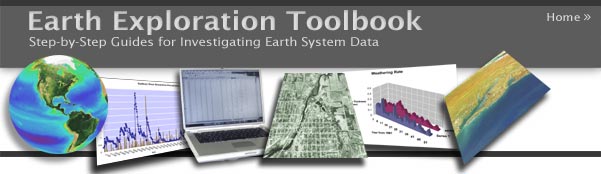
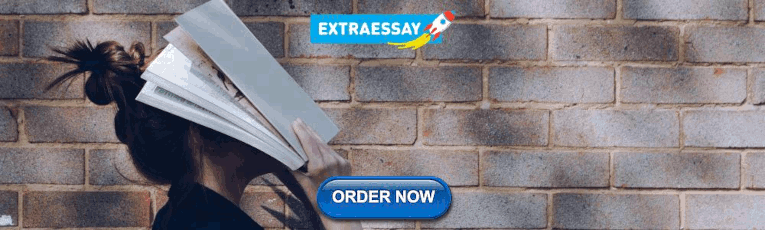
Case Study: Protecting Wetlands Through International Cooperation
Wetlands: unique ecosystems.
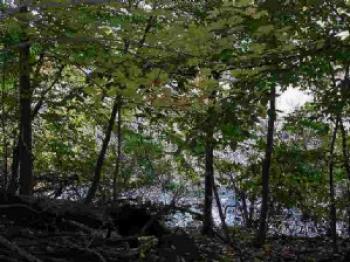
The U.S. Environmental Protection Agency and the U.S. Fish and Wildlife Service both offer additional information about wetlands as important wildlife habitats.
Wetlands around the World
New ways to study wetlands.
Wetland sites are commonly located in places that are hard to get to; scientists don't have the time or money to visit each site and collect data about it very frequently. Therefore, they are using satellite images to identify and monitor wetlands. NASA's Landsat program has been taking images of Earth's surface for over 25 years, and scientists have developed ways to monitor the long-term health of wetlands by examining them in these images. To see the range of studies that scientists are doing using satellite imagery, enter the terms "wetlands" and "Landsat" into an Internet search engine such as Google.
Selecting a Wetlands Site You want to Protect
As part of an Earth Day event, your environmental club decides that it might help protect a wetland site. To find an endangered wetland that everyone is interested in protecting, you'll use the Ramsar Wetlands Data Gateway, an online source of data about Wetlands of International Importance. The Gateway gives you the ability to identify wetland sites around the world based on a wide variety of characteristics, including the types of threats that endanger them. After you identify sites that meet the club's search criteria, you'll compare their characteristics and choose one site that you want to protect.
« Previous Page Next Page »
- Exploring Characteristics of Wetlands
- Teaching Notes
- Step-by-Step Instructions
- Tools and Data
- Going Further
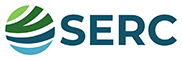
- About this Site
- Accessibility
Citing and Terms of Use
Material on this page is offered under a Creative Commons license unless otherwise noted below.
Show terms of use for text on this page »
Show terms of use for media on this page »
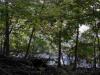
- None found in this page
- Last Modified: February 21, 2024
- Short URL: https://serc.carleton.edu/6290 What's This?
Click through the PLOS taxonomy to find articles in your field.
For more information about PLOS Subject Areas, click here .
Loading metrics
Open Access
Peer-reviewed
Research Article
Wetland biomass inversion and space differentiation: A case study of the Yellow River Delta Nature Reserve
Roles Writing – original draft
* E-mail: [email protected]
Affiliation School of Geography and Environment, Shandong Normal University, Jinan, China
- Mei Han,
- Bin Pan,
- Yu Bin Liu,
- Hao Zhe Yu,
- Yan Rong Liu
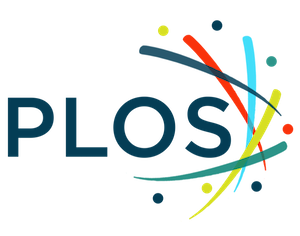
- Published: February 5, 2019
- https://doi.org/10.1371/journal.pone.0210774
- Reader Comments
With wetlands categorized as one of the three major ecosystems, the study of wetland health has global environmental implications. Multiple regression models were employed to establish relationships between Landsat-8 images, vegetation indices and field measured biomass in the Yellow River Delta Nature Reserve. These models were then used to estimate the spatial distribution of wetland vegetative biomass. The relationships between wetland vegetative biomass and soil factors (organic matter, nitrogen, phosphorus, potassium, water soluble salt, pH and moisture) were modeled. We were able to achieve higher correlations and improved model fits using vegetative indices and spectral bands 1–5 as independent variables. Several important soil factors were isolated, including soil moisture and salt concentrations, which affect wetland biomass spatial distributions. Overall, wetland biomass decreased from land to the ocean and from the river courses outward.
Citation: Han M, Pan B, Liu YB, Yu HZ, Liu YR (2019) Wetland biomass inversion and space differentiation: A case study of the Yellow River Delta Nature Reserve. PLoS ONE 14(2): e0210774. https://doi.org/10.1371/journal.pone.0210774
Editor: Arun Jyoti Nath, Assam University, INDIA
Received: September 29, 2016; Accepted: January 2, 2019; Published: February 5, 2019
Copyright: © 2019 Han et al. This is an open access article distributed under the terms of the Creative Commons Attribution License , which permits unrestricted use, distribution, and reproduction in any medium, provided the original author and source are credited.
Data Availability: The data used are accessed from the field survey and the remote sensing image in this paper is obtained from the URL: http://www.gscloud.cn/ . The data have also been uploaded on Fig share. One set is the field survey data ( https://figshare.com/articles/field_data/5446693 ), and another set is the remote sensing image ( https://figshare.com/articles/remote_image_rar/5355547 ).
Funding: This study was supported by the Natural Science Foundation of China NSFC General Projects (41371517) and the Scientific and Technological Project of Shandong Province (2013GSF11706) to MH. The URLs is http://www.nsfc.gov.cn/ and http://jihlx.sdstc.gov.cn/stdpms/ , respectively. The funders made the decision to publish.
Competing interests: The authors have declared that no competing interests exist.
Introduction
Wetlands, forests and oceans comprise the three most productive ecosystems on Earth. Although wetlands account for a relatively small proportion of total productivity, their contribution is nonetheless irreplaceable. Wetlands have therefore been described as "the kidney of the Earth" and "the species gene pool". The Ramsar Convention classifies wetlands as natural areas of marsh, peatland and forested swamps[ 1 ]. Wetland vegetation biomass incorporates the total mass of living vegetation within a given area, and this important index measures vegetation productivity and describes the circulation of material, energy flow processes, ecological function and ecosystem health within a wetland ecosystem[ 2 – 5 ].
The study of biomass can be traced back to the earliest research on forest litter and wood weight data, as conducted by the German scholar Ebemeyer in 1876, which was limited to specific tree species[ 6 ] Rapid development of forest biomass research occurred during the 20 th century at all spatial scales, from regional evaluation of biomass to the interactions between woodland soil microbes, fungi and nutrients.
Chinese scholars began studying forest biomass in the 1970s [ 7 ] The Sixth National Forest Resources Inventory mapped the distribution of vegetation at a scale of 1:1,000,000, thereby allowing the calculation of the spatial distribution of net primary productivity (NPP) based on Moderate Resolution Imaging Spectroradiometer (MODIS) inversion. Based on the determination of the aboveground and underground biomasses of plant communities in an alpine meadow of the Qinghai Tibetan Plateau over a span of four years [ 8 ] Yang et al. explored the correlations of carbon, nitrogen (N) and phosphorus (P) inputs with aboveground biomass[ 9 ]. Through the analysis of microbial respiration and micro-environmental factors of soil in the Shanghai Jiuduansha Wetland National Nature Reserve, Jia et al. found that microbial diversity and soil microbial biomass were the most significant factors affecting soil microbial respiration[ 10 ].
Since the 1990s, researchers have increasingly focused on studying wetland biomass using remote sensing and the accompanying statistical estimation models[ 11 – 14 ]. Valk et al. found that seasonal changes in water level and temperature affect all forms of vegetative biomass in the India Keoladeo National Park[ 15 ]. By using hyper spectral remote sensing, Mirik et al. developed an accurate model at 1 m resolution for modeling the vegetative biomass of pastures in the Yellowstone National Park[ 16 ]. Austin et al.[ 17 ] used non-metric multidimensional scaling on seven vegetative biomass types, thereby establishing that wetland hydrological conditions are the primary factors affecting vegetative biomass in a wetland in southeast Idaho. Fuller and Feng [ 18 , 19 ] used 10 years of remote sensing images and the normalized difference vegetation index (NDVI) to analyze the changes in vegetation structure for a wetland in the South Florida peninsula, with the results suggesting that increased saline intrusion associated with sea-level rise continues to reduce the photosynthetic biomass within freshwater and oligohaline marsh communities of the southeastern Everglades. Using data collected from 1998–2005 for a wetland in southern California, Daniels et al. [ 20 ] identified a strong correlation between Phragmites australis dry weight, density and aboveground biomass. Using multispectral remote sensing and non-parameter modeling, Güneralp and Filippi [ 21 ] estimated the biomasses of floodplain areas using stochastic gradient (SGB) and multivariate adaptive regression (SARS) analyses to obtain a more reliable result, which paved the way for research on regional and global scales. Using enhanced thematic mapper (ETM) and sampling data for Poyang Lake, Li and Liu [ 22 ] estimated the lake’s biomass using linear fitting and Albert projection.
By comparing HJ-1A charge-coupled device (CCD) satellite remote sensing and field measurement data for the Yellow River Delta (YRD), Fu et al. [ 23 ] was able to accurately estimate the fresh weight of Suaeda heteroptera Kitag., thereby providing a more efficient method to monitor and evaluate wetland biomass and ecological function. Using the YDR Nature Reserve as an experimental area, Gao et al. [ 24 ] achieved high-precision estimations of wetland vegetative biomass using multivariable linear regression modeling (MLRM).
At present, two remote sensing (RS)-based methods exist to estimate wetland biomass: optical and radar. Because RS is economical, readily available and easy to process, optical RS has been widely used. Radar RS can be used in a wider range of conditions, including during day or night in all types of weather, and has better penetration capabilities, which is advantageous when estimating vegetation biomass, particularly spectral bands L and C applied to forest land and low biomass vegetation wetlands, respectively. However, the factors most influencing wetland biomass can be divided into two categories: environmental factors and gene/phenotype factors. The former mainly includes temperature, moisture, light and soil properties, whereas the latter includes the number of species, species uniformity and spatial distribution, plant height, physiological regulation and carbon dioxide fixation. The dominant factors limiting biomass depends on specific circumstances.
In summary, research on wetland vegetative biomass has made the following progress: (1) Research methods have progressed from field sampling, drying and weighing to remote sensing and simulation modeling. Traditional methods of surveying wetland biomass have obvious limitations, including the manpower required and the limited spatial extend possible, whereas modern remote sensing technology can not only overcome these shortcomings, but can also objectively estimate wetland biomass through a full range, at a large spatial scale and through multi-angle remote sensing images; (2) The relationships between wetland vegetative biomass, hydrological conditions, climate and other factors are increasingly well understood. Wetland vegetation is an important part of the wetland ecosystem, and environmental factors are closely related to wetland biomass. The study of these factors can result in an improved quantitative understanding of the specific factors most influencing wetland vegetation biomass, thereby providing a scientific basis for the protection and restoration of wetland ecosystems. The majority of past studies explored the relationships of wetland biomass with hydrology and climate though outdoor sampling, monitoring and indoor testing, physical/chemical analysis and establishing regression models; (3) Biomass inversion models are now effective methods to study wetland biomass. By combining the traditional collection of sample data with the analysis of remote sensing images, wetland vegetation biomass can be more accurately estimated[ 25 – 27 ].
These advances have made it possible to study the relationship between vegetative biomass and environmental factors. Therefore, the present study used Landsat-8 imaging of the YDR Nature Reserve to evaluate the effect of soil organic matter (OM), total nitrogen, total phosphorus, total potassium (K), water soluble salt and soil reaction (pH) on wetland biomass to explore the correlation between the NDVI, difference vegetation index (DVI), ratio vegetation index (RVI) and spectral bands 1–6 with vegetative biomass. To provide scientific guidance and a reference for wetland biodiversity conservation and wetland restoration, we calculated total biomass and generated a biomass distribution map for the study wetland, following which we discuss how vegetative biomass is influenced by species diversity using the Simpson Diversity Index (SDI), Richness Index (RI) and Shannon Index. Finally, we analyzed the spatial distribution of biomass to explore the relationship between biomass and soil parameters.
Materials and methods
Study location.
Two sites within the YDR Nature Reserve were chosen for the present study. The YDR Nature Reserve forms part of the National Nature Reserve Administration of ShanDong Province, China. The chosen sites are located at the mouth of the Yellow River, one to the west of Laizhou Bay and the other to the north of Bohai Sea. The sites are separated from the Laodong Peninsula by the sea (37°35′–38°12′ N, 118°33′–119°20′ E). One site is located in the current Yellow River Estuary and covers an area of 104,500 ha, whereas the other is situated at the position of the Yellow River’s previous estuary before the river changed course in 1976, and covers an area of 48,500 ha ( Fig 1 ). The landforms of the YDR are complex, and can generally be divided into dryland, tidal flats and the subtidal zone. The study area has a semi-humid, monsoon climate. The annual average temperature is 11.9°C and annual average rainfall is 592.2 mm.
- PPT PowerPoint slide
- PNG larger image
- TIFF original image
https://doi.org/10.1371/journal.pone.0210774.g001
To begin with, we didn’t have any relevant permit numbers, but we have the name of permitting agency, that is the Yellow River National Nature Reserve Administration of ShanDong Province, China, which has the power to allow us enter the field and extract the soil samples. In addition, this research was funded by National Natural Science Foundation of China (41371517) and the Shandong Science and Technology Research Program (2013GSF11706), both of them are grant numbers. Moreover, we submitted the field investigation plan to the Yellow River National Nature Reserve Administration of ShanDong Province, China. Then, the Director of Scientific Research Department (Zhu Shuyu) and the Chairman of the Union (Lv juanzhang) verified and approved. After we obtained the permissions, we could extract the soil samples at the YDR Nature Reserve. And all the soil samples are extracted from the public land, which belongs to the Yellow River National Nature Reserve Administration of ShanDong Province, China.
Also, there are numerous endangered birds in the Yellow River National Nature Reserve. 7 species of birds are the first-grade State protection, such as rus japonensis , Otis tarda dows , Haliaeetus abbicuio albicilla , Ciconia bovciana and Mergus squamatus ,etc. Also, there is a national secondary protected plant: Glycine soja Sieb, et Zucc.
The locations were not affect the threatened species, the reasons are as follows:
- After careful consideration, all the locations were kept away from the species habitat and there are no endangered species in the sample range.
- Soil samples are collected in the field every October. During this period, some endangered species are not in the reserve because they come to the reserve only in November and December. Such as rus japonensis , Otis tarda dow , Ciconia bovciana .
- The soil depth of all samples is 10 cm, and the weight is 500 g, which is very small and has no effect on the endangered species environment.
- All sampling procedures were supervised by Director Zhu Shuyu of the Scientific Research Department of the National Nature Reserve Administration of the Yellow River Delta, Shandong Province, China.
Sample collection
Field samples (n = 43) were collected in August 2014 during the peak growth time of meadow and marsh biomass to identify the wetland growth status and obtain the highest correlation with remote sensing data. At each sampling location ( Fig 1 ), a square quadrat (1 m × 1 m) made of polyethylene pipe was randomly thrown and the geographic coordinates of the center of the square were recorded by means of a handheld global positioning system (GPS) receiver. In addition, biomass coverage, vegetation type and average vegetation height within the square were recorded. Fresh weight of the above-ground biomass was documented and one-third of the above-ground biomass was sealed in plastic bags. At each sampling location, a topsoil sample (0–10 cm) was collected and placed in a plastic bag.
Soil and biomass analysis
Biomass samples were initially oven-dried -at 80 °C for 8 h. They were further dried in a drying oven for 4 h and then the biomass dry weight was determined. Soil moisture was measured gravimetrically by means of a convection oven (105 °C). Furthermore, soil organic matter (OM) was determined through potassium dichromate titration [ 28 ] and soil pH was estimated in a 1:2 soil/water solution using a pH electrode. Nitrogen (N) concentration was determined using the Kjeldahl method and available phosphorus (P) was extracted using sodium bicarbonate and measured using photo-spectroscopy [ 29 ]. The amounts of water-soluble-salts were measured using filter evaporation.
Remote sensing image extraction and processing
The remote sensing images were captured using the Landsat-8 Operational Land Imager (OLI) on 20 July 2014. Since the study area shows relatively minor variations in topography, the remote sensing images were processed using radiometric calibration, fast line-of-sight atmospheric analysis of spectral hypercubes (FLAASH) atmospheric correction and irregular image cutting[ 30 ]. Remote sensing data extraction was based on the reflectance of each band and the vegetation indices. Among the 11 spectral bands of the images, spectral bands 1–6 and the NDVI, DVI and RVI indices were selected due to their accuracy in imaging of coastal biomass.
Biomass modeling
The RS retrieval model was established using the relationship between the vegetation index and biomass. A simple linear model could not accurately estimate biomass due to the influence of land use types, soil type and soil properties. Thus, before establishing the regression model, the influences of land use types, such as basic farmland, were removed from the vegetation coverage area to calibrate the results.
Using the Statistical Package for the Social Sciences (SPSS) software package, correlations between each remote sensing factor and vegetative biomass were calculated. The type of model chosen for each index was dependent upon the coefficient of determination. The highest correlation coefficient was then used to select the regression model from among the linear regression, nonlinear regression and multiple linear regression models.
Multi-dimensional linear regression modeling is superior to other evaluation models when eight factors (NDVI, DVI, RVI and spectral bands 1–5) rather than nine factors (by adding spectral band 6), are used as independent variables, and the coefficient of determination of R 2 = 0.69 was the highest achieved.
Because of the low precision of linear models, only multiple linear regression models were established. The prediction accuracy of the model was tested by retaining ten samples in the model. Average residual error coefficient has a negative relationship with predictive alignment of the model; therefore, an average residual coefficient of 0.09 and predicted inosculation of 85.85% indicates that the model precision is higher, and that the model can be used to simulate biomass in this area ( Table 1 ).
https://doi.org/10.1371/journal.pone.0210774.t001
Results and discussion
Vegetative biomass of the study area.
The multiple linear regression models were then input to the ArcGIS grid calculator to generate a map showing the spatial distribution of vegetative biomass dry weight divided into five classes, as according to natural break-point classification ( Table 2 ).
https://doi.org/10.1371/journal.pone.0210774.t002
The total estimated marsh and meadow biomass dry weight in the study area was 299,986 kg. Overall, an exponential inverse relationship between area extent and biomass quantity was evident ( Table 2 ; Fig 2 ). Most of the land (65.2%) fell within Class 1 with the lowest biomass, followed by Class 2 (17.3%), Class 3 (12.0%), Class 4 (4.6%) and Class 5 (0.9%). The areas with the most biomass were located at the edge of an artificial woodland and farmland. Class 1 biomass was primarily distributed in areas with poor water quality and soil salinity.
https://doi.org/10.1371/journal.pone.0210774.g002
Spatial distribution of vegetative biomass
Vegetative biomass decreased with distance from the Yellow River and increased with distance from the sea. For the Yiqianer Station, vegetative biomass dry weight generally decreased from southeast to northwest ( Fig 2 ). Areas of high biomass were located in the southern shrub swamps and marshes further from the coast and within wetland restoration areas where biomass growth is more influenced by human activities. Low biomass areas were located in the northern coastal muddy beaches and the ecological conservation areas. For the site of the previous river course, vegetative biomass dry weight decreased with distance from the riverbanks.
At Dawenliu Station, the course of the Yellow River from 1976–1996 and along the current river course, vegetative biomass was found to be dense ( Fig 2 ); however, the density of the former river course was found to be significantly higher than that of the latter river course. During the cut off in 1976–1996, the river deposits were mature and the groundwater level was relatively high. However, the latter course which formed after 1996 remains in an early stage of development and the groundwater level is lower. Furthermore, higher salt concentrations occur near the coast and soil moisture is higher near the current course of the Yellow River.
Effect of phragmites australis height on vegetative biomass
Feng and Zhao [ 19 ]found that the height of Phragmites australis ( P . australis ) in the YRD is directly proportional to water depth. To obtain more CO 2 during photosynthesis, the height of vegetation will increase relative to water depth; therefore, P . australis height can reflect water depth. As a result, the related features between P . australis height and biomass can indirectly reflect the relationship between water depth and P . australis biomass.
The correlation coefficients between plant height and P . australis fresh and dry weights are 0.48 and 0.78, respectively, whereas the same correlation coefficients for Suaeda salsa ( S . salsa) , a species of seepweed, are 0.28 and 0.19, respectively.
Effect of soil factors on vegetative biomass
The dry weight of P . australis was found to be negatively correlated with soil water soluble salt and soil pH, indicating that these factors limit P . australis growth ( Table 3 ). P . australis dry weight was positively correlated with P, OM and N, with the strength of the correlation from highest to lowest in that order, although soil moisture was found to have the highest positive correlations with P, OM and N. Since the YRD Nature Reserve is influenced by erosion and does not receive anthropological nutrient inputs, the soil layers are uniformly thin with little OM accumulation and low N and P concentrations. Therefore, N and P concentrations were not found to have a discernable correlation with P . australis dry weight. On the other hand, soil moisture and salt concentrations were highly correlated with P . australis dry weight. This illustrates that P . australis is well adapted to grow in weak alkaline soil environments.
https://doi.org/10.1371/journal.pone.0210774.t003
The dry weight of S . salsa was positively correlated with soil OM, N, P and pH and negatively correlated with water soluble salt and soil moisture. Since S . salsa is a halophyte and is adapted to alkaline soil, positive correlations between S . salsa dry weight and pH and soil moisture were evident. Soil water soluble salt concentrations are relatively high in many places near the coastal areas. Therefore, it is reasonable to assume a higher abundance of S . salsa would be found in regions with high concentrations of water soluble salt. However, in reality, since high soil moisture would also be found in areas with high water soluble salt concentration, the density and overall biomass of S . salsa is low.
Overall, the dry weights of P . australis and S . salsa were predominantly controlled by moisture, i.e., soil moisture was positively and negatively correlated with the growths of P . australis and S . salsa , respectively.
Effect of species diversity on vegetative biomass
The Simpson Diversity Index (SDI), Richness Index (RI) and Shannon Index (SI) are commonly used to classify species diversity. These diversity indices were positively correlated with wet and dry vegetation weight ( Table 4 ), with dry weight data showing higher correlation coefficients than fresh weight data. SI had the highest correlation with biomass weight, followed by SDI and then RI. Although SI accounts for the number of species, each species has equal weight. When the number of species and species distribution have little diversity, SI can accurately classify vegetative biomass.
https://doi.org/10.1371/journal.pone.0210774.t004
Using these techniques, the spatial distribution and correlations of several driving factors of wetland vegetative biomass in the YRD Nature Reserve was explored. Zeng et al. [ 31 ] showed that biomass varies with elevation; however, elevation changes were not accounted for since changes in topography of the study area are minor. Among environmental factors, P . australis height had the greatest effect on P . australis biomass and there was no significant effect of OM and P. Soil water had the greatest effect on S . salsa biomass and OM had no significant effect ( Fig 3 ). This shows that plant height and soil moisture are the key factors controlling P . australis and S . salsa growth, respectively. Although P . australis is well adapted to saturated conditions, S . salsa is less tolerant of salinity. The present study illustrated that not only the number of species, but also the spatial distribution of species is important for robust restoration and protection of biomass in the YRD.
https://doi.org/10.1371/journal.pone.0210774.g003
Using improved analysis techniques, stronger correlations between biomass dry weight and remote sensing data were obtained. Due to the unique hydrological relationship between soil and plants in wetlands, the actual biomass weight of perennial aquatic plants such as P . australis is lower than what field sampling can accurately reflect. Therefore, drying in the laboratory is a more accurate reflection of vegetative biomass in wetlands.
By employing multiple regression models, we were able to achieve stronger correlations and higher model fits with DVI, RVI, NDVI and spectral bands 1–5 as independent variables. The spatial distribution of vegetation dry weight was significantly correlated with various environmental factors, among which P . australis height had the greatest effect on P . australis biomass and P. Soil water had the greatest effect on S . salsa biomass, which illustrates that plant height and soil moisture are the key factors controlling P . australis and S . salsa growth, respectively, whereas both factors were positively correlated with vegetative biomass. At the same time, species diversity had a significant effect on biomass dry weight, thereby illustrating that the number of species and the evenness of the spatial distribution of species affect vegetative biomass. Thus, to promote the restoration and growth of biomass in natural areas, it is necessary to control soil moisture and salt concentrations to increase species distribution and biomass density.
Acknowledgments
This study was supported by the Natural Science Foundation of China (41371517) and the Scientific and Technological Project of Shandong Province (2013GSF11706).
- View Article
- Google Scholar
- 28. Bremner JM. Nitrogen-total. In: Sparks D. L. (Ed.), Methods of Soil Analysis, Part 3, Chemical Methods—SSSA Book Series no 5, Madison, Wisconsin. 1996; 1085–1121.
004 - Freshwater wetland management
Syllabus point.
Describe (AO1) the role of wetlands as a water resource.
Evaluate (AO3) the effectiveness of the management strategies that have been adopted in a major wetland.
Key Terminology
Define the terms above using your 'IB Geography. Course Book' by Cooke and Nagle, the command term posters in the classroom and the useful links below.
Useful Links
Activity one - describe.
In the past wetlands have been seen as swamps; breading grounds for mosquitoes and disease. Today their value is being recognised both in terms of their valuable biotic resource and as a prevention to flooding.
- Using the image under the syllabus points, what does a wetland look like?
- Name the five largest wetlands in the world.
- What are the main roles of a wetland?
- What types of flora and fauna survive in a wetland?
Useful Resources
- www.wetland.org
- www.defenders.org
Activity Two - Example
You will be developing a case study of a specific wetland but do remember that the emphasis is on the effectiveness of management strategies. You can also link this to competing demands for water as well as how agriculture can impact water sources. Things to include:
- Location map
- What type of wetland is it?
- Who uses the wetland area and for what purpose? How could this lead to conflict?
- What damage has been caused to the wetland?
- What management has been put in place? How successful has that management been? (Cost-benefit analysis)
There are two examples that you can choose from:
- Norfolk Broads, UK
- Kissimmee, USA
1 - Norfolk Broads
- 'Planet Geography' by Stephen Codrington Pages 332-340
2 - Kissimmee
- Undoing the damage to Florida's Kissimmee River
- South Florida Water Management District
- Wetland Restoration
- Disney and Wetlands
IB Geography Freshwater wetland management from geographypods

Paper Information
- Paper Submission
Journal Information
- About This Journal
- Editorial Board
- Current Issue
- Author Guidelines
International Journal of Ecosystem
p-ISSN: 2165-8889 e-ISSN: 2165-8919
2021; 11(2): 31-45
doi:10.5923/j.ije.20211102.01
Received: Apr. 15, 2021; Accepted: May 21, 2021; Published: Jul. 26, 2021
Best Practices of Wetland Degradation Assessment and Restoration in the Semi-Arid Areas. Case Study of Uganda
Bernard Barasa 1 , Moses Egaru 2 , Polycarp M. Musimami 3 , Paul M. Gudoyi 1 , Gertrude Akello 1
1 Department of Geography and Social Studies, Kyambogo University, Kyambogo University, Kampala, Uganda
2 International Union for Conservation for Nature, Kampala, Uganda
3 National Biodiversity Bank, Department of Environmental Management, Makerere University, Kampala, Uganda
Copyright © 2021 The Author(s). Published by Scientific & Academic Publishing.
Despite the harsh climate in the semi-arid areas of Uganda, anthropogenic activities continue to increase in many wetlands due to increasing human populations, causing incidences of degradation that require urgent restoration interventions. This study assessed wetland degradation and restoration in four wetland sites. To do this, the spatial- temporal extent and drivers of wetland use/cover changes and wetland degradation were determined, and the best steps, lessons, and principles of conducting wetland restoration were established. We used Sentinel-2 (2016-2019) and MODIS (2019) sensor images to estimate wetland changes and degradation status. Furthermore, focus group discussion, disaggregated by gender, was also conducted with representatives of the wetland user groups. The results revealed a reduction in the area under natural vegetation types compared to small-scale farming and built-up areas, between 2016 and 2019. This pattern was intensively driven by wetland cultivation, deforestation, occurrence of flash floods, and poor market linkages / price of yields. The consequential spatial extent of wetland degradation was generally high. To counteract this degradation, the best steps that ensued an effective wetland ecological restoration included conducting a baseline survey, stakeholder consultation, identification of reference sites, planning for future changes, implementation of restoration action plans, self-sustainability, and monitoring. During this exercise, the key lessons learned were to encourage equal participation of men and women, raise awareness, encourage restoration through income generation, and promote sustainability of restoration interventions. Therefore, if these practices are well implemented, they could increase the resilience of communities to climate change, enhance livelihood diversity and inform the processes of developing wetland management plans.
Keywords: Wetland degradation, Ecological restoration, Wetlands, Uganda
Cite this paper: Bernard Barasa , Moses Egaru , Polycarp M. Musimami , Paul M. Gudoyi , Gertrude Akello , Best Practices of Wetland Degradation Assessment and Restoration in the Semi-Arid Areas. Case Study of Uganda, International Journal of Ecosystem , Vol. 11 No. 2, 2021, pp. 31-45. doi: 10.5923/j.ije.20211102.01.
Article Outline
1. introduction, 2.1. study area, 2.2. spatial temporal extent and drivers of wetland use/cover changes, 2.3. status and scale of wetlands and degradation, 2.4. best practices and lessons learned in conducting wetland ecological restoration, 3.1. spatial temporal extent and drivers of wetland use/cover changes, 3.2. status and scale of wetland degradation, 3.3. ascertaining the best practices and lessons in conducting wetland ecological restoration, 4. discussion, 5. conclusions, acknowledgements.
Thank you for visiting nature.com. You are using a browser version with limited support for CSS. To obtain the best experience, we recommend you use a more up to date browser (or turn off compatibility mode in Internet Explorer). In the meantime, to ensure continued support, we are displaying the site without styles and JavaScript.
- View all journals
- My Account Login
- Explore content
- About the journal
- Publish with us
- Sign up for alerts
- Open access
- Published: 05 May 2024
Permanent areas and changes in forests, grasslands, and wetlands in the North European Plain since the eighteenth century—a case study of the Kościan Plain in Poland
- Beata Medyńska-Gulij ORCID: orcid.org/0000-0001-6969-4088 1 ,
- Krzysztof Szoszkiewicz ORCID: orcid.org/0000-0002-8196-333X 2 ,
- Paweł Cybulski ORCID: orcid.org/0000-0002-5514-5720 1 &
- Łukasz Wielebski ORCID: orcid.org/0000-0003-2923-7530 1
Scientific Reports volume 14 , Article number: 10305 ( 2024 ) Cite this article
330 Accesses
Metrics details
- Forest ecology
- Grassland ecology
- Sustainability
- Wetlands ecology
This study investigates the intricate and enduring interplay of historical events, human activities, and natural processes shaping the landscape of North European Plain in western Poland over 230 years. Topographic maps serve as reliable historical data sources to quantify changes in forest, grassland, and wetland areas, scrutinizing their fragmentation and persistence. The primary objectives are to identify the permanent areas of the landscape and propose a universal cartographic visualization method for effectively mapping these changes. Using topographic maps and historical data, this research quantifies land cover changes, especially in forest, grassland, and wetland areas. With the help of retrogressive method we process raster historical data into vector-based information. Over time, wetlands experienced a substantial reduction, particularly in 1960–1982, attributed to both land reclamation and environmental factors. Grassland areas fluctuated, influenced by wetland and drier habitat dynamics. Fragmentation in grassland areas poses biodiversity and ecosystem health concerns, whereas forested areas showed limited fluctuations, with wetland forests nearly disappearing. These findings highlight wetland ecosystems’ sensitivity to human impacts and emphasize the need to balance conservation and sustainable development to preserve ecological integrity. This study advances landscape dynamics understanding, providing insights into historical, demographic, economic, and environmental transformations. It underscores the imperative for sustainable land management and conservation efforts to mitigate human impacts on ecosystems and biodiversity in the North European Plain.
Similar content being viewed by others
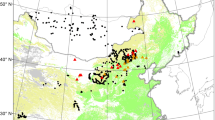
Agricultural development has not necessarily caused forest cover decline in semi-arid northern China over the past 12,000 years
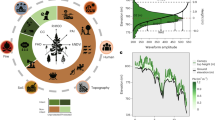
Human fingerprint on structural density of forests globally
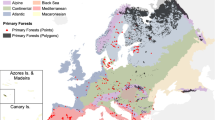
European primary forest database v2.0
Introduction.
Land cover plays a significant role in the environment and human life, determining the landscape and character of an area, the species of plants and animals inhabiting a given territory, as well as the economic 1 , 2 and touristic 3 , 4 potential of the lands. Human activity, along with the development of settlements, agriculture, and later industry, has led to transformations in the natural landscape 5 , 6 . The intensification of this intervention is also facilitated by population growth and technological advancement. Humans alter the landscape to accommodate their needs, and with the advancement of knowledge, this can be done in a more responsible and sustainable manner.
It is widely accepted that the analysis of alterations in land cover since the pre-industrial era could reveal pivotal events in Land Use and Land Cover (LULC) changes, particularly evident in the context of long-term change studies derived from topographical sources 7 , 8 . The analysis of LULC changes holds significance as it serves as a vital indicator required for the realization of the United Nations' Sustainable Development Goals (SDGs) 9 , 10 , 11 . LULC change studies based on topographic materials often focus on several elements such as grasslands, forests, urban areas, or arable land 12 , 13 , 14 .
In the context of Forest Transition Theory 15 , 16 , the forest lands in the European Plains were cleared for agricultural purposes 17 , 18 , while the remaining areas were heavily managed for timber production 19 , 20 . As a result, many countries in the region have implemented policies and programs aimed at promoting sustainable forest management practices, conserving biodiversity, and protecting forest ecosystems 21 , 22 , which might be recognized as a reversal of the deforestation trend 23 .
To track changes in Land Use and Land Cover (LULC) and incorporate them into a specific theoretical trend, methods based on the analysis of topographic maps are employed, as observed in the case of mountain landscapes 24 , 25 , 26 , 27 , 28 . Some research has focused on parts of the European Plain 29 , 30 , 31 , 32 incorporating grasslands that have consequently disappeared and fragmented due to agricultural development 33 , 34 .
In recent years, the drainage of wetlands for agriculture has slowed down in Europe, but the wetlands continue to face threats from urbanization and other forms of development 35 , 36 as well as climate change 37 . However, it is rare to find scientific research that examines the wetlands, their changes, and stability in Greater Poland based on topographic materials from the eighteenth century 38 , 39 . These processes might lead to rapid and abrupt changes, resulting in a regime shift that limits the possibility of predicting future changes 40 , 41 .
Therefore, the question arises: Have there been such significant changes in the area of forests, meadows, and wetlands over the past 230 years on the Kościan Plain that their continuity has been interrupted?
Cartographic visualizations depicting changes in land cover can assume two distinct approaches 42 . The first option entails an analytical approach, where the presentation method and data granularity allow for a detailed investigation of changes. Such visualizations can be challenging to interpret, and their users are typically experts who utilize them as tools for studying and understanding the phenomenon 43 . In such cases, geographic visualization (geovisualization) is often employed, involving interactive tools to enhance analysis efficiency 44 , 45 , 46 , 47 . The second approach involves simplifying the data, making the information more easily communicable in a graphical manner, thereby reaching a broader audience.
Another research question that arises is: How can cartographic visualization reveal permanent landscape features since the eighteenth century using topographic maps?
The goal of this study is to assess changes in forest, grassland, and wetland area and recognizing permanent areas in the Kościan Plain in Poland on the European Plain over the past 230 years on a topographical scale, using topographic maps. Specifically, we aim to assess changes in forest, grassland, and wetland area, their fragmentation, and durability. We also propose a universal method for cartographic visualization to support the visualization of changes in forest, grassland, and wetland areas in the North European Plain.
The main research hypothesis posits that the existence of permanent areas within grasslands and forests on the Kościan Plain has played a crucial role in preserving these landscapes over the past 230 years. These permanent areas, coupled with the conservation of drainage canals, have been instrumental in sustaining substantial portions of permanent grasslands and forests despite landscape alterations. The second research hypothesis is that the cartographic method proposed in a local study will allow determining the location and area of forests, grasslands and wetlands that have a permanent character. Unlike other studies of this type that have been mentioned here, we would also like to include wetlands in the landscape analysis. Wetlands are not a common element of analysis based on cartographic materials. Moreover, we aim to supplement this type of research with a universal method of cartographic presentation that can be employed regardless of the type of landscape.
Materials and methods
The Kościan Plain is a significant geographic region within the context of the North European Plain, located in western Poland (Greater Poland, Wielkopolska), covering an area of 194 km2, as illustrated in Fig. 1 . It is distinguished by its relatively flat topography, fertile soils, and a mosaic of land cover types, including forests, grasslands, and wetlands. This region plays a crucial role in the broader North European Plain, extending from the southern coast of the Baltic Sea to the Alpine foothills in the south.
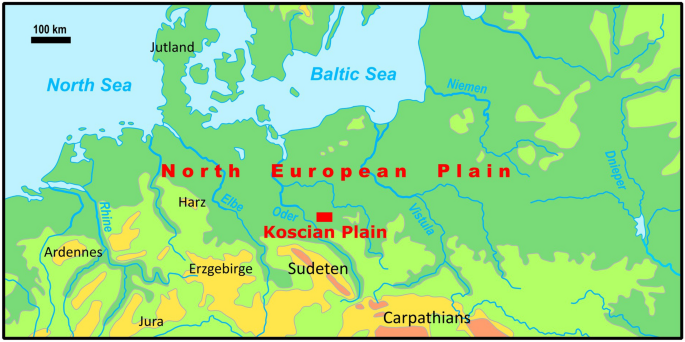
Localization of the Kościan Plain on the North European Plain.
Representativeness of the Kościan Plain for the North European Plain in the context of cartographic materials results from access to credible and continuous cartographic material (including the first professional topographic maps created in the eighteenth century) compared to similar consistent materials, e.g., Lower Saxony, Brandenburg, the Netherlands, Greater Poland, and Mazovia 48 , 49 , 50 , 51 . The territorial limitation of our research to the shape and size of map sheet and regular sheet division for topographic map aimed to propose a universal methodological approach to each fragment of the North European Plain, as its elementary part.
Forests have been an essential feature of the Kościan Plain’s landscape, contributing to its ecological diversity and providing numerous ecosystem services. The changes in land cover in the Greater Poland (Wielkopolska) were documented the impact of deforestation for agricultural expansion and urban development 39 . Additionally, the effects of afforestation efforts on restoring forest ecosystems and their implications for the spread of the pollutions in ground waters and in soils were investigated 52 .
Due to changes in land cover, some grasslands, which have a permanent character 53 , not only reduce their surface area, but also their functionality 54 . Historically, grasslands have been an important part of Poland’s landscape, providing habitat for a wide variety of plant and animal species 55 , 56 . However, in recent years, many grasslands have been lost or degraded due to changes in land cover, such as the conversion of grasslands to agriculture or urban areas, and the abandonment of traditional land management practices 57 . In addition, the loss of grasslands can also have negative impacts on soil quality, water retention, and carbon storage 58 , 59 . To address these challenges, efforts are underway in Poland to protect and restore grasslands 60 . Grasslands have also been a crucial component of the Kościan Plain’s land cover. Traditionally used for sustainable agriculture and livestock grazing, grasslands have faced significant transformations due to agricultural intensification and changes in land management practices 61 . They also had the flood protection function. Research by Kozaczyk 62 examined the water regulation impact on the permanent grasslands and their exploitation.
Historically, large areas of wetlands were drained for agricultural purposes, particularly in the Netherlands, Germany from the sixteenth century, and later in also Poland 63 . In western Poland, land reclamation was carried out on a small scale in the nineteenth century and on a much larger scale in the twentieth century, especially in the 1960s and by the early 1980s 62 , 64 . Wetlands, including marshes and peatlands, were once abundant in the Kościan Plain, contributing to water regulation, carbon sequestration, and wildlife habitat. However, Mizgajski 65 highlighted the alarming decline of wetlands in the region due to drainage and land reclamation for agricultural purposes, especially in the nineteenth century. This has raised concerns about the loss of valuable wetland ecosystems and the potential implications for flood control and water quality.
Understanding the changes in forest, grassland, and wetland cover in the Kościan Plain is essential in the context of broader European Plains. It allows us to assess the impacts of land cover changes on regional biodiversity, ecosystem services, and environmental sustainability. The integration of this regional knowledge into broader European studies, such as those conducted by Wulf and Gross 66 on pan-European land cover changes, enables a comprehensive understanding of the complex interactions between human activities and the natural environment across the continent.
For research on land cover in the European Plain for Western Poland, there is high potential but still unexplored.
Manuscript topographical maps from the second half of the eighteenth century constitute a unique documentation of pre-industrial space in Europe. This uniqueness results not only from the fact that these are the first cartographic records occurring in a single copy, but also from the fact that they are based on direct field observations. Field mapping was executed through table sketches using surveying tools and observations 49 . Due to their specific cartometric nature and interpretational possibilities, these works have been the subject of few studies until now 48 , 50 , 67 .
Table 1 presents a set of source maps with the following information: map name, publication year/years, scale, author/institution, provenance/owner, and publication technique. The year associated with each map in Table 1 indicates the approximate year in which forest, meadow, and wetland areas were mapped.
Figure 2 depicts the appearance of individual maps listed in Table 1 covering the study area (A-H) at a reduced scale.
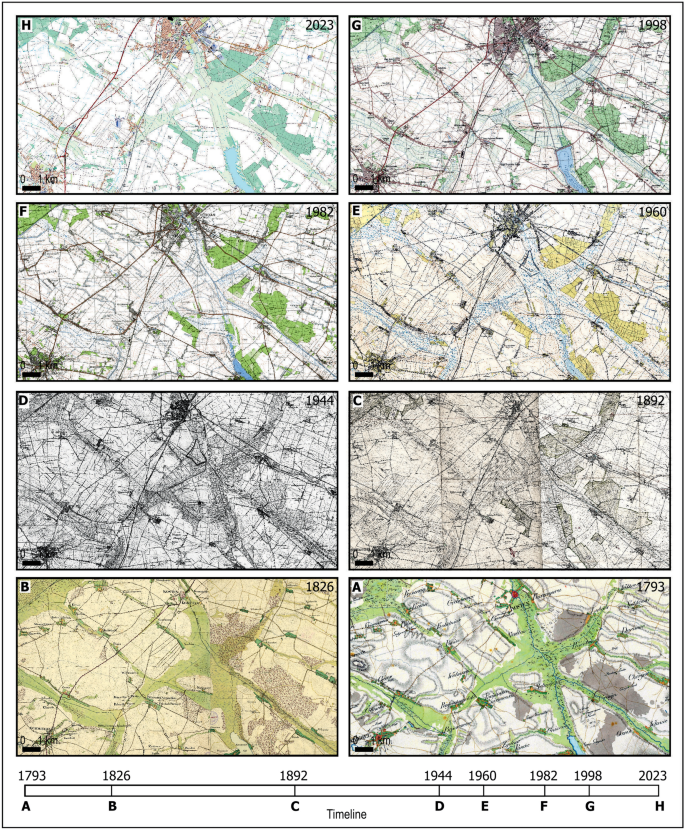
Topographic maps of Kościan Plain from 1793 to 2023, according to the description in Table 1 . An illustrative timeline is provided below the maps, aiding in conceptualizing the time intervals that separate various land cover states recorded in the source cartographic materials.
Visualization and data processing
Our method of result visualization will be a cartographic presentation method, which results from the overlapping of areas. Distinct extents represent wetland areas. The preparation of this type of visualization is preceded by data acquisition through the retrogressive method.
The retrogressive method in landscape studies involves examining landscapes by retracing steps from a more recent time period back to progressively older periods 68 , 69 , 70 , 71 . In cartographic studies, the retrogressive method is an approach that involves the analysis and interpretation of historical maps, charts, or aerial photographs to understand changes in land cover and landscape over time 72 . This method allows cartographers and researchers to examine past land cover patterns and identify historical trends and transformations in the landscape 73 , 74 . While older cartographic materials may lack the precision of modern surveys, they provide crucial baseline information for assessing historical land cover patterns and natural features with the use of modern GIS software 75 , 76 .
The foundation for the retrogressive vectorization of forest, grassland, and wetland from paper maps is georeferencing their scanned raster form to the current coordinate system to achieve the lowest possible root-mean-square error (RMSE), which, in the case of topographic maps, is approximately 1–2 mm on a paper map 77 . Considering the georeferencing methods of old maps 75 , we applied first-degree affine transformation to the currently valid UTM (Universal Transverse Mercator) coordinate system for topographic maps, zone 33, EPSG: 32,633 (Table 1 ).
Figure 3 illustrates an example of the retrogressive georeferencing process for a map from 1826. It is visible on the left side, with control points overlaid that correspond to identical, identifiable points on the contemporary reference map with spatial context. In this case, the same road intersections were identified on both maps.
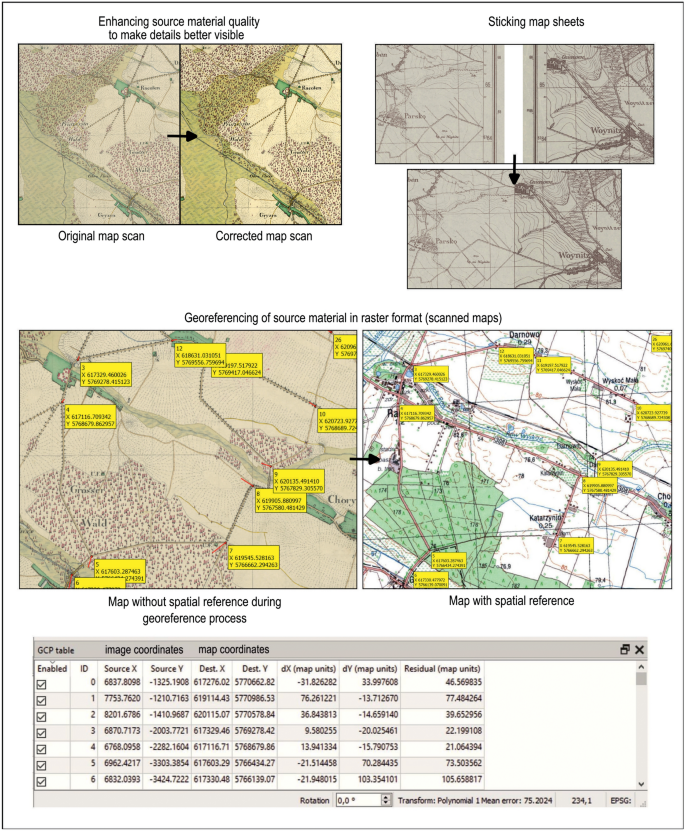
Stages of source data processing: Map color correction, map sheet integration, retrogressive georeferencing.
Based on the harmonization of the existing legends for the six newest maps from 1892 to 2023, the construction of the legend for the oldest map from 1793 48 , 49 , 51 , 67 and supplementation with cartographic signs not appearing in the legend but visible on the map from 1826 78 , 79 , the following land cover categories were defined for research: forest, grassland, wetland, wet forest, wet grassland.
For vectorization, objects from the previous state were utilized to retain shapes where there were no changes in the defined land cover boundaries or to modify only a portion of an object's shape. In order to preserve topological rules during the vectorization process, appropriate settings in GIS software were enabled, including snapping options such as topological editing, snapping tolerance, or prevention of polygons overlapping (“avoid overlap”). The layers were automatically checked by GIS software to identify features that could potentially have invalid geometry. Any such errors were subsequently fixed.
The use of appropriate cartographic visualization allowed to reveal those spatial relationships that would not be visible on individual maps. For this reason, in Fig. 4 , we can observe the elements of the landscape that have undergone spatio-temporal changes and those that have remained unchanged for 230 years, starting from 1793. Firstly, we can distinguish a large area of grasslands, which are located in the main line of canals and rivers. Secondly, we can distinguish large patches of forest in the vicinity of Kościan, which also in their core have not changed over this time. Both in the case of grasslands and in the case of forests, the core of the area has remained unchanged, while a certain envelope of these areas can be distinguished, which has fluctuated over the years.
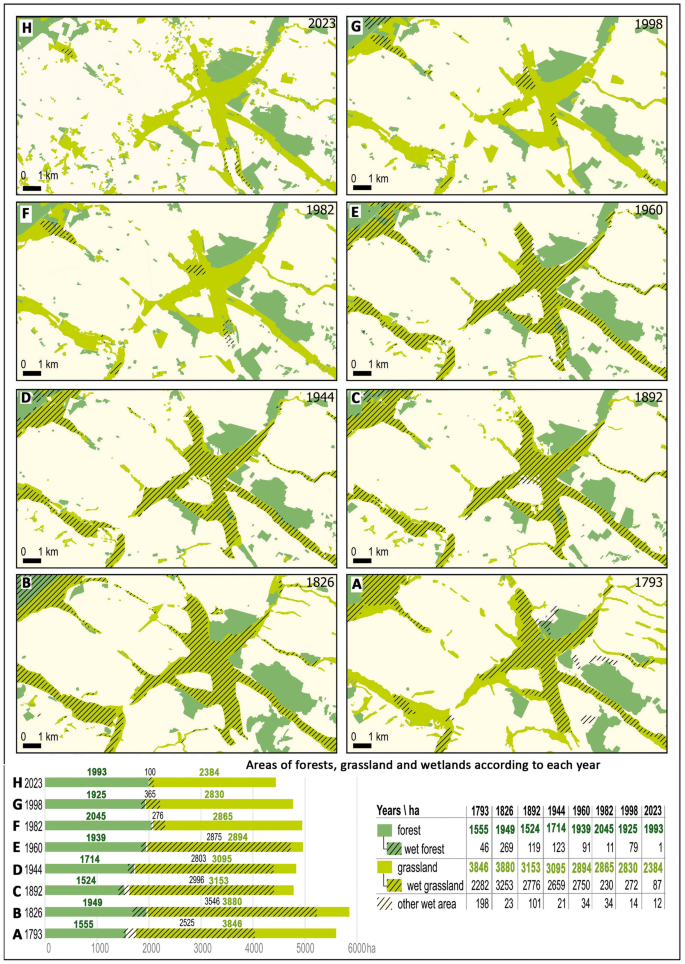
Areas of forests, grasslands and wetlands based on vectorization of topographic maps from 1793 to 2023. Below the maps, a bar chart illustrated the comparative distribution of different land cover types, including dry and wet grasslands, dry and wet forests, and other wetland areas.
Long-term changes in forest cover
In 1793, the area of forests on Kościan Plain was 1555 ha. This forested area expanded to 1949 ha by 1826, but it decreased once more to 1524 ha in 1892. Over the subsequent years, the forested land gradually increased, reaching 2045 hectares by 1982. This peak acreage was slightly reduced, and as of 2023, the forested area stands at 1993 hectares. Throughout the period of 230 years, larger forested segments maintained or even expanded their acreage, with only a few smaller forested spots disappearing. Conversely, new areas were afforested.
Currently, in the structure of the Kościan Plain, there are nearly no forests growing on wetlands. However, in the past, such land cover were present to a slightly greater extent, especially in the year 1826 when the area of forests growing on wetlands reached as high as 14%.
Long-term changes in grassland cover
In 1793, the area covered by grasslands was estimated as 3846 ha. The majority of this land was situated in wetland areas, (2282 ha), while non-wetland habitats overgrown by grasslands accounted for 1564 ha. The grassland area underwent substantial growth by 1826, expanding to 3880 ha. This expansion was primarily attributed to the significant increase in wetland grasslands, which encompassed 3253 ha, whereas grasslands in drier habitats decreased dropping to 627 ha.
Subsequently, by 1892, the grassland area was decreasing, both in wet and drier habitats and it was 2776 ha and 377 ha respectively. Over the next 50 years, land management remained relatively stable, with a slight increase in grasslands within drier habitats and a decline in wetlands. In 1960, a decrease in grassland coverage was observed, with wetland grasslands expanding to 2750 ha, while only 144 ha of grassland persisted outside of wetlands. Overall grassland coverage diminished by 201 ha, reaching 2894 ha.
By 1982, the total grassland area remained nearly constant (decreasing by only 29 ha). However, a significant decline in wetland grasslands (only 230 ha) was noted, with drier habitats taking their place (2626 ha). The situation exhibited stability through 1998, but by 2023, the overall grassland area had diminished by 2487 ha. This reduction included merely 87 ha of wetland grasslands, accompanied by a decreasing extent of other grasslands.
Over the past 230 years, grasslands have undergone profound changes in their surface area and habitat types, but the main grassland complexes have maintained their location along the watercourses. Nevertheless, a strongly advanced unfavorable process of fragmentation of grassland areas is visible. In recent years, new grasslands have been established in many drier areas, but these are mostly very small enclaves.
The second of the developed cartographic visualizations serves as a synthesis of the results of landscape changes throughout the entire time analysis (Fig. 5 ).
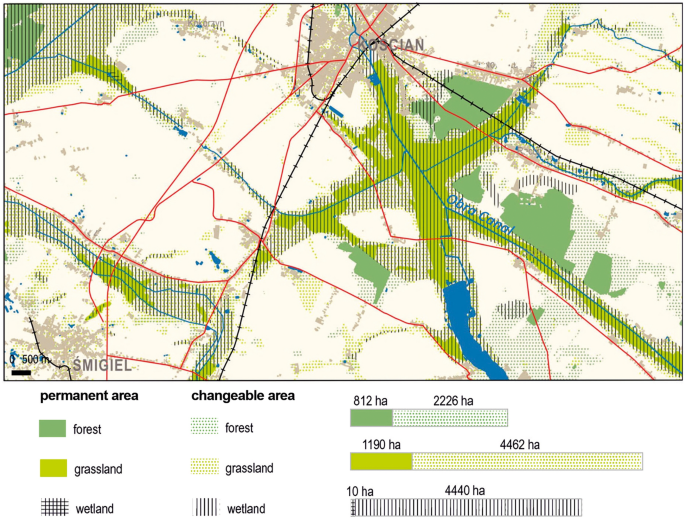
The map displays permanent areas and changes in forests, grasslands, and wetlands on the Kościan Plain between 1793 and 2023. It includes topographical features such as settlements, roads, railways, rivers, canals, and lakes.
Long-term changes in wetland cover
In 1793, a significant portion of the landscape, covering 2525 hectares, was comprised of wetlands, with the majority of it being utilized as grasslands (90%), while the forested wetland area was marginal (2%). By 1826, the wetland area had notably expanded to 3546 hectares, maintaining its predominant use as grasslands (92%). Over the following century, the wetland area gradually decreased, and by 1960, it had dropped to 2875 hectares, with grasslands still dominating (96%).
In 1982, a drastic reduction in the wetland area was detected, leaving only 276 hectares remaining, consisting mainly of wetland grasslands (87%) and some wetland forest (12%). The wetland area experienced a slight increase, reaching 365 hectares in 1998. However, by 2023, only 100 hectares of wetlands remained.
The Kościan Plain remained relatively untouched by industry, with sustainable agricultural practices and limited urbanization due to a small human population in 1793. However, local timber harvesting for construction, fuel, and other needs may have been prevalent 55 .
The most significant changes were observed in the wetland area. Over 200 years, a progressive drying of habitats occurred. This slow process over 150 years was primarily driven by water regulation and land reclamation efforts initiated in the nineteenth century 37 , 65 . In 1982, a dramatic reduction in wetland area occurred, with only 276 hectares remaining, primarily consisting of wetland grasslands (87%) and some wetland forest (12%). The wetland area experienced a slight increase, reaching 365 hectares in 1998. However, by 2023, only 100 wetlands remained. This was primarily the result of large-scale land reclamation efforts initiated in the late 1960s and carried out during the 1970s to the early 1980s 62 , 64 .
On the one hand spatial arrangement of the landscape within the designated preservation areas in the Greater Poland Province reveals that these protected regions exhibit a notably rich and diverse array of natural elements, with a high density of different natural units 80 . On the other hand there was an increase in the share of agricultural areas by 73–81% and an increase in forest areas by 10–16.4% in the communes of Kościan and Śmigiel in the period between 1989 and 2005 39 . Therefore, both of these growths, which are also visible from the study results (with a 7.9% increase in forest cover), could have been influenced by the decrease in grasslands by 6.2%.
The transition towards afforestation, observed between 1944 and 1960, exemplifies a broader trend in land management practices across Europe 81 . Over this temporal span, a discernible trend emerges, indicating a transition towards afforestation of previously cleared lands 23 . This pattern reflects urbanization processes that may result in the abandonment of land, facilitating forest regrowth, a phenomenon observed in the Greater Poland Province 82 or in the Carpathians 83 . The efforts to expand forest cover during this timeframe demonstrate a concerted endeavor to restore ecosystem services, enhance biodiversity, and mitigate the environmental impacts of human activities.
Grasslands have seen significant surface area changes and shifts in habitat types over the past 230 years. While the main grassland complexes have generally remained along watercourses, there is evidence of a notable unfavorable trend of fragmentation in grassland areas. Recent years have witnessed the establishment of new grasslands in drier areas, albeit in smaller enclaves. The decline and fragmentation of grasslands can be attributed, in part, to the intensification of agricultural production in Europe, as noted by Cousins 33 and Kiviniemi and Eriksson 34 . This trend is not unique to the Kościan Plain but is a global phenomenon affecting various landscapes, including mountains, boreal regions, lowlands, and islands, as documented by Monteiro et al. 27 and Aune et al. 84 .
The suggested method for studying the area using the topographic map sheet aimed to be universal, applicable to all handwritten topographic maps from the eighteenth century for the North European Plain 49 . The Map of Lower Saxony (Kurhannoversche Landesaufnahme 1764–1786) serves as an example of a handwritten topographic map for which the proposed method can be applied here due to the circumstances of the map's creation. The constant increase in the number of citizens in the Hanoverian Electorate had led to the necessity of expanding cultivable areas, including the drainage of wetlands, starting from the late seventeenth century. This need to cultivate moors and drain other extensive wastelands in Lower Saxony prompted efforts to create detailed maps 51 .
Conclusions
Overall, the landscape changes on the Kościan Plain reflect a complex interplay between historical events, human activities, and natural processes. In conclusion, the existence of stable permanent areas in both grasslands and forests has contributed to the overall preservation of these landscapes on the Kościan Plain. The conservation of these permanent areas, along with the preservation of drainage canals, has played a vital role in maintaining a significant portion of permanent grasslands and forests.
This study successfully achieved its goal of quantifying changes in forest, grassland, and wetland areas and their distribution in the Kościan Plain, Poland, on the European Plain over the past 230 years. To achieve this objective, we utilized topographic maps as a reliable historical data source. Specifically, the study assessed changes in forest, grassland, and wetland areas, examining their durability over the study period. Wetlands have undergone significant reductions over time, with a drastic decrease in 1982. Land reclamation efforts and environmental factors have driven wetland loss. By 2023, only a fraction of wetlands remained.
Over the past two centuries, the grassland areas in the Kościan Plain fluctuated, influenced by wetland and drier habitat dynamics. Wetland grasslands expanded by 1826 but later declined. By 2023, overall grassland area had diminished remaining modified habitats still situated along watercourses exhibiting a concerning trend towards increased fragmentation. On the other hand the forested area has experienced limited fluctuations, also having a growth tendency peaking at in 1982. Forests on wetlands, which were always marginal, have nearly disappeared.
In this study, landscape metrics, as utilized in literature 53 , 85 , 86 , 87 , were not employed. Our methodology differs in its emphasis, focusing not on the indicative determination of the shape, fragmentation, or dispersion of land cover, but rather on identifying areas where land cover types have remained unchanged over the years (referred to as "permanent"). This approach also considers changeable areas of occurrence and incorporates graphical solutions for effective map representation.
In addition to the quantification of landscape changes, the study proposed a universal method for cartographic visualization. This method aims to support the effective visualization of changes in forest, grassland, and wetland areas in the North European Plain, providing valuable tools for future research and land-use planning efforts. The comprehensive analysis presented in this study provides valuable insights into the historical dynamics of the Kościan Plain's landscape and contributes to our understanding of the long-term impacts of human activities and urbanization on natural ecosystems. The proposed cartographic visualization method offers a practical tool for researchers and policymakers to monitor and manage landscape changes effectively.
Data availability
The datasets used and/or analyzed during the current study available from the corresponding author on reasonable request.
Chen, C. et al. Analysis of regional economic development based on land use and land cover change information derived from Landsat imagery. Sci. Rep. 10 (1), 12721. https://doi.org/10.1038/s41598-020-69716-2 (2020).
Article ADS MathSciNet CAS PubMed PubMed Central Google Scholar
Qu, Y. & Long, H. The economic and environmental effects of land use transitions under rapid urbanization and the implications for land use management. Habitat Int. 82 (12), 113–121. https://doi.org/10.1016/j.habitatint.2018.10.009 (2018).
Article MathSciNet Google Scholar
Atik, M., Altan, T. & Artar, M. Land use changes in relation to coastal tourism developments in Turkish Mediterranean. Pol. J. Environ. Stud. 19 (1), 21–33 (2010).
Google Scholar
Morrison, A. M. Editorial land issues and their impact on tourism development. Land 11 (5), 658. https://doi.org/10.3390/land11050658 (2022).
Article Google Scholar
Aziz, A. & Anwar, M. Landscape change and human environment. Environ. Earth Ecol. 3 (1), 7–12. https://doi.org/10.24051/eee/110396 (2019).
Ellis, E. C. Land use and ecological change. A 12000-year history. Annu. Rev. Environ. Resour. 46 (1), 1–33. https://doi.org/10.1146/annurev-environ-012220-010822 (2021).
Affek, A. N., Zachwatowicz, M. & Solon, J. Long-term landscape dynamics in the depopulated Carpathian foothills: A Wiar River basin case study. Geogr. Pol. 93 (1), 5–23. https://doi.org/10.7163/GPol.0160 (2020).
Olah, B. Historical maps and their application in landscape ecological research. Ekol. Bratisl. 28 (2), 143–151. https://doi.org/10.4149/ekol_2009_02_143 (2009).
Sims, N. C. et al. Developing good practice guidance for estimating land degradation in the context of the United Nations sustainable development goals. Environ. Sci. Policy 92 , 349–355. https://doi.org/10.1016/j.envsci.2018.10.014 (2019).
Sims, N.C., Newnham, G.J., England, J.R., Guerschman, J., Cox, S.J.D., Roxburgh, S.H., Viscarra Rossel, R.A., Fritz. Good Practice Guidance. SDG Indicator 15.3.1. Proportion of Land That Is Degraded Over Total Land Area. Version 2.0. (United Nations Convention to Combat Desertification, Bonn, Germany, 2021).
Bucała-Hrabia, A. Land-use changes and their impact on land degradation in the context of sustainable development of the Polish Western Carpathians during the transition to free-market economics (1986–2019). Geogr. Pol. 96 (1), 131–143. https://doi.org/10.7163/GPol.0249 (2023).
Bucała-Hrabia, A. From communism to a free-market economy. A reflection of socio-economic changes in land use structure in the vicinity of the city (Beskid Sądecki, Western Polish Carpathians). Geogr. Pol. 90 (1), 65–79. https://doi.org/10.7163/GPol.0079 (2017).
Janík, T., Skokanová, H., Borovec, R. & Romportl, D. Landscape changes of rural protected landscape areas in Czechia. From Arable land to permanent Grassland—From Old to New unification?. J. Landsc. Ecol. 14 (3), 88–109. https://doi.org/10.2478/jlecol-2021-0018 (2021).
Skokanová, H. & Eremiášová, R. Changes in the secondary landscape structure and the connection to ecological stability. The cases of two model areas in the Czech Republic. Ekol. Bratisl. 31 (1), 33–45. https://doi.org/10.4149/ekol_2012_01_33 (2012).
Barbier, E. B., Burgess, J. C. & Grainger, A. The forest transition. Towards a more comprehensive theoretical framework. Land Use Policy 27 (2), 98–107. https://doi.org/10.1016/j.landusepol.2009.02.001 (2010).
Mather, A. S. The forest transition. Area 24 (4), 367–379 (1992).
Macias, A. & Szymczak, M. Changes in the forest cover in the town and commune of Krotoszyn in the years 1793–2005. Sylwan 156 (9), 710–720 (2012).
Williams, M. Dark ages and dark areas Global deforestation in the deep past. J. Hist. Geogr. 26 (1), 28–46. https://doi.org/10.1006/jhge.1999.0189 (2000).
Ceccherini, G. et al. Abrupt increase in harvested forest area over Europe after 2015. Nature 583 (7814), 72–77. https://doi.org/10.1038/s41586-020-2438-y (2020).
Article ADS CAS PubMed Google Scholar
Wernick, I. K. et al. Quantifying forest change in the European Union. Nature 592 (7856), E13–E14. https://doi.org/10.1038/s41586-021-03293-w (2021).
Article CAS PubMed Google Scholar
Kubacka, M., Żywica, P., Vila Subirós, J., Bródka, S. & Macias, A. How do the surrounding areas of national parks work in the context of landscape fragmentation? A case study of 159 protected areas selected in 11 EU countries. Land Use Policy 113 (2), 105910. https://doi.org/10.1016/j.landusepol.2021.105910 (2022).
Gordeeva, E., Weber, N. & Wolfslehner, B. The new EU forest strategy for 2030—An analysis of major interests. Forests 13 (9), 1503. https://doi.org/10.3390/f13091503 (2022).
Meyfroidt, P. & Lambin, E. F. Global forest transition. Prospects for an end to deforestation. Annu. Rev. Environ. Resour. 36 (1), 343–371. https://doi.org/10.1146/annurev-environ-090710-143732 (2011).
Latocha, A. Changes in the rural landscape of the Polish Sudety Mountains in the post-war period. Geogr. Pol. 85 (4), 13–21. https://doi.org/10.7163/GPol.2012.4.21 (2012).
Kaim, D. et al. Broad scale forest cover reconstruction from historical topographic maps. Appl. Geogr. 67 (1), 39–48. https://doi.org/10.1016/j.apgeog.2015.12.003 (2016).
Kozak, J. et al. Forest-cover increase does not trigger forest-fragmentation decrease. Case study from the Polish Carpathians. Sustainability 10 (5), 1472. https://doi.org/10.3390/su10051472 (2018).
Monteiro, A. T., Fava, F., Hiltbrunner, E., Della Marianna, G. & Bocchi, S. Assessment of land cover changes and spatial drivers behind loss of permanent meadows in the lowlands of Italian Alps. Landsc. Urban Plan. 100 (3), 287–294. https://doi.org/10.1016/j.landurbplan.2010.12.015 (2011).
Szymura, T. H., Murak, S., Szymura, M. & Raduła, M. W. Changes in forest cover in Sudety Mountains during the last 250 years. Patterns, drivers, and landscape-scale implications for nature conservation. Acta Soc. Bot. Pol. 87 (1), 3576. https://doi.org/10.5586/asbp.3576 (2018).
Baude, M. & Meyer, B. C. Changes in landscape structure and ecosystem services since 1850 analyzed using landscape metrics in two German municipalities. Ecol. Indic. 152 (11), 110365. https://doi.org/10.1016/j.ecolind.2023.110365 (2023).
Müller, S. et al. Land-use intensity and landscape structure drive the acoustic composition of grasslands. Agric. Ecosyst. Environ. 328 (80), 107845. https://doi.org/10.1016/j.agee.2021.107845 (2022).
Palang, H., Mander, Ü. & Luud, A. Landscape diversity changes in Estonia. Landsc. Urban Plan. 41 (3–4), 163–169. https://doi.org/10.1016/S0169-2046(98)00055-3 (1998).
Zachwatowicz, M. & Giętkowski, T. Temporal changes of land cover in relation to chosen environmental variables in different types of landscape. Misc. Geogr. 14 (1), 33–45. https://doi.org/10.2478/mgrsd-2010-0004 (2010).
Cousins, S. A. O. Analysis of land-cover transitions based on 17th and 18th century cadastral maps and aerial photographs. Landsc. Ecol. 16 (1), 41–54. https://doi.org/10.1023/A:1008108704358 (2001).
Kiviniemi, K. & Eriksson, O. Size-related deterioration of semi-natural grassland fragments in Sweden. Diversi. Distrib. 8 (1), 21–29. https://doi.org/10.1046/j.1366-9516.2001.00125.x (2002).
Walpole, M. & Davidson, N. Stop draining the swamp. It’s time to tackle wetland loss. Oryx 52 (4), 595–596. https://doi.org/10.1017/S0030605318001059 (2018).
Szoszkiewicz, J., Szoszkiewicz, K. ed. The estimation of the agricultural value of grassland communities on the base of large area geobotanical surveys. Mat. II Międzynarodowego Symposjum IAVS (Bailleul, 1994).
Ballut-Dajud, G. A. et al. Factors affecting wetland loss. Rev. Land 11 (3), 434. https://doi.org/10.3390/land11030434 (2022).
Ławniczak, R. & Kubiak, J. Cartographic sources as a base of knowledge about land use in selected areas in the north-western Poland. Polish Cartogr. Rev. 54 (1), 143–157. https://doi.org/10.2478/pcr-2022-0010 (2022).
Łowicki, D. Land use changes in Poland during transformation. Landsc. Urban Plan. 87 (4), 279–288. https://doi.org/10.1016/j.landurbplan.2008.06.010 (2008).
Müller, D. et al. Regime shifts limit the predictability of land-system change. Global Environ. Change 28 (1), 75–83. https://doi.org/10.1016/j.gloenvcha.2014.06.003 (2014).
Ramankutty, N. & Coomes, O. T. Land-use regime shifts. An analytical framework and agenda for future land-use research. Ecol. Soc. https://doi.org/10.5751/ES-08370-210201 (2016).
Medyńska-Gulij, B. Map compiling, map reading, and cartographic design in “Pragmatic pyramid of thematic mapping”. Quaest. Geogr. 29 (1), 57–63. https://doi.org/10.2478/v10117-010-0006-5 (2010).
Szantoi, Z. et al. Addressing the need for improved land cover map products for policy support. Environ. Sci. Policy 112 , 28–35. https://doi.org/10.1016/j.envsci.2020.04.005 (2020).
Article PubMed PubMed Central Google Scholar
Forrest, D. Thematic Maps in Geography, 260–267 (Elsevier, 2015).
Pieniążek, M. & Zych, M. Statistical maps. Data visualisation methods (Statistics Poland, Warszawa, 2020).
Smaczyński, M., Medyńska-Gulij, B. & Halik, Ł. The land use mapping techniques (including the areas used by pedestrians) based on low-level aerial imagery. ISPRS Int. Geo-Inf. 9 (12), 754. https://doi.org/10.3390/ijgi9120754 (2020).
Strode, G. et al. Geovisualization of land use and land cover using bivariate maps and Sankey flow diagrams. Proc. Int. Cartogr. Assoc. 1 , 1–5. https://doi.org/10.5194/ica-proc-1-106-2018 (2018).
de Coene, K. et al. Ferraris, the legend. Cartogr. J. 49 (1), 30–42. https://doi.org/10.1179/1743277411Y.0000000013 (2012).
Medyńska-Gulij, B. & Żuchowski, T. J. An analysis of drawing techniques used on european topographic maps in the eighteenth century. Cartogr. J. 55 (4), 309–325. https://doi.org/10.1080/00087041.2018.1558021 (2018).
Scharfe, W. Abriss der Kartographie Brandenburgs 1771–1821 (De Gruyter, 1972).
Book Google Scholar
Bauer, H. Die Kurhannoversche Landesaufnahme des 18. Jahrhunderts. Erläuterungen zu den farbigen Reproduktionen im Maßstab 1 : 25 000 mit Zeichenerklärung und Blattübersicht (Niedersächsisches Landesverwaltungsamt–Landesvermessung, Hanover, 1993).
Szajdak, L., Życzyńska-Bałoniak, I. & Jaskulska, R. Impact of afforestation on the limitation of the spread of the pollutions in ground water and in soils. Pol. J. Environ. Stud. 12 (4), 453–460 (2003).
CAS Google Scholar
Affek, A. N. Dynamika krajobrazu. Uwarunkowania i prawidłowości na przykładzie dorzecza Wiaru w Karpatach (XVIII-XXI wiek) = Landscape dynamics : determinants and patterns on the example of the Wiar river basin in the Carpathians (18th - 21st century) 251 (IGiPZ PAN, Warszawa, 2016).
Schils, R. L. M. et al. Permanent grasslands in Europe. Land use change and intensification decrease their multifunctionality. Agric. Ecosyst. Environ. 330 (3), 107891. https://doi.org/10.1016/j.agee.2022.107891 (2022).
Kędziora, A. et al. in Biodiversity enrichment in a diverse world , (ed by G. A. Lameed) (InTech, Rijeka, Croatia, 2012), pp. 281–336.
Kulik, M. et al. Grazing of native livestock breeds as a method of grassland protection in Roztocze National Park, Eastern Poland. J. Ecol. Eng. 21 (3), 61–69. https://doi.org/10.12911/22998993/118298 (2020).
Gabryszuk, M., Barszczewski, J. & Wróbel, B. Characteristics of grasslands and their use in Poland. J. Water Land. Dev. 51 (9–13), 243–249. https://doi.org/10.24425/jwld.2021.139035 (2021).
Hayes, E., Higgins, S., Geris, J. & Mullan, D. Grassland reseeding. Impact on soil surface nutrient accumulation and using LiDAR-based image differencing to infer implications for water quality. Agriculture 12 (11), 1854. https://doi.org/10.3390/agriculture12111854 (2022).
Article CAS Google Scholar
Bai, Y. & Cotrufo, M. F. Grassland soil carbon sequestration. Current understanding, challenges, and solutions. Science 377 (6606), 603–608. https://doi.org/10.1126/science.abo2380 (2022).
Szymura, T. H. & Szymura, M. Spatial structure of grassland patches in Poland. Implications for nature conservation. Acta Soc. Bot. Pol. 88 (1), 3615. https://doi.org/10.5586/asbp.3615 (2019).
Kryszak, A., Strychalska, A., Klarzyńska, A., Łubniewska, A. & Kryszak, J. Natural values of maritime salt grasslands and the possibilities of their preservation. J. Res. App. Agr. Eng. 60 (4), 13–16 (2015).
Kozaczyk, P. Transformations of land amelioration systems in the catchment of the Rów Wyskoc River in the context of their use to counteract the effects of drought. J. Ecol. Eng. 18 (6), 103–109. https://doi.org/10.12911/22998993/76834 (2017).
Czerwiński, S. et al. Environmental implications of past socioeconomic events in Greater Poland during the last 1200 years. Synthesis of paleoecological and historical data. Quat. Sci. Rev. 259 (2), 106902. https://doi.org/10.1016/j.quascirev.2021.106902 (2021).
Pijanowski, B. C. et al. Soundscape ecology. The science of sound in the landscape. BioScience 61 (3), 203–216. https://doi.org/10.1525/bio.2011.61.3.6 (2011).
Mizgajski, A. Development of rural landscape in Wielkopolska in reference to metabolism of agroecosystems. Quaest. Geogr. 26A , 39–51 (2007).
Hazeu, G. W., Mucher, C. A., Kramer, H. & Kienast, F. Compilation and assessment of pan-European land cover changes. Proceedings 28th EARSeL Symposium: Remote Sensing for a Changing Europe, Istanbul, Turkey, 2-7 June, 2008, 220–238 (IOS Press, Istanbul, Turkey, 2008).
Wulf, M. & Gross, J. Die Schmettau-Schulenburgsche Karte. Eine Legende für das Land Brandenburg (Ostdeutschland) mit kritischen Anmerkungen (Sauerländer, 2004).
Antonson, H. Revisiting the “Reading Landscape Backwards” approach. Advantages disadvantages, and use of the retrogressive method. Rural Landsc. Soc. Environ. Hist. 5 (1), 1–15 (2018).
Baker, A. A note on the retrogressive and retrospective approaches in historical geography. Erdkunde 22 (3), 243–244. https://doi.org/10.3112/ERDKUNDE.1968.03.07 (1968).
Gulley, J. L. M. The Retrospective approach in historical geography (Die rückschreitende Methode in der historischen Geographie). Erdkunde 15 (4), 306–309. https://doi.org/10.2478/quageo-2020-0019 (1961).
Medyńska-Gulij, B. et al. Die kartographische Rekonstruktion der Landschaftsentwicklung des Oberschlesischen Industriegebiets (Polen) und des Ruhrgebiets (Deutschland). KN J. Cartogr. Geogr. Inf. 69 (2), 131–142. https://doi.org/10.1007/s42489-019-00018-y (2019).
Gregory, I. & Ell, P. S. Historical GIS. Technologies, methodologies, and scholarship (Cambridge University Press, Cambridge, 2007).
Cybulski, P., Wielebski, Ł, Medyńska-Gulij, B., Lorek, D. & Horbiński, T. Spatial visualization of quantitative landscape changes in an industrial region between 1827 and 1883. Case study Katowice, southern Poland. J. Maps 16 (1), 77–85. https://doi.org/10.1080/17445647.2020.1746416 (2020).
Związek, T., Panecki, T. & Zachara, T. A retrogressive approach to reconstructing the sixteenth-century forest landscapes of western Poland. J. Hist. Geogr. 74 (4), 55–74. https://doi.org/10.1016/j.jhg.2021.01.008 (2021).
Affek, A. N. Georeferencing of historical maps using GIS, as exemplified by the Austrian Military Surveys of Galicia. Geogr. Pol. 86 (4), 375–390. https://doi.org/10.7163/GPol.0160 (2013).
Panecki, T. The evaluation of archival maps in geohistorical research. Misc. Geogr. 19 (4), 72–77. https://doi.org/10.1515/mgrsd-2015-0027 (2015).
Luft, J. & Schiewe, J. Automatic content-based georeferencing of historical topographic maps. Trans. GIS 25 (6), 2888–2906. https://doi.org/10.1111/tgis.12794 (2021).
Müller-Miny, H. Die Kartenaufnahme der Rheinlande durch Tranchot und v. Müffling, 1801–1828. Teil 2 - Das Gelände - Eine quellenkritische Untersuchung des Kartenwerkes (Köln, 1975).
Lorek, D. & Medyńska-Gulij, B. Scope of information in the legends of topographical maps in the nineteenth century-Urmesstischblätter. Cartogr. J. 57 (2), 113–129. https://doi.org/10.1080/00087041.2018.1547471 (2020).
Bródka, S., Kubacka, M. & Macias, A. Landscape diversity and the directions of its protection in Poland illustrated with an example of Wielkopolskie Voivodeship. Sustainability 13 (24), 13812. https://doi.org/10.3390/su132413812 (2021).
Jepsen, M. R. et al. Transitions in European land-management regimes between 1800 and 2010. Land Use Policy 49 (1), 53–64. https://doi.org/10.1016/j.landusepol.2015.07.003 (2015).
Macias, A. & Dryjer, M. Forest Cover dynamics in the city of Poznań from 1830 to 2004. Quaest. Geogr. 29 (3), 47–57. https://doi.org/10.2478/v10117-010-0022-5 (2010).
Affek, A. N., Wolski, J., Zachwatowicz, M., Ostafin, K. & Radeloff, V. C. Effects of post-WWII forced displacements on long-term landscape dynamics in the Polish Carpathians. Landsc. Urban Plan. 214 (3), 104164. https://doi.org/10.1016/j.landurbplan.2021.104164 (2021).
Aune, S., Bryn, A. & Hovstad, K. A. Loss of semi-natural grassland in a boreal landscape. Impacts of agricultural intensification and abandonment. J. Land Use Sci. 13 (4), 375–390. https://doi.org/10.1080/1747423X.2018.1539779 (2018).
Cain, D. H., Riitters, K. & Orvis, K. A multi-scale analysis of landscape statistics. Landsc. Ecol. 12 (4), 199–212. https://doi.org/10.1023/A:1007938619068 (1997).
Huang, J., Lu, X. X. & Sellers, J. M. A global comparative analysis of urban form. Applying spatial metrics and remote sensing. Landsc. Urban Plan. 82 (4), 184–197. https://doi.org/10.1016/j.landurbplan.2007.02.010 (2007).
Wang, Z., Liu, Y., Li, Y. & Su, Y. Response of ecosystem health to land use changes and landscape patterns in the Karst Mountainous regions of Southwest China. Int. J. Environ. Res. Public Health 19 (6), 3273. https://doi.org/10.3390/ijerph19063273 (2022).
Article CAS PubMed PubMed Central Google Scholar
Download references
Author information
Authors and affiliations.
Department of Cartography and Geomatics, Adam Mickiewicz University, Poznań, Krygowskiego 10, 61-680, Poznan, Poland
Beata Medyńska-Gulij, Paweł Cybulski & Łukasz Wielebski
Department of Ecology and Environmental Protection, Poznań University of Life Sciences, Poznan, Poland
Krzysztof Szoszkiewicz
You can also search for this author in PubMed Google Scholar
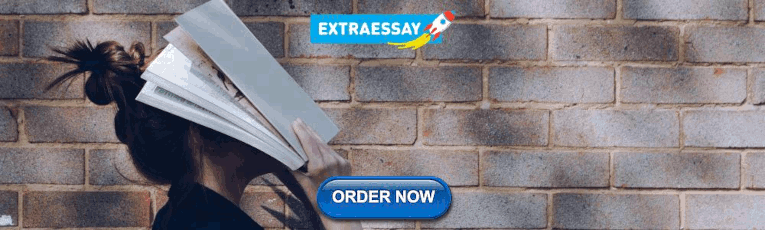
Contributions
Methodology, formal analysis, and investigation, original draft preparation, review, and editing: B.M.-G., K.S., P.C. & Ł.W.; B.M.-G., K.S., P.C. & Ł.W.; figure preparation: B.M.-G. & Ł.W.; conceptualization: B.M.-G..
Corresponding author
Correspondence to Paweł Cybulski .
Ethics declarations
Competing interests.
The authors declare no competing interests.
Additional information
Publisher's note.
Springer Nature remains neutral with regard to jurisdictional claims in published maps and institutional affiliations.
Rights and permissions
Open Access This article is licensed under a Creative Commons Attribution 4.0 International License, which permits use, sharing, adaptation, distribution and reproduction in any medium or format, as long as you give appropriate credit to the original author(s) and the source, provide a link to the Creative Commons licence, and indicate if changes were made. The images or other third party material in this article are included in the article's Creative Commons licence, unless indicated otherwise in a credit line to the material. If material is not included in the article's Creative Commons licence and your intended use is not permitted by statutory regulation or exceeds the permitted use, you will need to obtain permission directly from the copyright holder. To view a copy of this licence, visit http://creativecommons.org/licenses/by/4.0/ .
Reprints and permissions
About this article
Cite this article.
Medyńska-Gulij, B., Szoszkiewicz, K., Cybulski, P. et al. Permanent areas and changes in forests, grasslands, and wetlands in the North European Plain since the eighteenth century—a case study of the Kościan Plain in Poland. Sci Rep 14 , 10305 (2024). https://doi.org/10.1038/s41598-024-61086-3
Download citation
Received : 04 December 2023
Accepted : 30 April 2024
Published : 05 May 2024
DOI : https://doi.org/10.1038/s41598-024-61086-3
Share this article
Anyone you share the following link with will be able to read this content:
Sorry, a shareable link is not currently available for this article.
Provided by the Springer Nature SharedIt content-sharing initiative
- Land cover changes
- Topographic map
- North European Plain
By submitting a comment you agree to abide by our Terms and Community Guidelines . If you find something abusive or that does not comply with our terms or guidelines please flag it as inappropriate.
Quick links
- Explore articles by subject
- Guide to authors
- Editorial policies
Sign up for the Nature Briefing newsletter — what matters in science, free to your inbox daily.

Allocation, morphology, physiology: multiple aspects of above- and below-ground responses to water table stress, duration of drainage in alpine wetland plants ( Carex muliensis )
- Research Article
- Published: 07 May 2024
Cite this article
- Xiangtao Wang 1 ,
- Jun Yang 2 , 3 ,
- Yang Gao 1 , 4 ,
- Junqin Li 1 ,
- Yuting Yang 1 &
- Puchang Wang 1
38 Accesses
Explore all metrics
Plants respond to resource stress by modifying various aspects of morphology, physiology, and allocation. Yet, a comprehensive understanding of the mechanisms governing the functional coordination between phenotypic plasticity (the ability to regulate traits) and phenotypic integration (degree of association and coordination between traits) in alleviating resource stress remains elusive.
In alpine wetland, we investigated the interactions among root and leaf functional traits, phenotype plasticity, and integration mechanisms within a dominant species, Carex muliensis , under three water level treatments (control, deep, shallow) and two drainage durations (long-term, short-term).
Declining water table prompted a shift in leaf towards resource-conserving strategies, typified by reductions in specific leaf area and photosynthetic efficiency. Conversely, root shifted towards resource-acquisition strategies to improve competitiveness under low water tables. Notably, a discernible trade-off, manifests between leaf and root phenotypic plasticity and integration across instances of long-term drainage, while short-term drainage scenarios exhibit a synergistic relationship, between these attributes. This observation suggests that long-term drainage fosters a diminution in the integration of leaf and root traits despite heightened plasticity, contrasting with the more cohesive response observed in the context of short-term drainage.
Conclusions
We conclude that, declining water table altered the resource use strategies of plant leaf and root, and trade-offs/synergistic relationships between leaf and root phenotypic plasticity and integration contributed to plant adaptation to drainage durations. This study contributes to the understanding of the role of leaf and root overall phenotypic plasticity and integration in plant adaptation in the context of declining water tables of alpine wetlands, and will help predict the future dynamics of alpine wetland ecosystems.
This is a preview of subscription content, log in via an institution to check access.
Access this article
Price includes VAT (Russian Federation)
Instant access to the full article PDF.
Rent this article via DeepDyve
Institutional subscriptions
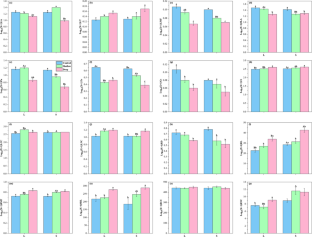
Similar content being viewed by others
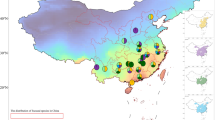
Environment-driven intraspecific variation shows coordination of functional traits of deciduous oaks among and within different biological levels
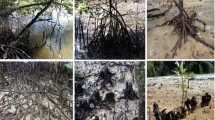
Mangrove root: adaptations and ecological importance
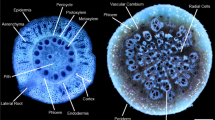
Root anatomy and soil resource capture
Data availability.
Data will be made available on request.
Abbreviations
Leaf thickness
Leaf mass fraction
Specific leaf area
Saturated net photosynthesis rate
Transpiration rate
Stomatal conductance
Intercellular CO 2 concentration
Leaf carbon content
Leaf nitrogen content
Root diameter
Root length
Root mass fraction
Specific root length
Root carbon concentration
Root nitrogen concentration
Barker DH, Vanier C, Naumburg E (2006) Enhanced monsoon precipitation and nitrogen deposition affect leaf traits and photosynthesis differently in spring and summer in the desert shrub Larrea tridentata. New Phytol 1694:799–808. https://doi.org/10.1111/j.1469-8137.2006.01628.x
Article Google Scholar
Bennett AE, Groten K (2022) The costs and benefits of plant-arbuscular mycorrhizal fungal interactions. Annu Rev Plant Biol 73:649–672. https://doi.org/10.1146/annurev-arplant-102820-124504
Article CAS PubMed Google Scholar
Bergmann J, Weigelt A, van Der Plas F (2020) The fungal collaboration gradient dominates the root economics space in plants. Science Advances 627:eaba375. https://doi.org/10.1126/sciadv.aba3756
Article CAS Google Scholar
Blom C, Voesenek L (1996) Flooding: the survival strategies of plants. Trends Ecol Evol 117:290–295. https://doi.org/10.1016/0169-5347(96)10034-3
Bloom AJ, Chapin FS, Mooney HA (1985) Resource limitation in plants - an economic analogy. Annu Rev Ecol Syst 16:363–392. https://doi.org/10.1146/annurev.es.16.110185.002051
Campany CE, Martin L, Watkins JJE (2019) Convergence of ecophysiological traits drives floristic composition of early lineage vascular plants in a tropical forest floor. Ann Bot 1235:793–803. https://doi.org/10.1093/aob/mcy210
Cao R, Wei X, Yang YHS, Xi XQ, Wu XW (2017) The effect of water table decline on plant biomass and species composition in the Zoige peatland: A four-year in situ field experiment. Agr Ecosyst Environ 247:389–395. https://doi.org/10.1016/j.agee.2017.07.008
Cetner MD, Kalaji HM, Goltsev V (2017) Effects of nitrogen-deficiency on efficiency of light-harvesting apparatus in radish. Plant Physiol Biochem 119:81–92. https://doi.org/10.1016/j.plaphy.2017.08.016
Chen H, Yang G, Peng C et al (2014) The carbon stock of alpine peatlands on the Qinghai-Tibetan Plateau during the Holocene and their future fate. Quatern Sci Rev 95:151–158. https://doi.org/10.1016/j.quascirev.2014.05.003
Chmara R, Szmeja J, Banas K (2018) The relationships between structural and functional diversity within and among macrophyte communities in lakes. J Limnol 771:100–108. https://doi.org/10.4081/jlimnol.2017.1630
Cui MM, Ma AZ, Qi HY, Zhuang GQ, Zhao GH (2015) Warmer temperature accelerates methane emissions from the Zoige wetland on the Tibetan Plateau without changing methanogenic community composition. Sci Rep 51:11616. https://doi.org/10.1038/srep11616
Damián X, Ochoa-López S, Gaxiola A (2020) Natural selection acting on integrated phenotypes: covariance among functional leaf traits increases plant fitness. New Phytol 2251:546–557. https://doi.org/10.1111/nph.16116
Dong ZB, Hu GY, Yan CZ, Wang WL (2010) Aeolian desertification and its causes in the Zoige Plateau of China’s Qinghai-Tibetan Plateau. Environ Earth Sci 59:1731–1740. https://doi.org/10.1007/s12665-009-0155-9
Dong LQ, Yang W, Yao PJ (2020) Responses of Carex muliensis growth characteristics to water level gradient in Zoige Plateau wetland. Acta Ecol Sin 40:590–598. https://doi.org/10.5846/stxb201807041465
Du EZ, De Vries W, Han WX, Liu XJ, Yan ZB, Jiang Y (2016) Imbalanced phosphorus and nitrogen deposition in China’s forests. Atmos Chem Phys 1613:8571–8579. https://doi.org/10.5194/acp-16-8571-2016
Eissenstat DM, Wells CE, Yanai RD (2000) Building roots in a changing environment: implications for root longevity. New Phytol 1471:33–42. https://doi.org/10.1046/j.1469-8137.2000.00686.x
Fontana V, Kohler M, Niedrist G, Bahn M (2017) Decomposing the land-use specific response of plant functional traits along environmental gradients. Sci Total Environ 599:750–759. https://doi.org/10.1016/j.scitotenv.2017.04.245
Freschet GT, Roumet C (2017) Sampling roots to capture plant and soil functions. Funct Ecol 318:1506–1518. https://doi.org/10.1111/1365-2435.12883
Freschet GT, Violle C, Bourget MY, Scherer-Lorenzen M (2018) Allocation, morphology, physiology, architecture: The multiple facets of plant above-and below-ground responses to resource stress. New Phytol 2194:1338–1352. https://doi.org/10.1111/nph.15225
Godoy O, Valladares F, Castro-Díez P (2012) The relative importance for plant invasiveness of trait means, and their plasticity and integration in a multivariate framework. New Phytol 1954:912–922. https://doi.org/10.1111/j.1469-8137.2012.04205.x
Gratani L (2014) Plant phenotypic plasticity in response to environmental factors. Advances in Botany. https://doi.org/10.1155/2014/208747
Heilmeier H (2019) Functional traits explaining plant responses to past and future climate changes. Flora 254:1–11. https://doi.org/10.1016/j.flora.2019.04.004
Ho MD, Rosas JC, Brown KM (2005) Root architectural tradeoffs for water and phosphorus acquisition. Funct Plant Biol 328:737–748. https://doi.org/10.1071/fp05043
Hodge A (2009) Root decisions. Plant, Cell Environ 326:628–640. https://doi.org/10.1111/j.1365-3040.2008.01891.x
Knight CA, Ackerly DD (2003) Evolution and plasticity of photosynthetic thermal tolerance, specific leaf area and leaf size: congeneric species from desert and coastal environments. New Phytol 1602:337–347. https://doi.org/10.1046/j.1469-8137.2003.00880.x
Kong D, Wang J, Wu H (2019) Nonlinearity of root trait relationships and the root economics spectrum. Nat Commun 101:2203. https://doi.org/10.1038/s41467-019-10245-6
Laiho R (2006) Decomposition in peatlands: Reconciling seemingly contrasting results on the impacts of lowered water levels. Soil Biol Biochem 388:2011–2024. https://doi.org/10.1016/j.soilbio.2006.02.017
Laine AM, Leppälä M, Tarvainen O (2011) Restoration of managed pine fens: effect on hydrology and vegetation. Appl Veg Sci 143:340–349. https://doi.org/10.1111/j.1654-109x.2011.01123.x
Lamanna C, Blonder B, Violle C (2014) Functional trait space and the latitudinal diversity gradient. Proc Natl Acad Sci 11138:13745–13750. https://doi.org/10.1073/pnas.1317722111
Lei L, Zhao C, Li X (2018) The relationship between chlorophyll and leaf area, leaf thickness of Liguaria virgaurea under density-dependent condition in Gahai wetland. Chinese J Ecol 3712:3647. https://doi.org/10.13292/j.1000-4890.201812.031
Leung C, Rescan M, Grulois D (2020) Reduced phenotypic plasticity evolves in less predictable environments. Ecol Lett 2311:1664–1672. https://doi.org/10.1111/ele.13598/v1/review1
Lin GG, Zeng DH, Mao R (2020) Traits and their plasticity determine responses of plant performance and community functional property to nitrogen enrichment in a boreal peatland. Plant Soil 449:151–167. https://doi.org/10.1007/s11104-020-04478-4
Liu H, Liu GH, Xing W (2021) Functional traits of submerged macrophytes in eutrophic shallow lakes affect their ecological functions. Sci Total Environ 760:143332. https://doi.org/10.1016/j.scitotenv.2020.143332
Liu H, Ye Q, Simpson KJ (2022) Can evolutionary history predict plant plastic responses to climate change? New Phytol 2353:1260–1271. https://doi.org/10.1111/nph.18194
Lukács BA, Vojtkó AE, Mesterházy A (2017) Growth-form and spatiality driving the functional difference of native and alien aquatic plants in Europe. Ecol Evol 73:950–963. https://doi.org/10.1002/ece3.2703
Luo FL, Huang L, Lei T (2016) Responsiveness of performance and morphological traits to experimental submergence predicts field distribution pattern of wetland plants. J Veg Sci 272:340–351. https://doi.org/10.1111/jvs.12352
Lynch JP (2015) Root phenes that reduce the metabolic costs of soil exploration: opportunities for 21st century agriculture. Plant, Cell Environ 38:1775–1784. https://doi.org/10.1111/pce.12451
Article PubMed Google Scholar
Ma X, Yan J, Wang F (2019) Trait and density responses of Spartina alterniflora to inundation in the Yellow River Delta, China. Mar Pollut Bull 146:857–864. https://doi.org/10.1016/j.marpolbul.2019.07.022
Matesanz S, Blanco-Sánchez M, Ramos-Muñoz M (2021) Phenotypic integration does not constrain phenotypic plasticity: differential plasticity of traits is associated to their integration across environments. New Phytol 2316:2359–2370. https://doi.org/10.1111/nph.17536
McCormack ML, Dickie IA, Eissensat DM (2015) Redefining fine roots improves understanding ofbelow-ground contributions to terrestrial biosphere processes. New Phytol 207:505–518. https://doi.org/10.1111/nph.13363
Miller CA, Benscoter BW, Turetsky MR (2015) The effect of long-term drying associated with experimental drainage and road construction on vegetation composition and productivity in boreal fens. Wetlands Ecol Manage 23:845–854. https://doi.org/10.1007/s11273-015-9423-5
Niinemets Ü (2010) A review of light interception in plant stands from leaf to canopy in different plant functional types and in species with varying shade tolerance. Ecol Res 25:693–714. https://doi.org/10.1007/s11284-010-0712-4
Poorter L, Rozendaal DMA (2008) Leaf size and leaf display of thirty-eight tropical tree species. Oecologia 158:35–46. https://doi.org/10.1007/s00442-008-1131-x
Potvin LR, Kane ES, Chimner RA (2015) Effects of water table position and plant functional group on plant community, aboveground production, and peat properties in a peatland mesocosm experiment (PEATcosm). Plant Soil 387:277–294. https://doi.org/10.1007/s11104-014-2301-8
Rubol S, Silver WL, Bellin A (2012) Hydrologic control on redox and nitrogen dynamics in a peatland soil. Sci Total Environ 432:37–46. https://doi.org/10.1016/j.scitotenv.2012.05.073
Santiago LS, Kitajima K, Wright SJ (2004) Coordinated changes in photosynthesis, water relations and leaf nutritional traits of canopy trees along a precipitation gradient in lowland tropical forest. Oecologia 139:495–502. https://doi.org/10.1007/s00442-004-1542-2
Schöb C, Armas C, Guler M, Prieto I (2013) Variability in functional traits mediates plant interactions along stress gradients. J Ecol 1013:753–762. https://doi.org/10.1111/1365-2745.12062
Shi XM, Qi JH, Zakari S (2023) Leaf phenotypic plasticity coupled with integration facilitates the adaptation of plants to enhanced N deposition. Environ Pollut 327:121570. https://doi.org/10.1016/j.envpol.2023.121570
Stotz GC, Salgado-Luarte C, Escobedo VM (2022) Phenotypic plasticity and the leaf economics spectrum: plasticity is positively associated with specific leaf area. Oikos 2022(11):e09342. https://doi.org/10.1111/oik.09342
Sun L, Yang GJ, Zhang Y (2022) Leaf functional traits of two species affected by nitrogen addition rate and period not nitrogen compound type in a meadow grassland. Front Plant Sci 13:841464. https://doi.org/10.3389/fpls.2022.841464
Article PubMed PubMed Central Google Scholar
Urbanová Z, Bárta J (2016) Effects of long-term drainage on microbial community composition vary between peatland types. Soil Biol Biochem 92:16–26. https://doi.org/10.1016/j.soilbio.2015.09.017
Wang S, Zhou DW (2021) Morphological canalization, integration, and plasticity in response to population density in Abutilon theophrasti: Influences of soil conditions and growth stages. Ecol Evol 1117:11945–11959. https://doi.org/10.1002/ece3.7960
Weemstra M, Mommer L, Visser EJW (2016) Towards a multidimensional root trait framework: a tree root review. New Phytol 2114:1159–1169. https://doi.org/10.1111/nph.14003
Wei X, Cao R, Wu XW, Eisenhauer N (2018) Effect of water table decline on the abundances of soil mites, springtails, and nematodes in the Zoige peatland of eastern Tibetan Plateau. Appl Soil Ecol 129:77–83. https://doi.org/10.1016/j.apsoil.2018.05.006
Westoby M, Falster DS, Moles AT (2002) Plant ecological strategies: some leading dimensions of variation between species. Ann Rev Ecol Syst 33:125–159. https://doi.org/10.1146/annurev.ecolsys.33.010802.150452
Wright IJ, Reich PB, Westoby M (2004) The worldwide leaf economics spectrum. Nature 428:821–827. https://doi.org/10.1038/nature02403
Wright IJ, Ackerly DD, Bongers F (2007) Relationships among ecologically important dimensions of plant trait variation in seven Neotropical forests. Ann Bot 99:1003–1015. https://doi.org/10.1093/aob/mcl066
Xiang S, Guo RQ, Wu N, Sun SC (2009) Current status and future prospects of Zoige Marsh in eastern Qinghai-Tibet Plateau. Ecol Eng 354:553–562. https://doi.org/10.1016/j.ecoleng.2008.02.016
Xiong J, Shao X, Yuan H (2022) Carbon, nitrogen, and phosphorus stoichiometry and plant growth strategy as related to land-use in hangzhou bay coastal wetland. China Frontiers in Plant Science 13:946949. https://doi.org/10.3389/fpls.2022.946949
Xue D, Chen H, Zhan W, He YX (2021) How do water table drawdown, duration of drainage, and warming influence greenhouse gas emissions from drained peatlands of the Zoige Plateau? Land Degrad Dev 32:3351–3364. https://doi.org/10.1002/ldr.4013
Yang G, Chen H, Wu N, Tian JQ (2014) Effects of soil warming, rainfall reduction and water table level on CH 4 emissions from the Zoige peatland in China. Soil Biol Biochem 78:83–89. https://doi.org/10.1016/j.soilbio.2014.07.013
Yang G, Wang M, Chen H (2017) Responses of CO 2 emission and pore water DOC concentration to soil warming and water table drawdown in Zoige Peatlands. Atmos Environ 152:323–329. https://doi.org/10.1016/j.atmosenv.2016.12.051
Yang J, Gao YH, Zhao C, Chen H (2023) Leaf phenotypic plasticity and integration balance plant adaptation to water table decline: a mesocosm experiment. Plant and Soil. 1–17. https://doi.org/10.1007/s11104-023-06418-4
Yin QL, Wang L, Lei ML, Dang H (2018) The relationships between leaf economics and hydraulic traits of woody plants depend on water availability. Sci Total Environ 621:245–252. https://doi.org/10.1016/j.scitotenv.2017.11.171
Yu CQ, Wang JW, Shen ZX et al (2019) Effects of experimental warming and increased precipitation on soil respiration in an alpine meadow in the Northern Tibetan Plateau[J]. Sci Total Environ 647:1490–1497
Zervas D, Tsiaoussi V, Kallimanis AS, Dimopoulis P (2019) Exploring the relationships between aquatic macrophyte functional traits and anthropogenic pressures in freshwater lakes. Acta Oecologica 99:103443. https://doi.org/10.1016/j.actao.2019.103443
Zhang D, Peng Y, Li F (2019) Trait identity and functional diversity co-drive response of ecosystem productivity to nitrogen enrichment. J Ecol 1075:2402–2414. https://doi.org/10.1111/1365-2745.13184
Zobel RW, Kinraide TB, Baligar VC (2007) Fine root diameters can change in response to changes in nutrient concentrations. Plant Soil 297:243–254. https://doi.org/10.1007/s11104-007-9341-2
Download references
Acknowledgements
We thank comrades Dan Xue and Qing Qiu for their help in sample collection and the anonymous reviewers for their valuable comments and suggestions.This work was funded by the National Natural Science Foundation of China (42161012);Science and Technology Major Project of Tibetan Autonomous Region of China (XZ202201ZD0005G02) and the Projects of Science and Technology of Guizhou Province (QKHPTRC-GCC[2022]022-1).
Author information
Authors and affiliations.
School of Life Sciences, Guizhou Normal University, Guiyang, 550025, China
Xiangtao Wang, Yang Gao, Junqin Li, Yuting Yang & Puchang Wang
Key Laboratory of Reservoir Aquatic Environment, Chongqing Institute of Green and Intelligent Technology, Chinese Academy of Sciences, Chongqing, 400714, China
Chongqing School, University of Chinese Academy of Sciences (UCAS Chongqing), Chongqing, 400714, China
School of Geography and Environmental Sciences/Karst Research Institute, Guizhou Normal University, Guiyang, 550025, China
You can also search for this author in PubMed Google Scholar
Corresponding author
Correspondence to Puchang Wang .
Ethics declarations
Competing interest.
The authors declare that they have no known competing financial interests or personal relationships that could have appeared to influence the work reported in this paper.
Additional information
Responsible Editor: Gustavo Gabriel Striker.
Publisher's Note
Springer Nature remains neutral with regard to jurisdictional claims in published maps and institutional affiliations.
Supplementary Information
Below is the link to the electronic supplementary material.
Supplementary file1 (DOCX 16.8 KB)
Rights and permissions.
Springer Nature or its licensor (e.g. a society or other partner) holds exclusive rights to this article under a publishing agreement with the author(s) or other rightsholder(s); author self-archiving of the accepted manuscript version of this article is solely governed by the terms of such publishing agreement and applicable law.
Reprints and permissions
About this article
Wang, X., Yang, J., Gao, Y. et al. Allocation, morphology, physiology: multiple aspects of above- and below-ground responses to water table stress, duration of drainage in alpine wetland plants ( Carex muliensis ). Plant Soil (2024). https://doi.org/10.1007/s11104-024-06701-y
Download citation
Received : 10 March 2024
Accepted : 24 April 2024
Published : 07 May 2024
DOI : https://doi.org/10.1007/s11104-024-06701-y
Share this article
Anyone you share the following link with will be able to read this content:
Sorry, a shareable link is not currently available for this article.
Provided by the Springer Nature SharedIt content-sharing initiative
- Alpine wetland
- Carex muliensis
- Functional traits
- Phenotypic plasticity
- Phenotypic integration
- Adaptation strategies
- Find a journal
- Publish with us
- Track your research
- 0 Shopping Cart
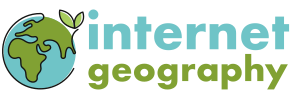
Sustainable Urban Living - East Village
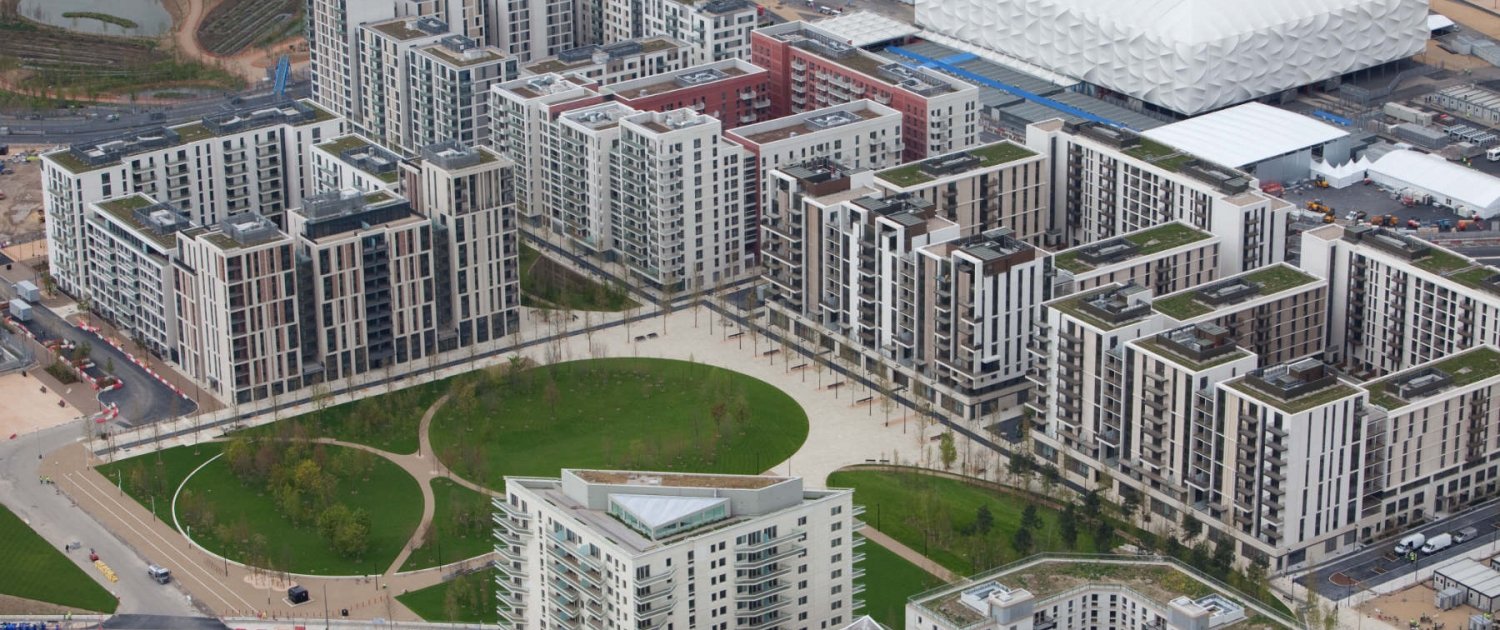
Sustainable Urban Living – East Village
East Village is one of the most sustainable communities in the UK. It is part of the London 2012 Olympic legacy and was converted from the Athletes’ Village into new homes after the Games. One of the main aims of the Olympics was to be the most sustainable Games ever.
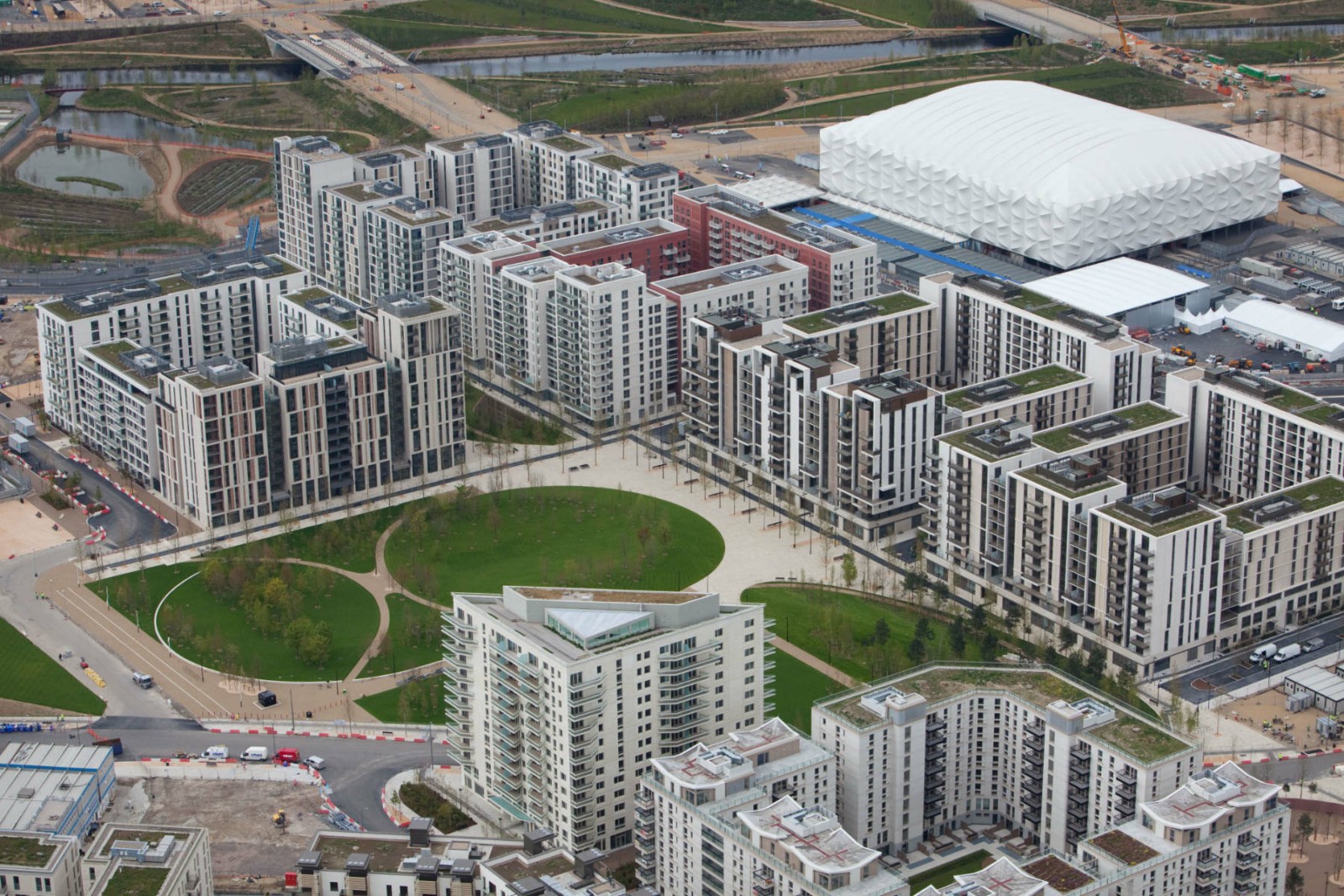
East Village, London – CC2.0 EG Focus https://www.flickr.com/photos/egfocus/7090449501/
How is East Village an example of sustainable urban living?
Transport
East Village has an integrated transport solution. Local trains and bus services connect to the London Underground. Stratford International station provides access to Central London and Europe.
Green open spaces
10 hectares of parkland have been created, containing hundreds of newly planted trees and ponds. These provide habitats for wildlife.
Modern, high-density apartments
The athletes’ village has been transformed into modern, high-density apartments. These have been constructed to a high standard of energy efficiency and insulation. Apartments lose less heat than low-rise housing.
Green roofs
A green roof or living roof is a roof of a building that is partially or completely covered with vegetation and a growing medium planted over a waterproofing membrane.
Green roofs have been included on residential blocks, providing habitats for wildlife as well as slowing the flow of surface run-off from rain.
Affordable housing
Half of the homes are available to ordinary people in East London. They are affordable as they are available to rent at lower rates.
Car parking
Parking is provided for each apartment block and residents must pay extra for a space. However, the area has good walking and cycling routes to encourage people from their cars.
Shops and services
There are 35 independent shops, cafes and bars serving the local community. This keeps money in the local area as they are less likely to travel to shop.
Public services
A large health centre has been constructed along with a school for 2000 3 to 18-year-olds. These services are essential to support the local community.
What green spaces have been created in East Village?
Although East Village is a high-density urban area, 37 per cent of the land is green open space. 10 of the 27 hectares that make up East Village is green space, including:
- a wetland area that recycles rainwater, which is used for flushing toilets and water plants;
- a large green park containing a children’s play area;
- green roofs;
- each apartment block having a shared, private green space; and
- fruit trees in an orchard in the children’s play area.
Waste Recycling
- 99% of parkland waste is recycled instead of being sent to landfill.
- Households are also encouraged to recycle with fortnightly collections of cardboard, paper, plastic bottles, tins and cans.
- Recycling is taken to a sorting facility at Jenkins Lane Reuse and Recycling Centre, where materials are separated. The sorted materials are made into bales and sent off to be recycled into new products.
How efficient is East Village?
Compared to other urban areas, East Village uses much less water and energy.
Water use in East Village is 50 per cent less than typical in urban areas. This has been achieved through recycling water naturally and using reed beds in wetland areas. Rainwater is filtered naturally before being recycled and used for irrigating plants and flushing toilets. Fresh drinking water is on a separate system.
East Village uses 30 per cent less energy than an average urban area. This is because of combined heat and power (CHP). CHP involves generating electricity and heat from the same energy source, in this case, burning biomass. Water is heated to create steam that drives a turbine to generate electricity. The hot water produced in this process is pumped around the nearby apartments and is used to heat them.
What are the benefits of East Village?
Social
- More than 10,000 new homes will have been built by 2030, and more than 10,000 new homes will have been built in the park.
- Five new neighbourhoods will be completed by the end of the decade.
- New jobs in tourism and construction have created a multiplier effect .
- By 2030 over 20,000 jobs could be created, bringing £5 billion into the area.
- The Westfield Stratford shopping centre created 10,000 permanent new jobs from day one, including 2,000 for previously unemployed local people.
Environmental
- The Olympic bid was, in part, successfully based on sustainability . The park is sustainable in many ways, including public transport , walking and cycling routes, the water and energy-efficient design of homes and the protection of green spaces and natural habitats .
- 300,000 new plants have been planted in the wetlands.
- The London Olympic Athletes’ Village is the largest sustainable homes project in the UK.
- Before construction, the Olympic Delivery Authority (ODA, 2015) excavated and cleaned more than 2.3 million cubic metres of contaminated soil.
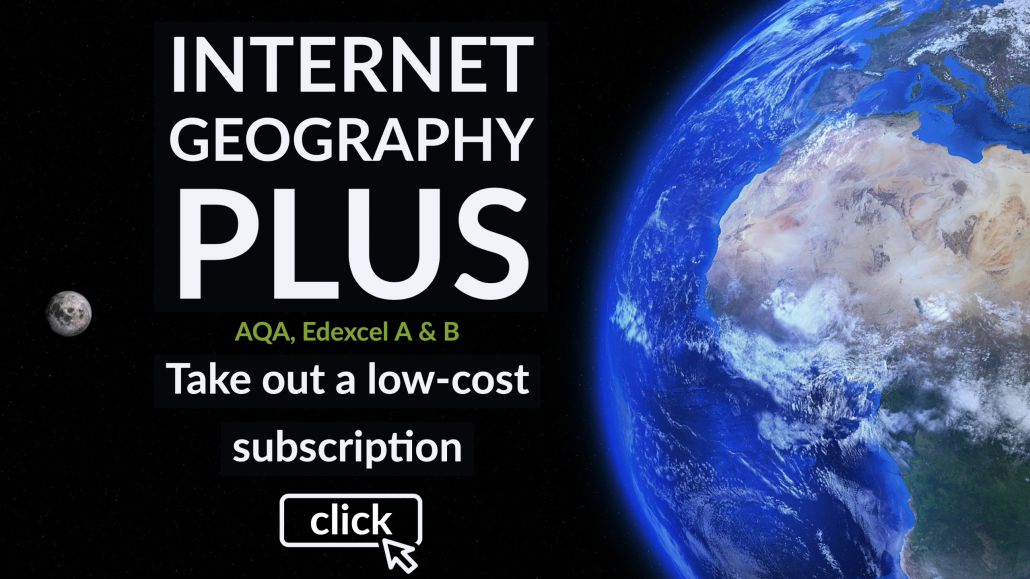
Premium Resources
Please support internet geography.
If you've found the resources on this page useful please consider making a secure donation via PayPal to support the development of the site. The site is self-funded and your support is really appreciated.
Urban regeneration in London: Lower Lea Valley
Topic home, sustainable urban living: freiburg, share this:.
- Click to share on Twitter (Opens in new window)
- Click to share on Facebook (Opens in new window)
- Click to share on Pinterest (Opens in new window)
- Click to email a link to a friend (Opens in new window)
- Click to share on WhatsApp (Opens in new window)
- Click to print (Opens in new window)
If you've found the resources on this site useful please consider making a secure donation via PayPal to support the development of the site. The site is self-funded and your support is really appreciated.
Search Internet Geography
Top posts and pages.

Latest Blog Entries

Pin It on Pinterest
- Click to share
- Print Friendly
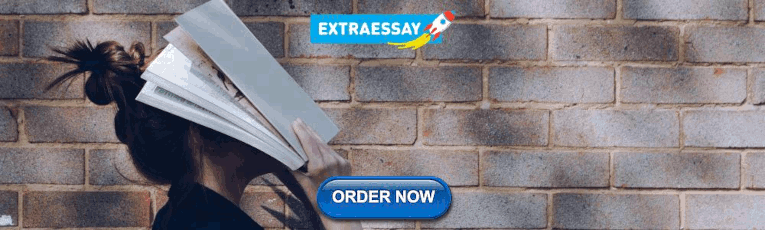
IMAGES
VIDEO
COMMENTS
Wetlands International and partners are implementing a five-year project from 2024, to restore degraded peatlands in Mongolia, which is based on the national 'Mongolian Strategic Plan for Peatlands Conservation and Wise Use'. The project will focus on four areas - Gatchuurt, Khurkh, Khashaat, and Orkhon - and will restore close to ...
NASA's Landsat program has been taking images of Earth's surface for over 25 years, and scientists have developed ways to monitor the long-term health of wetlands by examining them in these images. To see the range of studies that scientists are doing using satellite imagery, enter the terms "wetlands" and "Landsat" into an Internet search ...
Summary of basin characteristics. The Everglades is a rain-fed, flooded grass- land/wetland that once extended from Lake Okeechobee in the north to Florida Bay in the south. The slow-moving, shallow water flowed as a vast sheet through varied landscapes from sawgrass marshes to mangrove estuaries, ending its journey by mixing with the seawater ...
With wetlands categorized as one of the three major ecosystems, the study of wetland health has global environmental implications. Multiple regression models were employed to establish relationships between Landsat-8 images, vegetation indices and field measured biomass in the Yellow River Delta Nature Reserve. These models were then used to estimate the spatial distribution of wetland ...
Wetland Biogeochemistry Case Study J. Plach Oct 31 Online Quiz Wetland Ecology and Adaptation (Ch. 3, p. 274-287) Nov 5 Wetland Ecology Case Study: C. Robichaud; Robichaud and Rooney (2017) Nov 7 Human Use of Wetlands (Ch. 12, p.259-261; Ch. 13, p.262-279) Nov 12 Contamination of wetlands Case Study Nov 14 Wetland Restoration (Ch. 12, p.287-294 ...
Due to the complexity of wetland ecosystems, wetlands have a wide area of alternating land and water zones and complex vegetation composition, making it challenging to achieve dynamic displays of virtual wetland scenes using three-dimensional modeling. This study proposes a workflow of game engine-based virtual wetland scene construction for the rapid modeling of virtual wetland scenes. The ...
A wetland is an area of land that is either covered by water or saturated with water. ... Biology, Ecology, Earth Science, Geography, Physical Geography ... Case Study: Tres Rios The arid urban area of Phoenix, Arizona, serves an example of how wetlands support the economy, ...
Conversely, the localized specific problems have yielded specialized policy responses in each case. Declaration of fixed biological reference points for wetlands were not used at any stage of the policy development process. However, the wetland managers formally or informally set up ecosystem-services oriented benchmarks for urban wetland ...
Physical Geography 38: 62-82. Crossref. Google Scholar. Marambanyika T, Beckedahl H, Ngetar NS (2017b) Community strategies to promote sustainable wetland-based food security in rural areas of Zimbabwe. ... (2020) Socio-Economic Determinants of Land Use/Cover Change in Wetlands in East Africa: A Case Study Analysis of the Anyiko Wetland ...
Remote Sensing of Wetlands: Case Studies Comparing Practical Techniques. V. Klemas. Published 21 March 2011. Environmental Science. Abstract To plan for wetland protection and sensible coastal development, scientists and managers need to monitor the changes in coastal wetlands as the sea level continues to rise and the coastal population keeps ...
You will be developing a case study of a specific wetland but do remember that the emphasis is on the effectiveness of management strategies. You can also link this to competing demands for water as well as how agriculture can impact water sources. ... IB Geography Freshwater wetland management from geographypods. kissimmee_case_study 1.doc.
School of Geography, Archaeology and Environmental Studies WETLANDS MANAGEMENT AND CONSERVATION IN RWANDA. CASE STUDY: GIKONDO WETLAND Scholastica Shumbusho Mbabazi 0718806k A research report submitted to the School of Geography, Archaeology and Environmental Studies, University of the Witwatersrand, Johannesburg, in partial
The Convention uses a broad definition of wetlands. It includes all lakes and rivers, underground aquifers, swamps and marshes, wet grasslands, peatlands, oases, estuaries, deltas and tidal flats, mangroves and other coastal areas, coral reefs, and all human-made sites such as fish ponds, rice paddies, reservoirs and salt pans.
Wetland classification is the primary tool to identify and determine wetland landscapes, but it seems to be very difficult as wetlands are the transitional zone between aquatic boundary and terrestrial boundary. Wetlands are also found in different hydroclimatic and topographic settings. Recently ecological wetland classification focuses on hydrogeomorphic unit to know the physical background ...
Request PDF | The Geography of Trading Ecosystem Services: A Case Study of Wetland and Stream Compensatory Mitigation Markets | With the exception of greenhouse gas trading programs, environmental ...
1 Department of Geography and Social Studies, Kyambogo University, Kyambogo University, Kampala, Uganda. ... In this case study, Adollo wetland in Otuke district was identified as a model site comparable in structure and function to the proposed restoration sites before degradation. The reference site was used as model for catchment/ecosystem ...
This study investigates the intricate and enduring interplay of historical events, human activities, and natural processes shaping the landscape of North European Plain in western Poland over 230 ...
THE GEOGRAPHY OF TRADING ECOSYSTEM SERVICES: A CASE STUDY OF WETLAND AND STREAM COMPENSATORY MITIGATION MARKETS Philip Womble* & Martin Doyle** With the exception of greenhouse gas trading programs, environmental markets are prisoners of their own geography — and with good reason. Climate change is a
The Ruoergai alpine wetland, situated on the eastern periphery of the Tibetan Plateau, stands as the world's largest alpine wetland, covering an expansive area of 4.6 × 10 3 km 2 and hosting a substantial organic carbon reservoir, accounting for approximately 1‰ of the global total (Chen et al. 2014; Cui et al. 2015).Analogous to the prevailing predicament confronting numerous ecosystems ...
AROUND THE WORLD WITH IB DP GEOGRAPHY. ib dp geography map link to google maps. Click on the map below that shows you the spread of current case studies for the IB DP Geography course. Part 1 - Themes. Theme A - Freshwater (blue tap) Theme D - Geophysical Hazards (blue earthquake trace) Theme E - Leisure, Sport, Tourism (brown binoculars)
5.4C - Drought and Ecosystems. The impacts of drought on ecosystem functioning (wetlands, forest stress) and the resilience of these ecosystems. Ecological resilience is the capacity of an ecosystem to withstand and recover from a natural event (e.g. drought or flooding) or some form of human disturbance.
The wetlands retain 60% all year round. Impacts of 2014/2015 drought • The drought tested the resilience of the ecosystem. • There was an increase of tree mortality, which reduced habitats. • There have also been more wildfires, due to routine grass burning by cattle farmers
A case study of a sparsely populated area - Himalayan Mountains; ... 300,000 new plants have been planted in the wetlands. ... Geography Case Studies. The Holderness Coast Case Study. Typhoon Haiyan Case Study. AQA Pre-release 2024 Figure 1 Flashcards. Latest Blog Entries.
IB geography Case Study of Bhoj Wetlands, Wetland management. IB geography Case Study of Bhoj Wetlands, Wetland management. 100% satisfaction guarantee Immediately available after payment Both online and in PDF No strings attached. Previously searched by you. Previously searched by you. Sell. Where do you study. Your language.