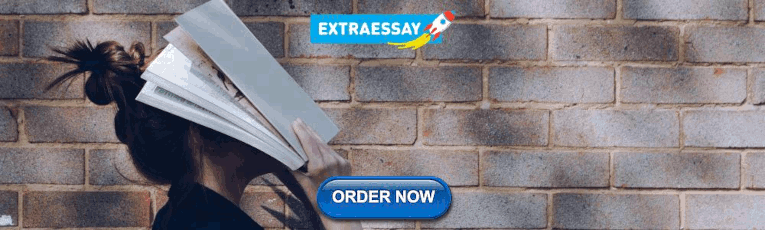
Hydrate your body with certified water
Water crystals and structured water.
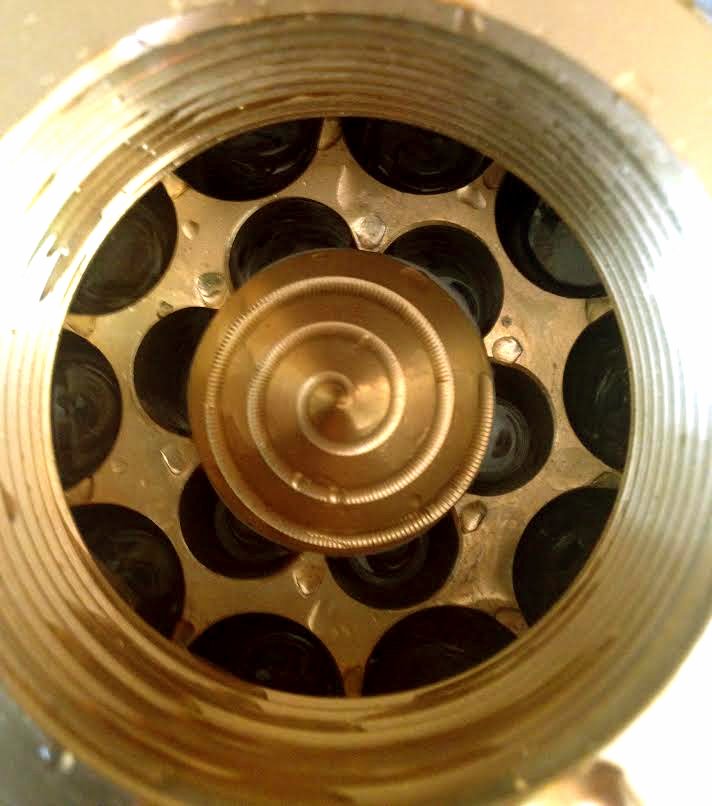
With vortexing and sacred geometry along with nature’s energies through precious stones, Bernhard Ratheiser has created a way for water to be revitalized when it passes through one of his water structuring devices. Every measurement in his beautiful devices is designed to evoke the energy of the cosmos and realign water with the energy of creation.
When water passes through his UMH Devices, everything life supporting in the water is enhanced, energy increases, memory is erased, and toxins are energetically neutralized. The following are actual photos of water that passed through the UMH device using the Emoto Protocol and demonstrates how inherent spring water quality is restored. You can see how the water crystal photos before and after passing through the UMH Master whole house device result in a significant improvement in crystalline structure.
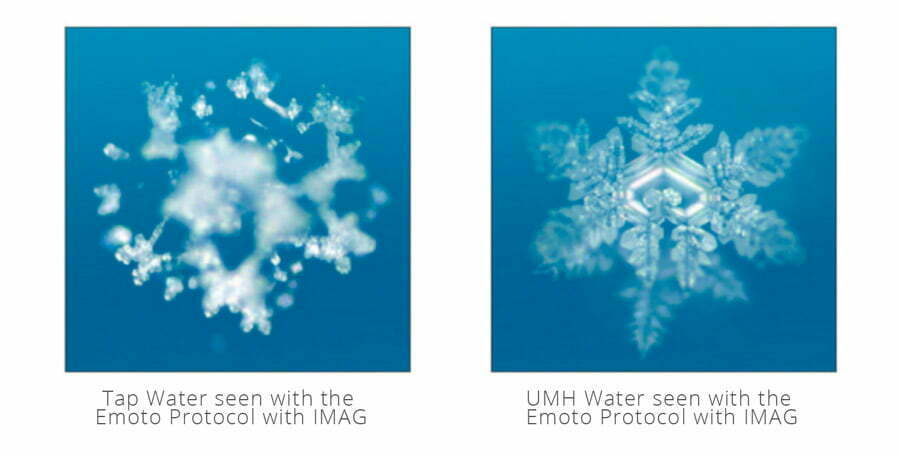
Another of our collections, VitaJuwel, asked the Hadolife Laboratories in Austria, which was co-founded by Dr. Masaru Emoto and his oldest student to take microscopic photos of water before and after it had been treated with VitaJuwel. You can see the stunning result below that’s an example of the crystalline structure you’ll be drinking in the water you can charge in the VitaJuwel Wellness bottl e found at The Wellness Enterprise.
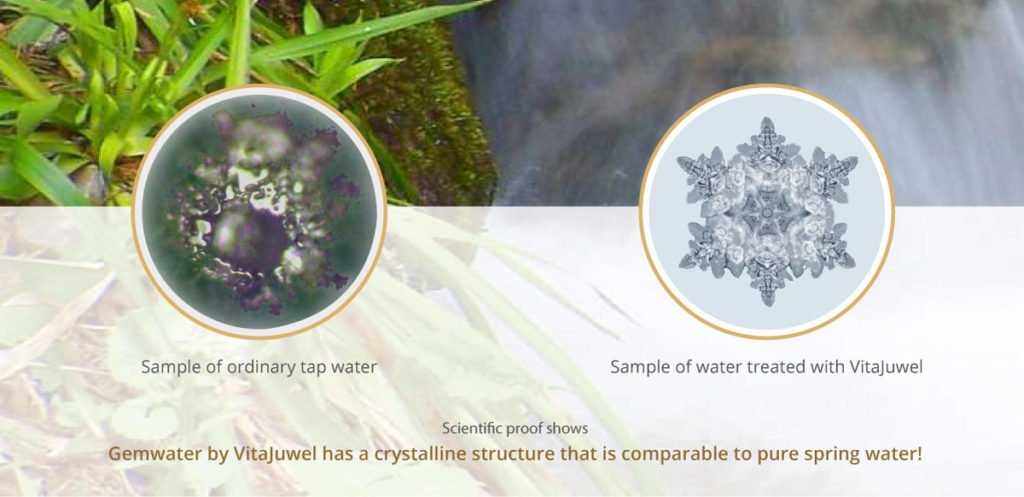
We live in exciting times when new technologies like water structuring devices are making it easy for us to access water’s highest vibration. Our understanding of the natural world is moving to new levels as technology embraces the truth of structured water science with sacred geometry, vortexing, and gemstones.
Dr. Zach Bush: Water and Memory
Thanks to how Dr. Masaru Emoto’s work opened the door to understanding water and memory, many doctors, scientists and researchers have made exciting new discoveries. One of those doctors is triple board certified M.D, Zach Bush. In the following video, Zach Bush shares that there is no part of the human brain that holds long term memory, but there is, however, evidence that memory is held elsewhere. Watch the video below to hear what he has to say about where and how and how memory is held in the human body.
Certified Structured Water Devices Create Beautiful Imprints in Your Water.
- related articles -.
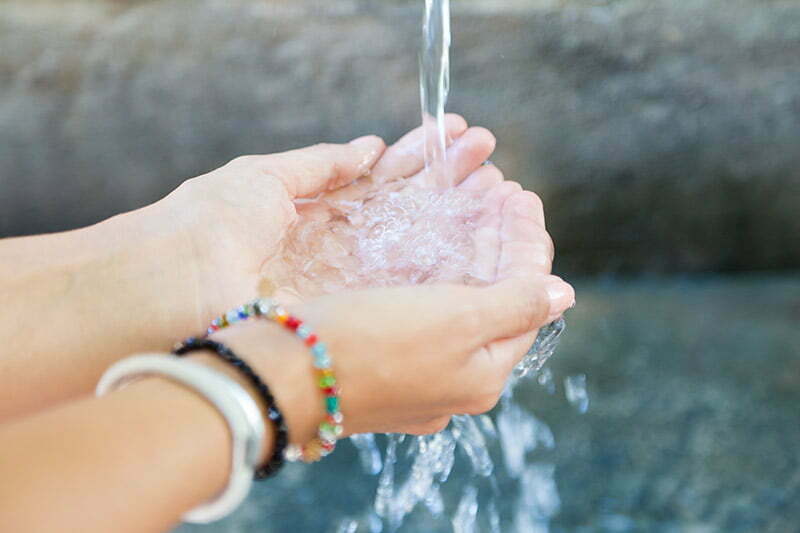
September 17, 2021
Is structured water real? Let's take a look into where it can be found in Nature and how beneficial it can be for humans, animals, plants, and even entire ecosystems.
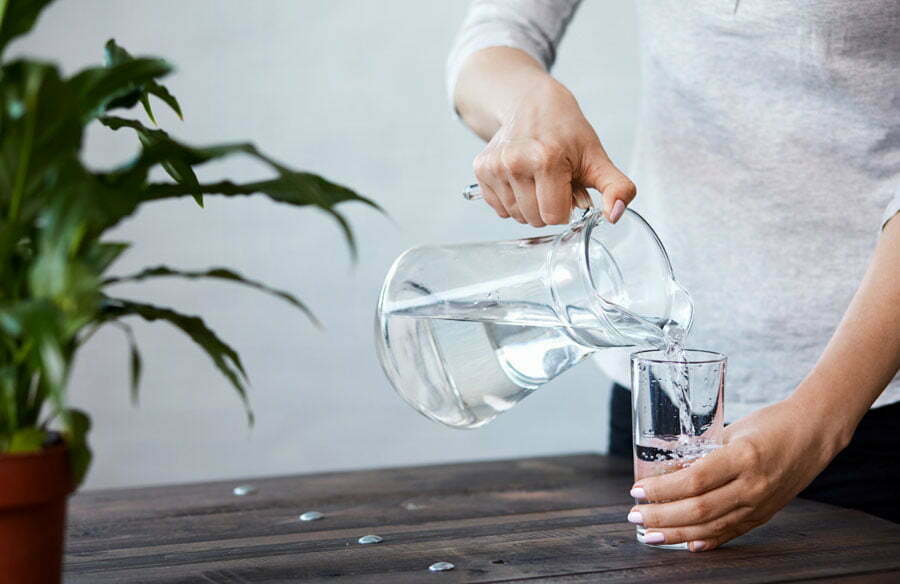
August 5, 2021
A question many people ask when they begin drinking structured water is: how long does structured water stay structured? Here, we break down this question by exploring the many different environments water can be exposed to that affect the molecular structure of it.
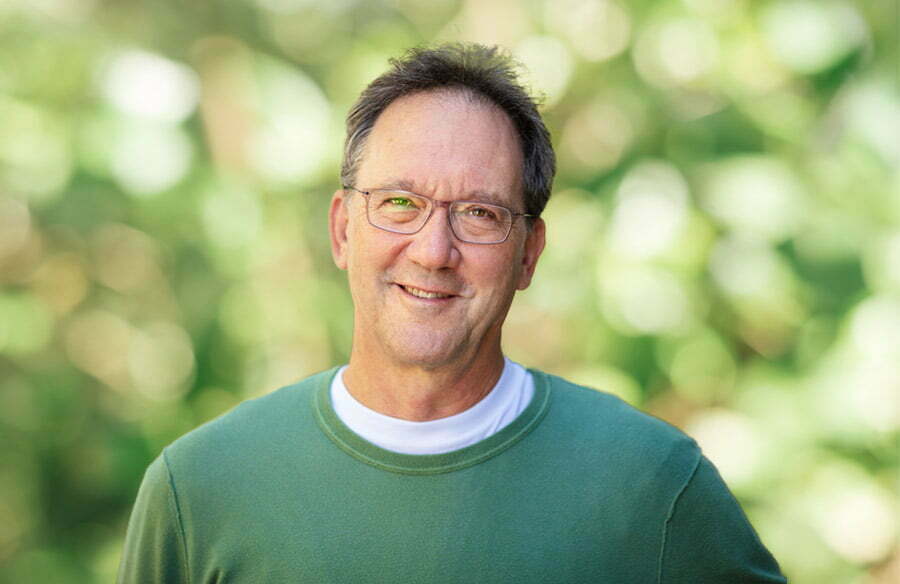
July 7, 2021
“Health is the state of perfect intracellular gels.” Experience the significant relationship Dr. Thomas Cowan has unveiled between structured water and blood flow, cancerous tumors, and epigenetics. This information goes well beyond traditional hydration. Water, and its unique structure, is pivotal to life itself.
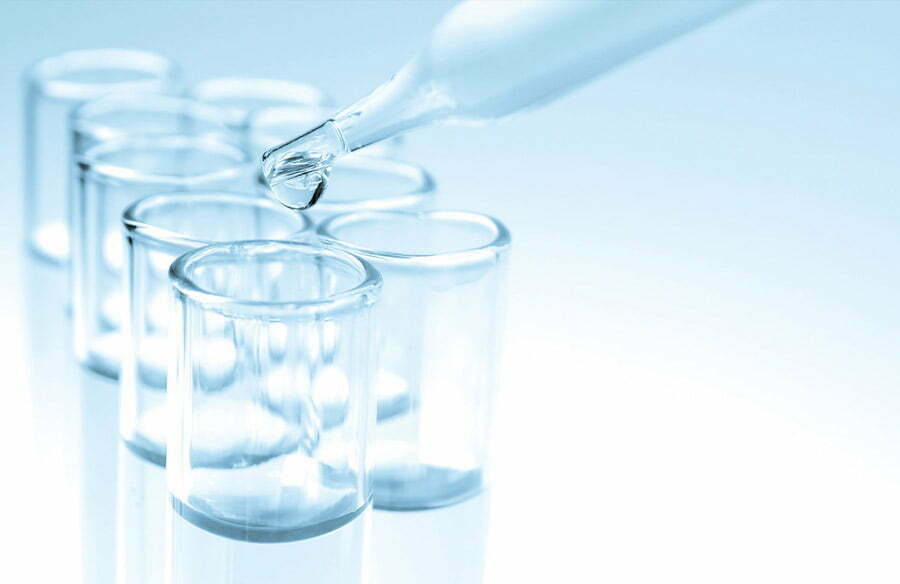
January 21, 2021
Groundbreaking Results For Structured Water Science: AQUA ENERGIZERS™ Remove Toxins
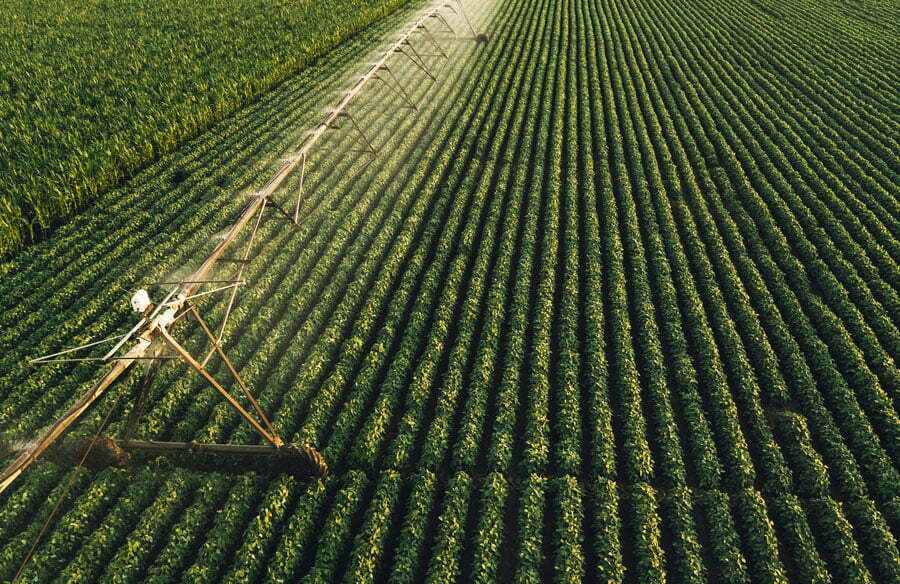
December 4, 2020
Structured water hydrates plants like rainwater does. Refresh your fields and decrease season water usage, increase your yields, and lower your production costs.
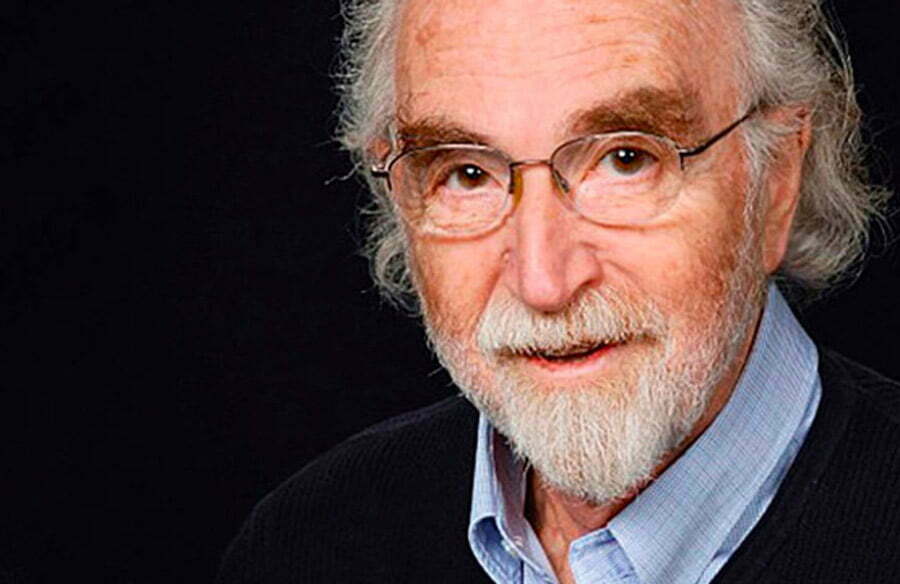
The most important scientist you've never heard about
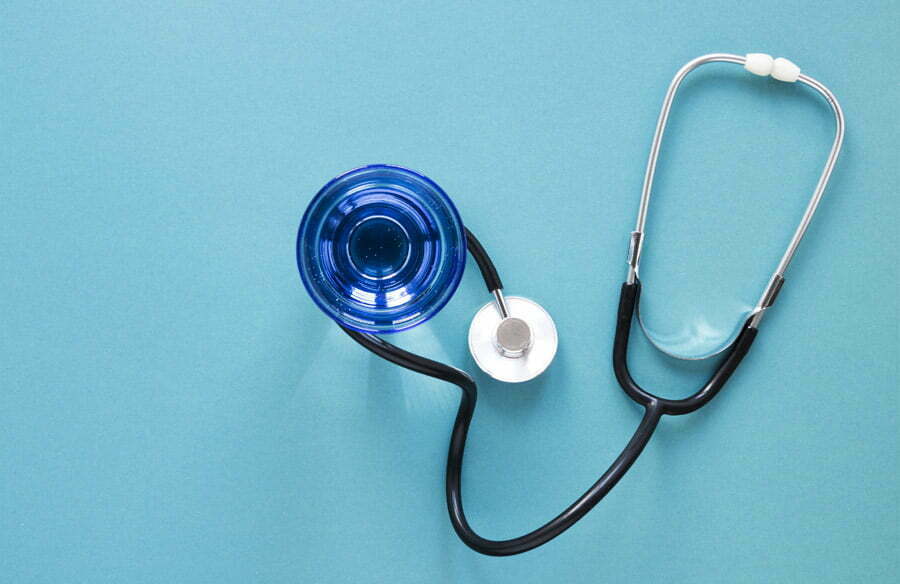
November 16, 2020
Top medical doctors explain why Structured Water is the best if you want to be properly hydrated.
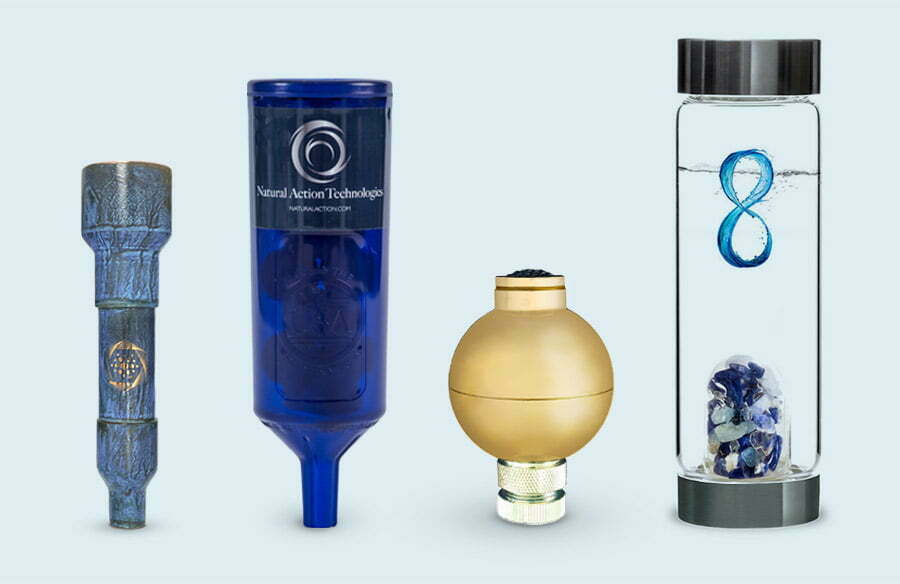
June 5, 2019
*“Natural Action” and “Natural Action Technologies” and their logo are trademarks of Natural Action Technologies. The Wellness Enterprise Inc is no longer an authorized dealer of Natural Action Technologies products and we are now a competitor.
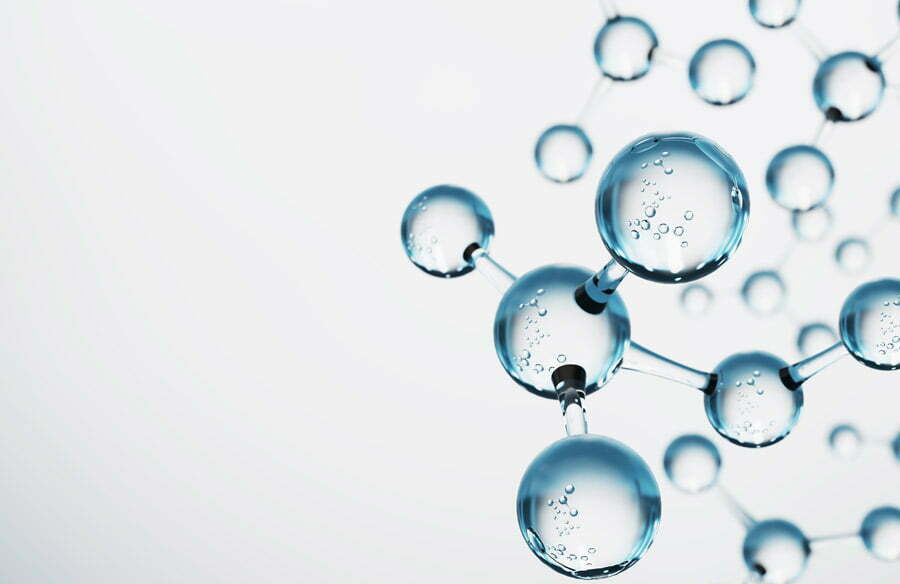
June 3, 2019
The Structured Water devices are a flow-form, or shape, which allows water to revitalize itself with the energy of Creation by interacting with Sacred Geometry and a double vortex spin.
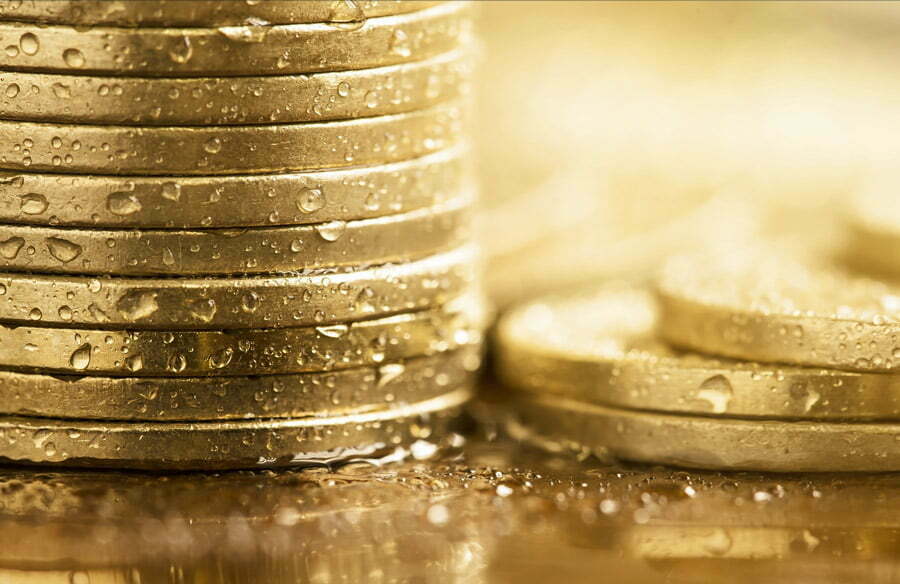
June 2, 2019
How much money can people save using Structured Water devices and why? Discover how Structured Water can help your pocket and the planet.
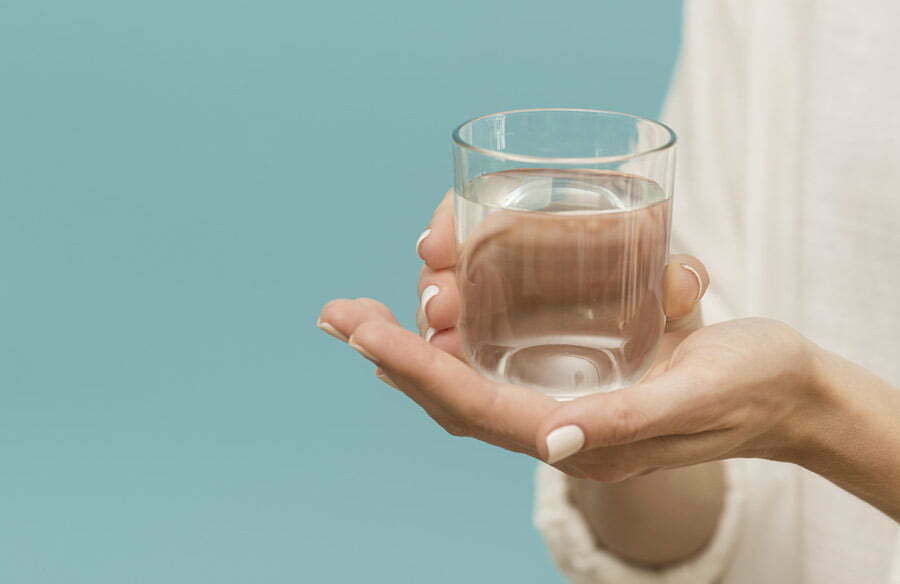
December 5, 2017
Understanding that water has memory is more than knowing that water crystals are influenced by words or sounds. Water truly listens to us, even mirroring our thoughts and attitudes back to us.
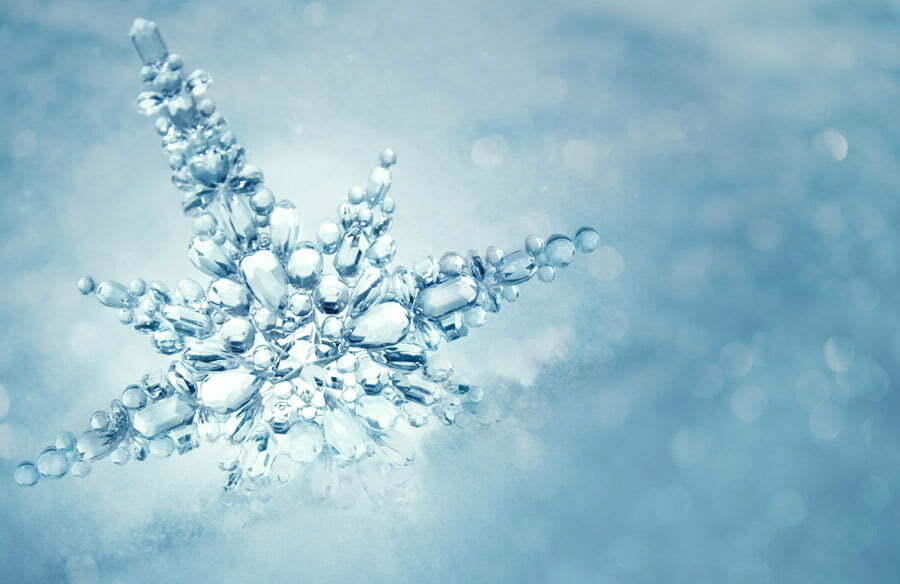
December 2, 2017
One of the greatest virtues we have as human beings is curiosity. The Universe, playing along with us gave us many mysteries to unravel. Water is one of them. Something so obvious, but so fundamental hides secrets that we are just beginning to discover.
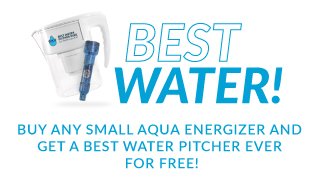
To be a part of our community and get cool information about water, enter your email address.
DISCLOSURE: We may earn a commission when you use one of our coupons/links to make a purchase.
Are you inspired by our mission and want to join our distribution team? Opportunities exist for like-minded people. To learn more, contact us and tell us about yourself. The Wellness Enterprise © 2023 . All rights reserved.
Privacy Overview

- News Releases
The shape of water: What water molecules look like on the surface of materials
Scientist combine data analysis techniques with molecular dynamics simulations to understand the structure of water on material surfaces.
Tokyo University of Science
image: Combination of data analysis techniques with molecular dynamics simulations can help us understand the structure of water on material surfaces. view more
Credit: Tokyo University of Science
Understanding the various molecular interactions and structures that arise among surface water molecules would enable scientists and engineers to develop all sorts of novel hydrophobic/hydrophilic materials or improve existing ones. For example, the friction caused by water on ships could be reduced through materials engineering, leading to higher efficiency. Other applications include, but are not limited to, medical implants and anti-icing surfaces for airplanes. However, the phenomena that occur in surface water are so complicated that Tokyo University of Science, Japan, has established a dedicated research center, called "Water Frontier Science and Technology," where various research groups tackle this problem from different angles (theoretical analysis, experimental studies, material development, and so on). Prof Takahiro Yamamoto leads a group of scientists at this center, and they try to solve this mystery through simulations of the microscopic structures, properties, and functions of water on the surface of materials.
For this study in particular, which was published in the Japanese Journal of Applied Physics , the researchers from Tokyo University of Science, in collaboration with researchers from the Science Solutions Division, Mizuho Information & Research Institute, Inc., focused on the interactions between water molecules and graphene, a charge-neutral carbon-based material that can be made atomically flat. "Surface water on carbon nanomaterials such as graphene has attracted much attention because the properties of these materials make them ideal for studying the microscopic structure of surface water," explains Prof Yamamoto. It had been already pointed out in previous studies that water molecules on graphene tend to form stable polygonal (2D) shapes in both surface water and "free" water (water molecules away from the surface of the material). Moreover, it had been noted that the probability of finding these structures was drastically different in surface water than in free water. However, the differences between surface and free water have to be established, and the transition between the two is difficult to analyze using conventional simulation methods.
Considering this situation, the research team decided to combine a method taken from data science, called persistent homology (PH), with simulations of molecular dynamics. PH allows for the characterization of data structures, including those contained in images/graphics, but it can also be used in materials science to find stable 3D structures between molecules. "Our study represents the first time PH was used for a structural analysis of water molecules," remarks Prof Yamamoto. With this strategy, the researchers were able to obtain a better idea of what happens to surface water molecules as more layers of water are added on top.
When a single layer of water molecules is laid on top of graphene, the water molecules align so that their hydrogen atoms form stable polygonal structures with different numbers of sides through hydrogen bonds. This "fixes" the orientation and relative position of these first-layer water molecules, which are now forming shapes parallel to the graphene layer. If a second layer of water molecules is added, the molecules from the first and second layers form 3D structures called tetrahedrons, which resemble a pyramid but with a triangular base. Curiously, these tetrahedrons are mostly pointing downwards (towards the graphene layer), because this orientation is "energetically favorable." In other words, the order from the first layer translates to the second one to form these 3D structures with a consistent orientation. However, as a third and more layers are added, the tetrahedrons that form don't necessarily point downwards and instead appear to be free to point in any direction, swayed by the surrounding forces. "These results confirm that the crossover between surface and free water occurs within only three layers of water," explains Prof Yamamoto.
The researchers have provided a video of one of their simulations where these 2D and 3D structures are highlighted, allowing one to understand the full picture. "Our study is a good example of the application of modern data analysis techniques to gain new and important insights," adds Prof Yamamoto. What's more, these predictions should not be hard to measure experimentally on graphene through atomic-force microscopy techniques, which would, without a doubt, confirm the existence of these structures and further validate the combination of techniques used. Prof Yamamoto concludes: "Although graphene is a rather simple surface and we could expect more complicated water structures on other types of materials, our study provides a starting point for discussions of more realistic surface effects, and we expect it will lead to the control of surface properties."
Website: https://www.tus.ac.jp/en/mediarelations/
Takahiro Yamamoto has been with the Tokyo University of Science since 2003, when he joined as a research associate in the Department of Physics. Since then, he progressively climbed until obtaining the title of Professor at the Departments of Liberal arts (Physics) and Electrical Engineering. He now manages his own lab group, who focus on using quantum theoretical simulations to understand the physical properties of materials. In addition, he works at the Water Frontier Science and Technology Research Center, where he is the leader of a research group that aims to understand the properties of surface water through theoretical studies and simulations.
This work was supported in part by Grants-in-Aid for Exploratory Research (Nos. 17H02756 and 16H02079) from the Japan Society for the Promotion of Science (JSPS). This work was also supported by the Ministry of Education, Culture, Sports, Science and Technology (MEXT) under the Program for the Strategic Research Foundation at Private Universities, 2015-2019.
Japanese Journal of Applied Physics
10.7567/1347-4065/ab6564
Disclaimer: AAAS and EurekAlert! are not responsible for the accuracy of news releases posted to EurekAlert! by contributing institutions or for the use of any information through the EurekAlert system.
The Role of Water Network Chemistry in Proteins: A Structural Bioinformatics Perspective in Drug Discovery and Development
Affiliation.
- 1 Department of Pharmacoinformatics, National Institute of Pharmaceutical Education and Research, Niper Sas Nagar, India.
- PMID: 35894474
- DOI: 10.2174/1568026622666220726114407
Background: Although water is regarded as a simple molecule, its ability to create hydrogen bonds makes it a highly complex molecule that is crucial to molecular biology. Water molecules are extremely small and are made up of two different types of atoms, each of which plays a particular role in biological processes. Despite substantial research, understanding the hydration chemistry of protein-ligand complexes remains difficult. Researchers are working on harnessing water molecules to solve unsolved challenges due to the development of computer technologies.
Objectives: The goal of this review is to highlight the relevance of water molecules in protein environments, as well as to demonstrate how the lack of well-resolved crystal structures of proteins functions as a bottleneck in developing molecules that target critical therapeutic targets. In addition, the purpose of this article is to provide a common platform for researchers to consider numerous aspects connected to water molecules.
Conclusion: Considering structure-based drug design, this review will make readers aware of the different aspects related to water molecules. It will provide an amalgamation of information related to the protein environment, linking the thermodynamic fingerprints of water with key therapeutic targets. It also demonstrates that a large number of computational tools are available to study the water network chemistry with the surrounding protein environment. It also emphasizes the need for computational methods in addressing gaps left by a poorly resolved crystallized protein structure.
Keywords: Bioinformatics; Directionality; Drug discovery; Molecular Dynamics; Thermodynamics; Water network; water map.
Copyright© Bentham Science Publishers; For any queries, please email at [email protected].
Publication types
- Computational Biology*
- Drug Discovery
- Proteins / chemistry
- Water* / chemistry
share this!
April 12, 2024
This article has been reviewed according to Science X's editorial process and policies . Editors have highlighted the following attributes while ensuring the content's credibility:
fact-checked
peer-reviewed publication
trusted source
Novel hydrogel removes microplastics from water
by Shreya Gangwal, Indian Institute of Science
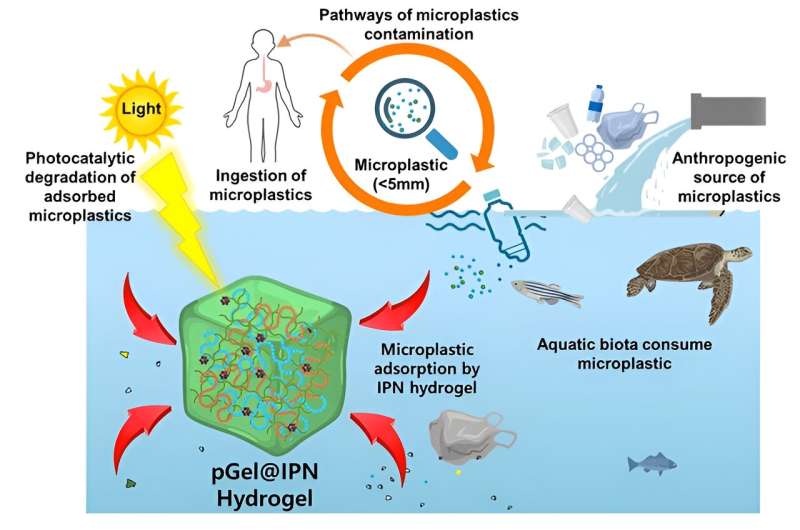
Microplastics pose a great threat to human health. These tiny plastic debris can enter our bodies through the water we drink and increase the risk of illnesses. They are also an environmental hazard; found even in remote areas like polar ice caps and deep ocean trenches, they endanger aquatic and terrestrial lifeforms.
To combat this emerging pollutant, researchers at the Indian Institute of Science (IISc) have designed a sustainable hydrogel to remove microplastics from water. The material has a unique intertwined polymer network that can bind the contaminants and degrade them using UV light irradiation. The research is published in the journal Nanoscale .
Scientists have previously tried using filtering membranes to remove microplastics. However, the membranes can become clogged with these tiny particles, rendering them unsustainable. Instead, the IISc team led by Suryasarathi Bose, Professor at the Department of Materials Engineering, decided to turn to 3D hydrogels.
The novel hydrogel developed by the team consists of three different polymer layers—chitosan, polyvinyl alcohol and polyaniline—intertwined together, making an Interpenetrating Polymer Network (IPN) architecture. The team infused this matrix with nanoclusters of a material called copper substitute polyoxometalate (Cu-POM).
These nanoclusters are catalysts that can use UV light to degrade the microplastics. The combination of the polymers and nanoclusters resulted in a strong hydrogel with the ability to adsorb and degrade large amounts of microplastics.
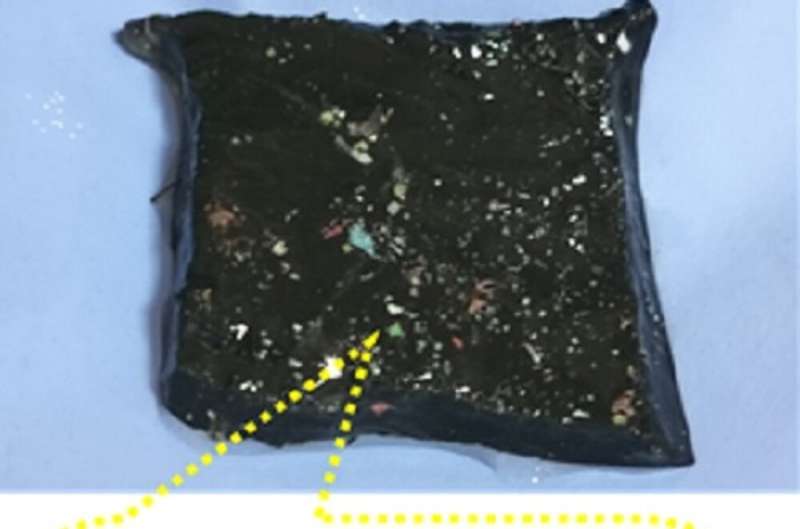
Most microplastics are a product of the incomplete breakdown of household plastics and fibers. To mimic this in the lab, the team crushed food container lids and other daily-use plastic products to create two of the most common microplastics existing in nature: polyvinyl chloride and polypropylene.
"Along with treatment or removal of microplastics, another major problem is detection. Because these are very small particles , you cannot see them with the naked eye," explains Soumi Dutta, first author of the study and SERB National Post-doctoral fellow at the Department of Materials Engineering.
To solve this problem, the researchers added a fluorescent dye to the microplastics to track how much was being adsorbed and degraded by the hydrogel under different conditions. "We checked the removal of microplastics at different pH levels of water, different temperatures, and different concentrations of microplastics," explains Dutta.
The hydrogel was found to be highly efficient—it could remove about 95% and 93% of the two different types of microplastics in water at near-neutral pH (∼6.5). The team also carried out several experiments to test how durable and strong the material was. They found that the combination of the three polymers made it stable under various temperatures.
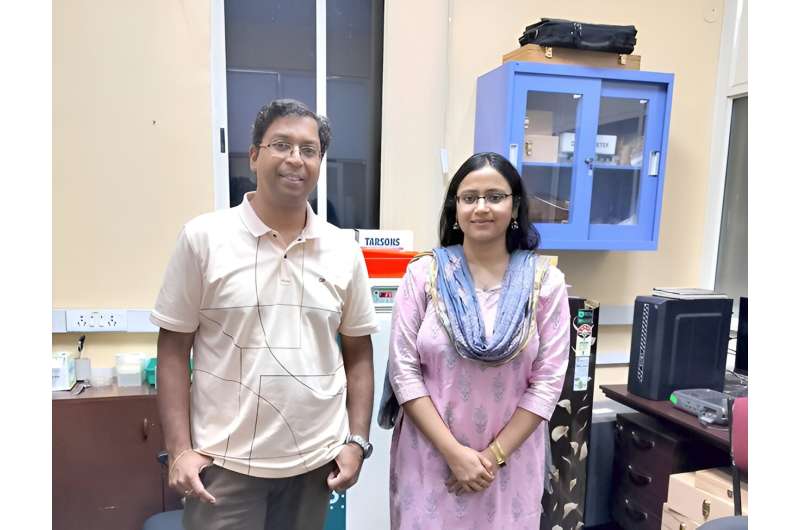
"We wanted to make a material that is more sustainable and can be used repetitively," explains Bose. The hydrogel could last for up to five cycles of microplastic removal without significant loss of efficacy. What's more, Bose points out, is that once it has outlived its use, the hydrogel can be repurposed into carbon nanomaterials that can remove heavy metals like hexavalent chromium from polluted water.
Moving forward, the researchers plan to work with collaborators to develop a device that can be deployed on a large scale to help clean up microplastics from various water sources.
Journal information: Nanoscale
Provided by Indian Institute of Science
Explore further
Feedback to editors

Why European colonization drove the blue antelope to extinction

Bumblebees don't care about pesticide cocktails: Research highlights their resilience to chemical stressors

Researchers discover new clues to how tardigrades can survive intense radiation

Nanovials method for immune cell screening uncovers receptors that target prostate cancer

Orbital eccentricity may have led to young underground ocean on Saturn's moon Mimas
2 hours ago

Researchers control quantum properties of 2D materials with tailored light

Study uses thermodynamics to describe expansion of the universe

Internet can achieve quantum speed with light saved as sound

New research highlights effects of gentrification on urban wildlife populations across US cities
3 hours ago

Machine learning could help reveal undiscovered particles within data from the Large Hadron Collider
5 hours ago
Relevant PhysicsForums posts
System curve and centrifugal pump suitability.
Apr 3, 2024
Maximum mass flow in a shell & tube heat exchanger
Air-to-fuel ratio of ethanol.
Mar 27, 2024
Isotopic exchange and SIMS diffusion profile measurement
Mar 20, 2024
Stainless Steel corrosion issue
Mar 5, 2024
H2O2 35% on Al 5083 at 150° gives 'limescale'
Mar 3, 2024
More from Materials and Chemical Engineering
Related Stories

Study finds microbes hitchhike on microplastics to reach the sea
Apr 9, 2024

Magnetic material mops up microplastics in water
Nov 29, 2022

A not so silver lining: Microplastics found in clouds could affect the weather
Nov 15, 2023

Bit by bit, microplastics from tires are polluting our waterways
Sep 5, 2023

Japanese scientists find microplastics are present in clouds
Sep 30, 2023

Researchers propose new method to identify label-free microplastics in crop plants
Jun 26, 2023
Recommended for you

A magnetic nanographene butterfly poised to advance quantum technologies
8 hours ago

A machine learning-based approach to discover nanocomposite films for biodegradable plastic alternatives
Apr 13, 2024

Laser-patterned thin films that swell into kirigami-like structures offer new opportunities in hydrogel technology
Apr 11, 2024

Defect removal of 2D semiconductor crystals: Trapping oxygen molecules offers greater control

A molecular moon lander: Insight into molecular motion on surfaces at the nanoscale

Unraveling the behavior of nanoconfined water and ice in extreme conditions
Let us know if there is a problem with our content.
Use this form if you have come across a typo, inaccuracy or would like to send an edit request for the content on this page. For general inquiries, please use our contact form . For general feedback, use the public comments section below (please adhere to guidelines ).
Please select the most appropriate category to facilitate processing of your request
Thank you for taking time to provide your feedback to the editors.
Your feedback is important to us. However, we do not guarantee individual replies due to the high volume of messages.
E-mail the story
Your email address is used only to let the recipient know who sent the email. Neither your address nor the recipient's address will be used for any other purpose. The information you enter will appear in your e-mail message and is not retained by Phys.org in any form.
Newsletter sign up
Get weekly and/or daily updates delivered to your inbox. You can unsubscribe at any time and we'll never share your details to third parties.
More information Privacy policy
Donate and enjoy an ad-free experience
We keep our content available to everyone. Consider supporting Science X's mission by getting a premium account.
E-mail newsletter
EDITORIAL article
This article is part of the research topic.
Dynamics at Surfaces: Understanding Energy Dissipation and Physicochemical Processes at the Atomic and Molecular Level
Editorial for Dynamics at Surfaces: Understanding Energy Dissipation and Physicochemical Processes at the Atomic and Molecular Level. Provisionally Accepted
- 1 Graz University of Technology, Austria
- 2 Swansea University, United Kingdom
- 3 Technical University of Munich, Germany
- 4 University of Surrey, United Kingdom
The final, formatted version of the article will be published soon.
The study by A. Sabik et al. investigates the surface dynamical motion of cobalt phthalocyanine molecules on silver using helium spin-echo (HeSE) spectroscopy, revealing that the activation energy for lateral diffusion decreases with temperature, leading to a transition from single jumps to predominantly long jumps at higher temperatures. It highlights the importance of considering a wide temperature range to capture the complete dynamics of molecular motion on surfaces. Using the same method, S. Kyrkjebø et al. illustrate a stark contrast in water mobility across graphenecovered and bare Ir(111) surfaces. On graphene-covered Ir(111), water molecules exhibit significantly hindered diffusion, attributed to the trapping at specific sites within the surface's corrugated structure. Their findings not only advance our understanding of water-surface interactions but also implicate potential impacts on the development of anti-icing and anti-corrosion materials. Via atom-surface scattering, P. Maier et. al. study the surface properties of 1T-TaS2 and TlBiTe2, contrasting electron-phonon coupling between these materials, and potential implications for phase transitions driven by phonons. The study of thermal expansion and interaction potentials offers valuable Figure 1. Energy dissipation processes are ubiquitous on surfaces and interfaces, from molecular motion to the formation of thin-films, determining adsorption, desorption and dissociation processes of molecules as well as the energetics upon molecular scattering from surfaces.insights into the complex behaviour of these compounds, contributing to a broader knowledge of charge-density wave systems and topological insulators. The scattering of keV protons through graphene is studied by J. Bühler et al. who challenge prior assumptions of the process. By incorporating the lattice thermal motion in simulations, they uncover that previously observed phenomena, such as the outer rainbow scattering, are artefacts of statistical averaging. At the same time, they illustrate new avenues for detailed studies of proton-graphene interactions and the orientations of graphene membranes. A. C. Dorst et al. study the recombinative desorption of O atoms from Ag(111) by combining ion imaging techniques with temperature-programmed desorption. The hyperthermal velocity distribution of the resulting O2 is consistent with activated recombinative desorption but lower than state-of-the-art calculations currently predict. These results, therefore, provide a valuable benchmark for refining theoretical models of metal oxidation processes. The influence of vibrational excitation on the sublimation of CO2 is investigated by C. Jansen et.al, where they use a laser to excite the antisymmetric stretch vibration (ν3) of the CO2 impinging on the CO2 ice. They report that exciting ν3 has a negligible effect on either the sticking of CO2 to the ice, or the resulting structure of the CO2 ice despite the additional vibrational energy being greater than the CO2 desorption energy. P. Floß et al. studied the surface-induced vibrational energy redistribution of methane scattering from Ni(111) and Au(111). Quantum state and angle-resolved measurements reveal a stark contrast in the vibrational energy conversion from ν3 to ν1 modes of methane, underlining the catalytic superiority of Ni( 111) over the more inert Au(111). It thus shows a direct correlation between surface-induced vibrational energy redistribution efficiency and catalytic activity. A. Tetenoire et al. elucidated the complex interplay between electrons and phonons in driving the photoinduced desorption and oxidation of CO on ruthenium surfaces. They demonstrate that phononic excitations play a pivotal role in CO desorption, while both electronic and phononic excitations significantly contribute to CO oxidation. Their research opens new avenues for optimising photochemical reactions on metal surfaces. Neubi F. Xavier Jr and co-workers investigate graphene nanoribbons (GNRs) as potential catalysts for catalytic methane decomposition using density functional theory. They find that armchair edges offer lower energy barriers for hydrogen desorption, compared to zizag edges on GNRs, indicating a better regeneration potential. Highlighting GNRs as comparable to metallic catalysts for methane decomposition, their research may pave the way for sustainable hydrogen production and emphasises the significance of nanomaterials in catalytic processes for green technology. The study by M. K. Prabhu and I. M. N. Groot demonstrates direct synthesis of metallic 1T Co-promoted MoS2 without intercalating agents via growth in a highly reducing environment. High-pressure in-situ reactor scanning tunnelling microscopy measurements, reveal the transformation from a disordered CoMoSx phase at low temperatures to crystalline 1T slabs at around 600 K. It highlights the importance of reducing conditions in materials growth thus avoiding the need of additional chemicals. In their mini review, H. Ueta et al. summarise recent studies on ortho-para conversion of hydrogen in molecular chemisorption and isolated matrix systems. These have found that nuclear-spin conversion can occur on a timescale of seconds, even for non-magnetic surfaces, and that the surface can provide a pathway for dissipating the accompanying change in rotational energy.The collection of 11 articles within this research topic, though only a fraction of the extensive work in the field, highlights that understanding energy dissipation and transfer at interfaces is an extremely active area of research being studied with state-of-the-art methods both experimentally and theoretically. The importance of understanding these surface dynamical processes at the molecular level, focusing on phenomena such as photoinduced reactions, vibrational energy redistribution, and molecular diffusion on surfaces cannot be overstated. Advancements in both experimental setups and theoretical models have opened up new avenues. For example, experiments include the dynamics of larger and more complex molecules and studies of more complex surfaces compared to flat metal substrates, including two-dimensional materials and heterostructures. Similarly, enhanced computing power and the utilisation of computational clusters have enabled more sophisticated ab initio calculations, incorporating phenomena like non-adiabatic effects and quantum friction (Alducin et al., 2017;Chadwick and Beck, 2017;Yu et al., 2023). Furthermore, the integration of machine learning approaches promises to refine theoretical analysis further (Jiang et al., 2016;Kapil et al., 2022). Thus, the studies do not only shed light on the underlying atomic-level interactions but also pave the way for optimising materials for specific technological applications, from optoelectronics to hydrogen production.However, challenges remain, e.g. in extending ab initio methods to larger systems and longer timescales and in conducting experiments under conditions that more closely mimic "real-life" parameters in catalysis to name a few (Yang and Wodtke, 2016). Moreover, while theory does well in studying specific nanosystems, there is still a need for experimental development to measure dynamical processes at tailored nanostructures or in confinement (Sacchi and Tamtögl, 2023;Yu et al., 2023). By overcoming these challenges and unravelling the mechanisms governing energy dissipation at interfaces, our community can unlock a new era of material fabrication and device control. For example, imagine designing catalysts with unparalleled efficiency, tailoring self-assembly processes for nanomaterial fabrication, or even manipulating environmental interactions on a molecular level. Future research will thus be pivotal for advancing various applications, including catalysis, energy production, and materials science by providing insights into the interaction mechanisms between molecules and surfaces, the influence of surface properties on these interactions, and the development of novel materials with enhanced functionalities.
Keywords: Surface chemistry, Catalysis, Ab initio (calculations), Energy Transfer, Scattering spectroscopy, Nanotechnology / nanomaterials, Thin film growth and stability, surface diffusion
Received: 03 Apr 2024; Accepted: 10 Apr 2024.
Copyright: © 2024 Tamtögl, Chadwick, Lechner and Sacchi. This is an open-access article distributed under the terms of the Creative Commons Attribution License (CC BY) . The use, distribution or reproduction in other forums is permitted, provided the original author(s) or licensor are credited and that the original publication in this journal is cited, in accordance with accepted academic practice. No use, distribution or reproduction is permitted which does not comply with these terms.
* Correspondence: Mx. Anton Tamtögl, Graz University of Technology, Graz, Austria
People also looked at
Thank you for visiting nature.com. You are using a browser version with limited support for CSS. To obtain the best experience, we recommend you use a more up to date browser (or turn off compatibility mode in Internet Explorer). In the meantime, to ensure continued support, we are displaying the site without styles and JavaScript.
- View all journals
- My Account Login
- Explore content
- About the journal
- Publish with us
- Sign up for alerts
- Open access
- Published: 03 February 2017
Ultrahigh-resolution imaging of water networks by atomic force microscopy
- Akitoshi Shiotari 1 &
- Yoshiaki Sugimoto 1
Nature Communications volume 8 , Article number: 14313 ( 2017 ) Cite this article
21k Accesses
100 Citations
15 Altmetric
Metrics details
- Atomic force microscopy
- Supramolecular chemistry
Local defects in water layers growing on metal surfaces have a key influence on the wetting process at the surfaces; however, such minor structures are undetectable by macroscopic methods. Here, we demonstrate ultrahigh-resolution imaging of single water layers on a copper(110) surface by using non-contact atomic force microscopy (AFM) with molecular functionalized tips at 4.8 K. AFM with a probe tip terminated by carbon monoxide predominantly images oxygen atoms, whereas the contribution of hydrogen atoms is modest. Oxygen skeletons in the AFM images reveal that the water networks containing local defects and edges are composed of pentagonal and hexagonal rings. The results reinforce the applicability of AFM to characterize atomic structures of weakly bonded molecular assemblies.
Similar content being viewed by others
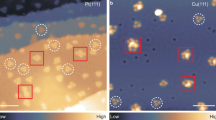
Identification of a common ice nucleus on hydrophilic and hydrophobic close-packed metal surfaces
Pengcheng Chen, Qiuhao Xu, … Nan Yao
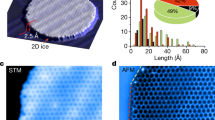
Atomic imaging of the edge structure and growth of a two-dimensional hexagonal ice
Runze Ma, Duanyun Cao, … Ying Jiang
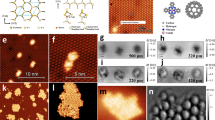
Precise determination of molecular adsorption geometries by room temperature non-contact atomic force microscopy
Timothy Brown, Philip James Blowey & Adam Sweetman
Introduction
Mechanisms of ice growth on metal surfaces are determined by the adsorption structure of the first water layer. Water in the first layer on the surfaces creates various hydrogen (H)-bonding networks, including one-, two- and three-dimensional (1D, 2D and 3D) structures, where the ice rules 1 are frequently broken. The water networks on well-ordered metal surfaces generally form periodic phases, which readily turn into other phases depending on the surface structures, temperature and coverage. Moreover, heterogeneous catalysis of metals facilitates dissociative reactions of water to yield hydroxyl (OH) groups, which further complicates the structures of the H 2 O–OH mixed layers. The configurations of the first water layers have been investigated with spectroscopic methods, such as vibrational spectroscopy, photoelectron spectroscopy and thermal desorption spectroscopy 2 , 3 , 4 . Although these methods are well-established and easily analysable, they average out wide-range information on the surface. Therefore, local structures (vacancies, impurities, dissociative products and layer edges), which may have different configurations and properties from the intact water networks, are generally undetectable or buried in the main peaks in the spatially averaged spectra. However, such local defects in water layers on metals play an important role in the catalysis and wettability; on copper (Cu) surfaces, for example, OH groups initiate a low-temperature water-gas shift reaction 5 , and behave as anchors for adsorbing onto the substrate 6 and as bonding sites for the second water layer 7 .
Scanning tunnelling microscopy (STM) is known to be a useful tool for investigating local structures in water networks at the single-molecule level. Since various H-bonding networks on metal surfaces have already been observed directly and characterized, STM brings innovative information to the experimental methodology of water–solid interfaces 3 , 4 , 8 , 9 , 10 , 11 , 12 . Hitherto STM was employed to trace thermal diffusion of individual water molecules 13 , induce dissociation of water 14 , 15 , 16 , fabricate H-bonding complexes 16 , 17 , 18 and control H-atom dynamics 16 , 17 , 18 , 19 , 20 , 21 on surfaces. In particular, recent STM studies visualized the intramolecular structures of water monomers and small clusters on an ultrathin NaCl film 18 and controlled the direction of H-bonds in the cluster 20 . On the other hand, it remains difficult to identify the atomic configurations of self-assembled water networks on metal surfaces by topographic STM images alone, and thus STM-based information is best supported with vibrational spectroscopy and/or theoretical calculations 7 , 22 , 23 . This is mainly because STM images reflect both electronic states near the Fermi level and geometries of the adsorbates.
As well as STM, non-contact atomic force microscopy (AFM) is a powerful option for visualization and analysis of surface structures 24 . Non-contact AFM has a remarkable range of applications, including atomic-resolution imaging of insulators 24 and chemical identification of individual atoms 25 ; however, water-layer studies using non-contact AFM have been notably scarce 26 . For aromatic molecules on surfaces, on the other hand, Gross et al . 27 have proposed that the spatial resolution of AFM can be significantly enhanced by using probe tips functionalized by molecules such as carbon monoxide (CO). Carbon skeletons in the molecules can then be visualized 27 owing to the sensitive detection of the repulsive forces between atoms in the adsorbate and the sharpened tip apex 28 . H bonds between organic molecules are also potentially visualized 29 , even though the results of these imagings remain controversial 28 , 30 . This imaging technique has already been applied to various organic molecules 31 , 32 and non-carbon materials such as iron clusters 33 and metal chalcogenide thin films 34 , 35 . In contrast to the rigid and stable materials, water molecules in the first layers would form various adsorption geometries differing from the monomeric configurations, owing to H bonds 16 , 18 , 22 , 36 . Therefore, it was unobvious whether AFM can/cannot non-destructively visualize atomic structures of the weakly bonded molecular assemblies.
Here, we aim to visualize water networks on a metal surface with non-contact AFM at much higher spatial resolution than in existing STM images. We focus on water adsorbed onto a Cu(110) surface, on which many different kinds of H-bonding networks can be formed 7 , 16 , 19 , 22 , 23 , 37 , 38 , 39 , 40 , 41 .We demonstrate the application of AFM with a functionalized probe tip to the visualization of water networks, including their defects, edges and domain boundaries.
Visualization of 1D water chains on Cu(110)
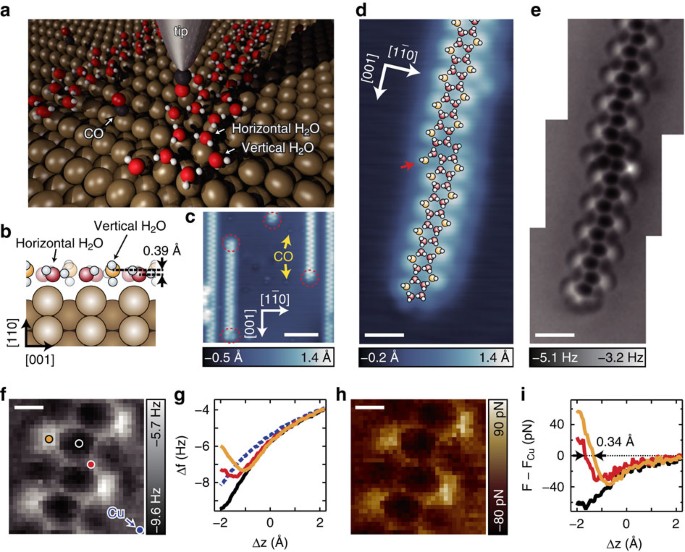
( a ) Schematic of STM/AFM measurement for pentagonal water chains on Cu(110) with a CO-terminal tip. Red, black, white and brown spheres show O, C, H and Cu atoms, respectively. ( b ) Side-view schematic of the water chain 22 . Red (yellow) spheres represent O atoms of horizontal (vertical) H 2 O. ( c ) STM image of the water chains on Cu(110) with a CO-terminal tip (sample bias V =30 mV, tunnelling current I =20 pA). The zigzag chains have terminals (red ellipses). ( d , e ) STM ( V =30 mV, I =20 pA) and AFM ( V =0 mV, oscillation amplitude A =2 Å) images, respectively, of a water chain including a kink and a terminal. An atomic structure of the chain is superposed in d . Note that the other possible structure is shown in Supplementary Fig. 5 . The tip height in e was set over the bare surface under the same conditions as in d . ( f ) Δ f map of the pentagonal chain at a tip height Δ z =−2 Å ( A =1 Å). ( g ) Δ f ( Δz ) curves recorded over the markers in f . ( h ) Force map of the chain at Δ z =−1.95 Å after subtraction of the force for the bare surface F Cu . ( i ) Force curves over the makers in f after subtraction of F Cu (Δ z ). Scale bars, 50 Å ( c ); 10 Å ( d , e ); 3 Å ( f , h ).
Figure 1c shows typical STM image of the water chains on Cu(110) at 4.8 K. By using a probe tip functionalized by a CO molecule 27 , 42 ( Fig. 1a ), the zigzag shape of the STM image is emphasized ( Supplementary Fig. 1 and Supplementary Note 1 ); however, the pentagonal bonding structure is still hardly discriminated. The zigzag chains have ‘terminals’ where the chain elongation along the [001] direction is ended (the red ellipses in Fig. 1c ), and ‘defects’ where the zigzag manner is locally broken. Some of the defects correspond to ‘kinks’ deflecting the 1D chains ( Fig. 1d ). An AFM image of the water chain with the CO-terminal tip ( Fig. 1e ) clearly reflects the atomic position of O atoms, in contrast with an STM image of the same area ( Fig. 1d ). For AFM, the frequency shift (Δ f ) was measured in constant-height mode 27 . An intact chain is observed as fused pentagonal rings in complete agreement with the model proposed previously ( Fig. 1a,b ). To identify the source of the observed structure, a 3D force map 43 was recorded over the pentagonal chain ( Fig. 1f–i ; see also Supplementary Fig. 2 and Supplementary Note 2 ). The imaging mechanism is consistent with that for organic molecules; the Pauli repulsion between the atoms on the surface and CO at the tip apex leads to high Δ f contrasts 27 . The zero-force distance for vertical H 2 O (the yellow curve in Fig. 1i ) is displaced by 0.34 Å towards the vacuum relative to that for horizontal H 2 O (red), because the O atom of vertical H 2 O is 0.39 Å more protruded than that of horizontal H 2 O (ref. 22 ), as shown in Fig. 1b . As a result of this displacement, vertical H 2 O is the most repulsive to the tip at a tip height Δ z of about −2 Å ( Fig. 1h,i ) and the Δ f intensity of vertical H 2 O at the tip height (the yellow curve in Fig. 1g ) is higher than that of horizontal H 2 O (red). On the other hand, the centre of a pentagon where no molecules exist (the black dot in Fig. 1f ) is still attractive at Δ z ≈−2 Å (the black curve in Fig. 1i ) due to attractive interactions of the surrounding molecules such as van der Waals forces.
Characterization of local defects in 1D water chains
The high-resolution AFM images enable each local defect in the 1D water chains to be characterized. Three kinds of terminals are mainly observed: a pentagonal ring (type i; Fig. 2a–c ), a pentagonal ring with an additional vertical H 2 O (type ii; Fig. 2d–f ) and two fused pentagonal rings (type iii; Fig. 2g–i ). The STM image of type i ( Fig. 2a ) is very similar to that of type ii ( Fig. 2d ), but the corresponding AFM images clarify the structural difference ( Fig. 2b,e ). We also find a small cluster imaged as tetraphyllous-shaped protrusions with STM ( Fig. 2j ). The corresponding AFM image ( Fig. 2k ) indicates that the cluster is composed of a hexagonal ring surrounded by four pentagonal rings, suggesting that it consists of 16 H 2 O molecules ( Fig. 2l ). Because the cluster is predominantly observed at relatively low coverages at 78 K ( Supplementary Fig. 6 ), this is a stable structure at the initial stage. This cluster has a similar structure to the terminal of type iii; at the terminal, the 1D chain is elongated from one of the four fused pentagonal rings, whereas the nearest neighbouring pentagonal ring is broken ( Fig. 2i ). This suggests that the ‘tetraphyllous cluster’ probably corresponds to a core for growing the 1D chain to yield a type-iii terminal. We also observed several kinds of defects and kinks, which are composed of fused pentagonal (and hexagonal) rings ( Supplementary Fig. 7 ). Note that OH groups are probably included in some of the fused hexagonal defects ( Supplementary Fig. 8 and Supplementary Note 5 ).
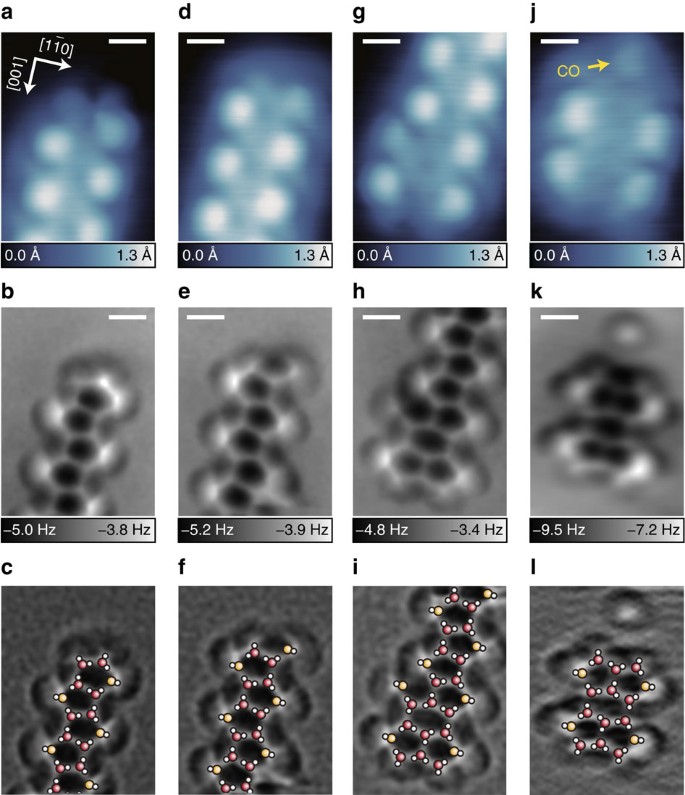
STM (top) and AFM (middle) images of terminals for the pentagonal water chains. An atomic structure of each terminal is superposed on the Laplacian-filtered AFM image (bottom). ( a – c ) Pentagonal terminal. ( d – f ) Pentagonal terminal with an additional vertical H 2 O. ( g – i ) Fused hexagonal and pentagonal terminal. ( j – l ) ‘Tetraphyllous cluster’ consisting of four pentagons. The images in a , b are magnified and 180°-rotated versions of those in Fig. 1d,e , respectively. The images in d , g were obtained at V =30 mV and I =20 pA, the image in j at V =50 mV and I =20 pA, the images in e , h at V =0 mV, A =2 Å, and Δ z =0 Å, and the image in k at V =0 mV, A =1 Å, and Δ z =0 Å. The tip height Δ z in e , h , k was set over the bare surface under the same conditions as in d , g , j , respectively. Scale bar, 5 Å.
High-resolution imaging of a water-hydroxyl network on Cu(110)
Even if H atoms are almost invisible, the distance between O atoms correlates highly with the strength of H bonds and can be sensitively detected by AFM with CO-terminal tips. To demonstrate that, we observed a H 2 O–OH mixed network on Cu(110), which was formed by partial dissociation of water after the sample was annealed at 160 K. At the temperature, OH groups are yielded by water dissociation and coexists with remaining H 2 O molecules 37 . The islands appear as ‘crooked bands’ elongated along the [001] direction ( Fig. 3a ; see also Supplementary Fig. 9 and Supplementary Note 6 ), which are quite similar to the appearance of H 2 O/O/Cu(110) at 155 K (ref. 41 ). However, the atomic structure of the bands was not resolved by STM (ref. 41 ). On the other hand, a CO-terminal tip enables the STM image to show a honeycomb structure ( Fig. 3a ). In the AFM image at the same region, each O atom is resolved ( Fig. 3b ). This island is composed only of hexagonal rings, whereas we also find several pentagonal rings remaining at the edges of another island ( Supplementary Fig. 9 ). Remarkably, the hexagonal rings (especially inside the island) in Fig. 3b seem to be asymmetric. The superposed lines in Fig. 3c show the apparent O–O bonds in the oxygen skeleton of the island. In the image, three O–O bonds (dotted lines; with the lengths of ∼ 4 Å) are much longer than the other bonds (solid lines; 2–3 Å). The inhomogeneous O–O distances originate from the mixture of intact H 2 O molecules and dissociative OH groups.
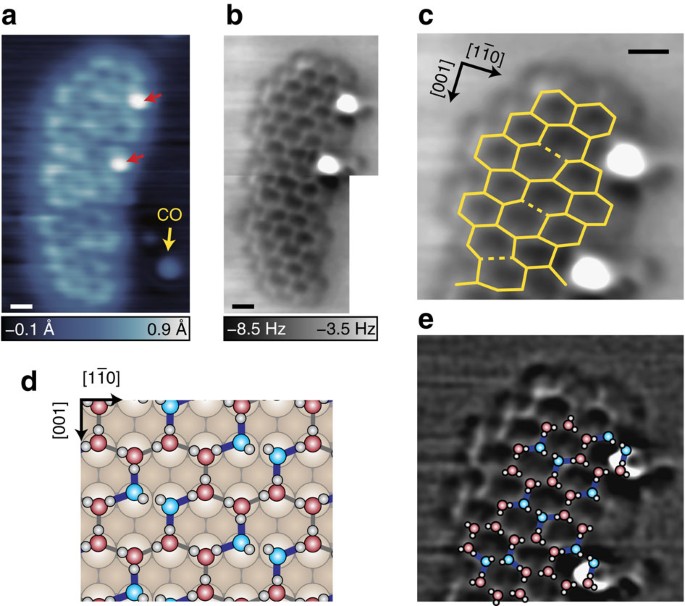
( a ) STM image of H 2 O/Cu(110) after annealing at 160 K ( V =50 mV, I =20 pA). The image was obtained at 4.8 K with a CO-terminal tip. ( b ) AFM image of the island in a ( V =0 mV, A =1 Å, Δ z =−1 Å). The tip height Δ z was set over the bare surface under the same conditions as a . ( c ) AFM image magnified at the upper part of b . The solid (dotted) lines represent the apparent O–O bonds with lengths of less (more) than 3.1 Å. ( d ) Schematic structure of the p (2 × 6) H 2 O–OH network on the surface 7 . ( e ) Proposed inside structure of the island superposed on the Laplacian-filtered image in c . Note that other possible structures are shown in Supplementary Fig. 5 . Scale bar, 5 Å.
We ascribe this structure to the p (2 × 6) network with a component ratio of 2 H 2 O:1 OH (refs 7 , 45 ; see Fig. 3d ). In the network, two OH groups face each other without H-bonds, namely, Bjerrum defects 7 . These defects have no H bond between the OH groups, whereas OH behaves as a good acceptor to provide strong H bonds with the two adjacent H 2 O molecules (blue lines in Fig. 3d ). Therefore, the hexagonal ring shows a long O–O side between the OH groups (at a distance of 3.2 Å), two short sides between H 2 O and OH (2.5 Å) and four middle-level sides between H 2 O molecules (2.7–2.8 Å). We assign the long O–O bonds (the dotted lines in Fig. 3c ) to Bjerrum defects. The direction of the long O–O bonds is partially oriented, corresponding to a domain boundary. We tentatively proposed the inside structure of the island including the boundary as shown in Fig. 3e . This assignment indicates that the apparent O–O bond length between the OH groups is 4.0±0.2 Å, that between H 2 O and OH is 2.5±0.3 Å and that between H 2 O molecules is 2.8±0.2 Å ( Supplementary Fig. 10 and Supplementary Note 6 ). Although the apparent bond lengths in AFM images with CO-terminal tips become exaggerated 46 , the bond distances are in good agreement with those in the theoretical model ( Fig. 3d ). According to the model, O atoms of both H 2 O and OH locate at an almost identical height along the surface normal 45 , which is also in good agreement with the similar appearance of the hexagonal vertices in the AFM image. The experimental image is comparable to our simulated AFM images based on the model in Fig. 3d , rather than those for the ‘H-down’ model consisting of H 2 O without OH (ref. 45 ; see Supplementary Fig. 11 and Supplementary Note 7 ).
In addition, this island has two discriminating protrusions (the red arrows in Fig. 3a ) probably corresponding to H 2 O in the second layer 7 . The structure proposed in Fig. 3e suggests that the second-layer molecules are located at the bridge site between OH and horizontal H 2 O. Because Bjerrum defects become an H-bonding acceptor for the second layer 7 , the admolecules are probably H-bonded to the lower OH group and oriented to the adjacent H 2 O.
Capture of an H-bonging recombination
Finally, we demonstrate a capture of an H-bonding rearrangement. Unlike covalently bonded organic molecules, H-bonding networks can be rearranged readily. High-resolution imaging of such ‘flexible’ structures allows us to trace the recombination of the bonding structure. The Cu(110) surface on which pentagonal 1D chains have been formed ( Fig. 1c ) was further exposed to H 2 O gas in a small amount at 6 K. The low-temperature dosing allows H 2 O molecules to adsorb onto the surface as isolated monomers which are observed as round protrusions 16 , 19 (the solid red arrows in Fig. 4a ). On the other hand, several molecules are attached to the 1D chains, which are imaged like knobs of the zigzag chain with STM (the dotted orange arrows). The AFM image in Fig. 4b shows that the additional H 2 O molecules are located at the vertices of the pentagonal rings, indicating that they are H-bonded to vertical H 2 O. During successive scanning, the additional H 2 O moved to the next vertical H 2 O ( Fig. 4c ). We note that the AFM images in Fig. 4b,c have scratching noises over the chains because another H 2 O molecule was attached to the tip apex together with CO and/or another H 2 O molecule (probably bonded onto horizontal H 2 O) diffused rapidly along the pentagonal chain. An interaction with the tip was probably responsible for the hopping motion of the attached molecules. As schematically shown in Fig. 4d , H 2 O monomers attached to vertical H 2 O are expected to be a horizontal configuration located on the trough between the Cu rows, which is different from the bonding site of isolated monomers (the atop site) 16 . Applying voltage pulses rarely induced the bonding of isolated monomers to the chains ( Supplementary Fig. 12 and Supplementary Note 8 ), which is compatible with the different configurations. This result implies that vertical H 2 O in the pentagonal chains acts as an ‘active site’ to trap a free H 2 O molecule. Above 150 K, the pentagonal unit with an additional H 2 O molecule may turn into a hexagonal ring ( Fig. 3 ) via partial dissociation to yield OH groups.
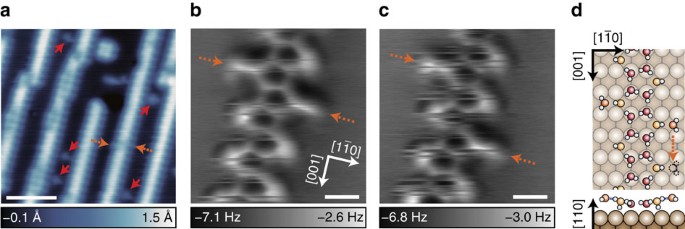
( a ) STM image of pentagonal H 2 O chains along with additional H 2 O monomers on Cu(110) with a CO-terminal tip ( V =50 mV, I =20 pA). The solid red (dotted orange) arrows show H 2 O monomers detached from (attached to) the chains. ( b , c ) AFM images of two H 2 O monomers attached to the chain ( V =0 mV, A =1 Å, Δ z =−0.5 Å). The images in b , c were obtained successively with a duration of 17 min per image. The tip height Δ z was set over the bare surface under the same conditions as in a . ( d ) Top-view (top) and side-view (bottom) schematic illustrations of a proposed atomic structure of a pentagonal chain with additional H 2 O monomers. Orange spheres represent O atoms of the attached H 2 O. Scale bars, 50 Å ( a ); 5 Å ( b , c ).
In summary, we observe H-bonding water networks and their defects with a combination of STM and non-contact AFM. In the first layer on Cu(110), water networks are constructed by pentagonal and hexagonal H-bonding units. In the water networks, the atomic structures of local defects, which are still rendered unspecifically with STM, can be well-characterized with AFM. We also demonstrate that rearrangements of readily convertible bonds, such as H-bonds, can be traced by high-resolution AFM imaging in real time. These results reinforce that the application of AFM with molecular functionalized tips to water networks constitutes a second breakthrough—after the application of STM—in the science of water–solid interfaces.
Experimental setup
The STM/AFM experiments were carried out in an ultrahigh-vacuum chamber (Omicron low-temperature STM/AFM system) at 4.8 K. A tuning fork with an etched tungsten tip was used as a force sensor 47 (resonance frequency f 0 =20.1 kHz, spring constant k 0 ≈1.8 × 10 3 N m −1 , quality factor Q ≈3 × 10 4 ). STM images were measured in constant-current mode. AFM measurements were operated in frequency-modulation mode with an oscillation amplitude A =1–2 Å. The frequency shift (Δ f ) was measured in constant-height mode at the sample bias V =0 mV.
Single-crystalline Cu(110) was cleaned by repeated cycles of Ar + sputtering and annealing to ∼ 600 °C. The probe tip was sometimes poked slightly into the clean surface so that its apex was coated with Cu atoms. Distilled water was purified by freeze-and-pump cycles. The clean surface was sequentially exposed to H 2 O gas at 78 K and CO gas at 8 K via a tube doser positioned a few centimetres away from the sample surface. To achieve high-resolution images, a CO molecule coadsorbed onto the surface was picked up to attach to the tip apex 27 , 42 .
3D force mapping
We obtained 1024 Δ f (Δ z ) curves for a pentagonal water chain on Cu(110) with a CO-terminal tip (32 point × 32 point; Fig. 1f ), as described in ref. 48 . Before the measurement of each curve, the tip was set over the yellow marker in Fig. 1f , and atom tracking was conducted with the tunnelling-current feedback loop closed (set point of V =50 mV and I =20 pA) in order to compensate for the thermal drift 49 . The origin of Δ z is defined by the tip height set at the tracking point. The positive (negative) value of Δ z means the tip height is further from (closer to) the sample than the set-point height. The force curve F (Δ z ) at each measurement point was calculated from the Δ f (Δ z ) curve by using the Sader formula 50 . The force curves are displayed after subtraction of the force curves obtained over the bare Cu surface F Cu (Δ z ) in order to clarify the short-range force distribution ( Fig. 1h ). The force maps are also displayed after subtraction of the force value for the bare surface at the same Δ z ( Fig. 1i and Supplementary Fig. 2 ).
Data availability
The data that support the findings of this study are available from the corresponding author on reasonable request.
Additional information
How to cite this article: Shiotari, A. & Sugimoto, Y. Ultrahigh-resolution imaging of water networks by atomic force microscopy. Nat. Commun. 8, 14313 doi: 10.1038/ncomms14313 (2017).
Publisher’s note: Springer Nature remains neutral with regard to jurisdictional claims in published maps and institutional affiliations.
Bernal, J. D. & Fowler, R. H. A theory of water and ionic solution, with particular reference to hydrogen and hydroxyl ions. J. Chem. Phys. 1 , 515–548 (1933).
Article ADS CAS Google Scholar
Thiel, P. A. & Madey, T. E. The interaction of water with solid surfaces: fundamental aspects. Surf. Sci. Rep. 7 , 211–385 (1987).
Henderson, M. A. The interaction of water with solid surfaces: fundamental aspects revisited. Surf. Sci. Rep. 46 , 1–308 (2002).
Hodgson, A. & Haq, S. Water adsorption and the wetting of metal surfaces. Surf. Sci. Rep. 64 , 381–451 (2009).
Gokhale, A. A., Dumesic, J. A. & Mavrikakis, M. On the mechanism of low-temperature water gas shift reaction on copper. J. Am. Chem. Soc. 130 , 1402–1414 (2008).
Article CAS Google Scholar
Yamamoto, S. et al. Hydroxyl-induced wetting of metals by water at near-ambient conditions. J. Phys. Chem. C 111 , 7848–7850 (2007).
Forster, M., Raval, R., Hodgson, A., Carrasco, J. & Michaelides, A. c (2 × 2) water-hydroxyl layer on Cu(110): a wetting layer stabilized by Bjerrum defects. Phys. Rev. Lett. 106 , 046103 (2011).
Article ADS Google Scholar
Verdaguer, A., Sacha, G. M., Bluhm, H. & Salmeron, M. Molecular structure of water at interfaces: wetting at the nanometer scale. Chem. Rev. 106 , 1478–1510 (2006).
Carrasco, J., Hodgson, A. & Michaelides, A. A molecular perspective of water at metal interfaces. Nat. Mater. 11 , 667–674 (2012).
Maier, S. & Salmeron, M. How does water wet a surface? Acc. Chem. Res. 48 , 2783–2790 (2015).
Björneholm, O. et al. Water at interfaces. Chem. Rev. 116 , 7698–7726 (2016).
Article Google Scholar
Guo, J., Bian, K., Lin, Z. & Jiang, Y. Perspective: structure and dynamics of water at surfaces probed by scanning tunneling microscopy and spectroscopy. J. Chem. Phys. 145 , 160901 (2016).
Mitsui, T., Rose, M. K., Fomin, E., Ogletree, D. F. & Salmeron, M. Water diffusion and clustering on Pd (111). Science 297 , 1850–1852 (2002).
Lauhon, L. J. & Ho, W. Inducing and observing the abstraction of a single hydrogen atom in bimolecular reactions with a scanning tunneling microscope. J. Phys. Chem. B 105 , 3987–3992 (2001).
Shin, H. J. et al. State-selective dissociation of a single water molecule on an ultrathin MgO film. Nat. Mater. 9 , 442–447 (2010).
Okuyama, H. & Hamada, I. Hydrogen-bond imaging and engineering with a scanning tunnelling microscope. J. Phys. D: Appl. Phys. 44 , 464004 (2011).
Kumagai, T. et al. H-atom relay reactions in real space. Nat. Mater. 11 , 167–172 (2012).
Guo, J. et al. Real-space imaging of interfacial water with submolecular resolution. Nat. Mater. 13 , 184–189 (2014).
Kumagai, T. et al. Direct observation of hydrogen-bond exchange within a single water dimer. Phys. Rev. Lett. 100 , 166101 (2008).
Meng, X. et al. Direct visualization of concerted proton tunnelling in a water nanocluster. Nat. Phys. 11 , 235–239 (2015).
Guo, J. et al. Nuclear quantum effects of hydrogen bonds probed by tip-enhanced inelastic electron tunneling. Science 352 , 321–325 (2016).
Carrasco, J. et al. A one-dimensional ice structure built from pentagons. Nat. Mater. 8 , 427–431 (2009).
Forster, M., Raval, R., Carrasco, J., Michaelides, A. & Hodgson, A. Water-hydroxyl phases on an open metal surface: breaking the ice rules. Chem. Sci. 3 , 93–102 (2012).
Giessibl, F. J. Advances in atomic force microscopy. Rev. Mod. Phys. 75 , 949–983 (2003).
Sugimoto, Y. et al. Chemical identification of individual surface atoms by atomic force microscopy. Nature 446 , 64–67 (2007).
Thürmer, K. & Nie, S. Formation of hexagonal and cubic ice during low-temperature growth. Proc. Natl Acad. Sci. USA 110 , 11757–11762 (2013).
Gross, L., Mohn, F., Moll, N., Liljeroth, P. & Meyer, G. The chemical structure of a molecule resolved by atomic force microscopy. Science 325 , 1110–1114 (2009).
Hapala, P. et al. Mechanism of high-resolution STM/AFM imaging with functionalized tips. Phys. Rev. B 90 , 085421 (2014).
Zhang, J. et al. Real-space identification of intermolecular bonding with atomic force microscopy. Science 342 , 611–614 (2013).
Lee, A. J., Sakai, Y., Kim, M. & Chelikowsky, J. R. Repulsive tip tilting as the dominant mechanism for hydrogen bond-like features in atomic force microscopy imaging. Appl. Phys. Lett. 108 , 193102 (2016).
Jarvis, S. P. Resolving intra- and inter-molecular structure with non-contact atomic force microscopy. Int. J. Mol. Sci. 16 , 19936–19959 (2015).
Altman, E. I., Baykara, M. Z. & Schwarz, U. D. Noncontact atomic force microscopy: an emerging tool for fundamental catalysis research. Acc. Chem. Res. 48 , 2640–2648 (2015).
Emmrich, M. et al. Subatomic resolution force microscopy reveals internal structure and adsorption sites of small iron clusters. Science 348 , 308–311 (2015).
Shiotari, A. et al. Local characterization of ultrathin ZnO layers on Ag (111) by scanning tunneling microscopy and atomic force microscopy. J. Phys. Chem. C 118 , 27428–27435 (2014).
Barja, S. et al. Charge density wave order in 1D mirror twin boundaries of single-layer MoSe2 . Nat. Phys. 12 , 751–756 (2016).
Chen, J. et al. An unconventional bilayer ice structure on a NaCl (001) film. Nat. Commun. 5 , 4056 (2014).
Andersson, K. et al. Molecularly intact and dissociative adsorption of water on clean Cu (110): a comparison with the water/Ru (001) system. Surf. Sci. 585 , L183–L189 (2005).
Yamada, T., Tamamori, S., Okuyama, H. & Aruga, T. Anisotropic water chain growth on Cu(110) observed with scanning tunneling microscopy. Phys. Rev. Lett. 96 , 036105 (2006).
Lee, J., Sorescu, D. C., Jordan, K. D. & Yates, J. T. Jr Hydroxyl chain formation on the Cu(110) surface: watching water dissociation. J. Phys. Chem. C 112 , 17672–17677 (2008).
Shi, Y., Choi, B. Y. & Salmeron, M. Water chains guide the growth of monoatomic copper wires on Cu(110). J. Phys. Chem. C 117 , 17119–17122 (2013).
Pang, Z. et al. Adsorption and reactions of water on oxygen-precovered Cu(110). J. Phys. Chem. C 120 , 9218–9222 (2016).
Bartels, L., Meyer, G. & Rieder, K.-H. Controlled vertical manipulation of single CO molecules with the scanning tunneling microscope: A route to chemical contrast. Appl. Phys. Lett. 71 , 213–215 (1997).
Albers, B. J. et al. Three-dimensional imaging of short-range chemical forces with picometre resolution. Nat. Nanotechnol. 4 , 307–310 (2009).
Yurtsever, A. et al. Understanding image contrast formation in TiO2 with force spectroscopy. Phys. Rev. B 85 , 125416 (2012).
Baghbanpourasl, A., Hingerl, K., Wippermann, S. & Schmidt, W. G. Copper(110) surface in thermodynamic equilibrium with water vapor studied from first principles. Surf. Sci. 612 , 82–89 (2013).
Gross, L. et al. Bond-order discrimination by atomic force microscopy. Science 337 , 1326–1329 (2012).
Giessibl, F. J. High-speed force sensor for force microscopy and profilometry utilizing a quartz tuning fork. Appl. Phys. Lett. 73 , 3956–3958 (1998).
Kawai, S., Glatzel, T., Koch, S., Baratoff, A. & Meyer, E. Interaction-induced atomic displacements revealed by drift-corrected dynamic force spectroscopy. Phys. Rev. B 83 , 035421 (2011).
Abe, M., Sugimoto, Y., Custance, O. & Morita, S. Room-temperature reproducible spatial force spectroscopy using atom-tracking technique. Appl. Phys. Lett. 87 , 173503 (2005).
Sader, J. E. & Jarvis, S. P. Accurate formulas for interaction force and energy in frequency modulation force spectroscopy. Appl. Phys. Lett. 84 , 1801–1803 (2004).
Download references
Acknowledgements
We thank H. Okuyama for valuable discussions. This work was supported by JSPS KAKENHI Grant Numbers JP15H06127, JP15H03566, JP16K13680, JP16H00933 and JP16H00959. A.S. acknowledges the support of the Kurita Water and Environment Foundation. Y.S. acknowledges the support of the Iketani Science and Technology Foundation, the Noguchi Institute, the Japan Association for Chemical Innovation and the Murata Science Foundation.
Author information
Authors and affiliations.
Department of Advanced Materials Science, The University of Tokyo, Kashiwanoha 5-1-5, Kashiwa, 277-8561, Japan
Akitoshi Shiotari & Yoshiaki Sugimoto
You can also search for this author in PubMed Google Scholar
Contributions
Both authors designed and planned the experiments, and discussed the results. A.S. performed the experiments and wrote the manuscript.
Corresponding author
Correspondence to Akitoshi Shiotari .
Ethics declarations
Competing interests.
The authors declare no competing financial interests.
Supplementary information
Supplementary information.
Supplementary Figures 1-12, Supplementary Notes 1-8 and Supplementary References (PDF 1713 kb)
Rights and permissions
This work is licensed under a Creative Commons Attribution 4.0 International License. The images or other third party material in this article are included in the article’s Creative Commons license, unless indicated otherwise in the credit line; if the material is not included under the Creative Commons license, users will need to obtain permission from the license holder to reproduce the material. To view a copy of this license, visit http://creativecommons.org/licenses/by/4.0/
Reprints and permissions
About this article
Cite this article.
Shiotari, A., Sugimoto, Y. Ultrahigh-resolution imaging of water networks by atomic force microscopy. Nat Commun 8 , 14313 (2017). https://doi.org/10.1038/ncomms14313
Download citation
Received : 17 November 2016
Accepted : 13 December 2016
Published : 03 February 2017
DOI : https://doi.org/10.1038/ncomms14313
Share this article
Anyone you share the following link with will be able to read this content:
Sorry, a shareable link is not currently available for this article.
Provided by the Springer Nature SharedIt content-sharing initiative
This article is cited by
- Pengcheng Chen
Nature Communications (2023)
Nanoscale one-dimensional close packing of interfacial alkali ions driven by water-mediated attraction
Nature Nanotechnology (2023)
Ultrahigh resistance of hexagonal boron nitride to mineral scale formation
- Kuichang Zuo
- Xiang Zhang
Nature Communications (2022)
Nanoscopic diffusion of water on a topological insulator
- Anton Tamtögl
- Marco Sacchi
- William Allison
Nature Communications (2020)
Water models for interfacial water simulations
Science China Technological Sciences (2019)
By submitting a comment you agree to abide by our Terms and Community Guidelines . If you find something abusive or that does not comply with our terms or guidelines please flag it as inappropriate.
Quick links
- Explore articles by subject
- Guide to authors
- Editorial policies
Sign up for the Nature Briefing newsletter — what matters in science, free to your inbox daily.

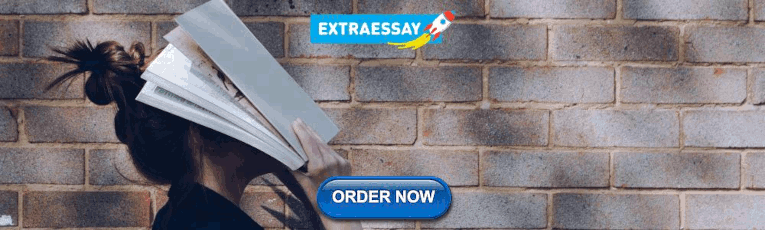
IMAGES
VIDEO
COMMENTS
"This research shows that we should really think of those trees and plants as towers of water holding sugars and proteins in place." Photo credit: Terry Ott For decades, the fields of physics and chemistry have maintained that the atoms and molecules that make up the natural world define the character of solid matter.
And several others, over the years, have indicated that water molecules exist in two or more "structures" and with a distribution of diverse hydrogen-bonding formations (e.g. Stillinger [57] in his Science paper - mostly 2 and 3 sites and Franck [58], also Henry Armstrong in 1920s [62], Oleg Samoilov in 1940s [63] and Wilse Robinson et al ...
Water molecules associate with each other relatively tightly. Therefore, H 2 O has relatively high values of surface tension, melting point, and boiling point. And, water has density anomalies that are manifested in ... His research has been on water statistical mechanics, the protein-folding problem, aspects of nonequilibrium statistical ...
Innovative Research Techniques. In a paper published today (January 15) in the journal Nature Chemistry, researchers from the University of Cambridge and the Max Planck Institute for Polymer Research in Germany show that ions and water molecules at the surface of most salt-water solutions, known as electrolyte solutions, are organized in a completely different way than traditionally understood.
The availability of drinking water is limited, and it is shrinking worldwide. By the year 2030, the world's 8.5 billion people 9 will consume 6 trillion cubic meters (6000 km 3) of water per year. 10 While today 11% of the global population lives with poor access to clean drinking water, 11 it is estimated that in 2030 half the world's population will be living under severe water stress ...
Water—at times individual molecules— facilitates enzyme catalysis and water molecules strongly bound to biomolecules impart thermodynamic stabilization to the latter 5.
In the hydrogen bond networks, over 95% of water molecules and over 60% of global box are involved in the formation of water clusters. Hence, the ring-based clusters can be regarded as specific ...
2.2.1 Water Molecule. It is well known that water consists of two elements, hydrogen (H) and oxygen (O). Two hydrogen atoms and one oxygen atom form a V-shaped structure (Fig. 2.3), which constitutes the water molecule (H 2 O). The experimental determination of the OH bond length of the gaseous free water molecule at room temperature and pressure is 0.9572 Å (1 Å = 10 -10 m), and the HOH ...
The interface can act to align water molecules, but the water molecules can act back on the interface, through both protonation and deprotonation, and dissociative adsorption at the interface 59 ...
The spectra shown in Figure 4 imply that the increasing concentration of KCl causes "blue shift", i.e., a shift towards the spectral range referring to the band of less hydrogen-bonded water molecules. Our findings, hence show a good agreement with the results of previous research [15,40,41,42].
A water molecule is made up of two hydrogen atoms connected by covalent bonds to one oxygen atom. Water molecules interact with each other through a type of interaction called hydrogen bonding. A tetrahedral arrangement of four water molecules around a central one is the key to understanding water. It helps to explain the structure of water in ...
Although the molecules of water are simple in structure (H 2 O), the physical and chemical properties of the compound are extraordinarily complicated, and they are not typical of most substances found on Earth.For example, although the sight of ice cubes floating in a glass of ice water is commonplace, such behaviour is unusual for chemical entities. . For almost every other compound, the ...
The key to understanding water's chemical behavior is its molecular structure. A water molecule consists of two hydrogen atoms bonded to an oxygen atom, and its overall structure is bent. This is because the oxygen atom, in addition to forming bonds with the hydrogen atoms, also carries two pairs of unshared electrons.
Water molecules are very cohesive because of the molecule's polarity. This is why you can fill a glass of water just barely above the rim without it spilling. Solid State (Ice) All substances, including water, become less dense when they are heated and more dense when they are cooled. So if water is cooled, it becomes more dense and forms ice.
June 4, 2021 — New research shows that when water comes into contact with an electrode surface all its molecules do not respond in the same way. This can dramatically affect how well various ...
The extraordinary life work of Dr. Emoto is documented in the New York Times Bestseller, The Hidden Messages in Water.In his book, Dr. Emoto demonstrates how water exposed to loving, benevolent, and compassionate human intention results in aesthetically pleasing physical molecular formations in the water while water exposed to fearful and discordant human intentions results in disconnected ...
Calculations showed that water self-ionization is more favourable in water clusters of 20 (ref. 167) or 21 (ref. 168) water molecules compared with bulk solution. This unexpected result is ...
Abstract. As an introduction to the eighth session 'Water and Biosystems' of the XVth 'Horizon in Hydrogen Bond Research', water molecules are presented with the stress on some elusive points which justify the existence of a session devoted to this topics. Thus, despite its familiar character, H 2 O is a molecule which remains still ...
Abstract. The water molecule with diverse properties is the most studied molecule of natural system. Various models have been designed to describe the molecular dynamics of water molecule and its ...
If a second layer of water molecules is added, the molecules from the first and second layers form 3D structures called tetrahedrons, which resemble a pyramid but with a triangular base. Curiously ...
Water molecules are extremely small and are made up of two different types of atoms, each of which plays a particular role in biological processes. Despite substantial research, understanding the hydration chemistry of protein-ligand complexes remains difficult.
The hydrogel was found to be highly efficient—it could remove about 95% and 93% of the two different types of microplastics in water at near-neutral pH (∼6.5). The team also carried out ...
We received fully calibrated flux data from SOFIA. The data are in the form of spectral images that are 248 × 248 pixels, where the x axis is wavelengths covering 5 to 8 µm and the y axis is the ...
The study by A. Sabik et al. investigates the surface dynamical motion of cobalt phthalocyanine molecules on silver using helium spin-echo (HeSE) spectroscopy, revealing that the activation energy for lateral diffusion decreases with temperature, leading to a transition from single jumps to predominantly long jumps at higher temperatures. It highlights the importance of considering a wide ...
In contrast to the rigid and stable materials, water molecules in the first layers would form various adsorption geometries differing from the monomeric configurations, owing to H bonds 16,18,22 ...